Carl W. Dieffenbach, Edmund C. Tramont Keywords adaptive immunity; antimicrobial proteins; C-type lectin (CLR)-like receptor family; innate immunity; natural antibodies; NOD-like receptor (NLR) family; nonspecific antimicrobial barriers; pattern recognition receptors; retinoic acid–inducible gene I (RIG-I)-like receptor; Toll-like receptor (TLR) family
Innate (General or Nonspecific) Host Defense Mechanisms
Host defense mechanisms against microbial invasion are a continuum. An invading microbe first encounters the physical barrier—the skin, mucous membranes, the normal microbiome, and nonspecific antimicrobial peptides or proteins. If microbes overcome these barriers and progress toward an infection, the next hurdles are the pattern recognition receptors (PRRs) on sentinel cells (usually macrophages). The PRRs bind specific structural signatures common to bacteria, viruses, and fungi and trigger immune mediators, such as cytokines and chemokines. This initial engagement between the host and pathogen is termed the innate immune system. It is believed to constitute an evolutionarily older defense mechanism that is the dominant immune system in invertebrate organisms.1 The innate immune system provides immediate defense against an infection and must be breached for an infection to occur (Table 4-1).1 Even if breached, it also has an essential role in focusing and directing the microbial-specific long-lasting immune memory of the adaptive immune system (see Chapters 2 and 5 through 9).
The general nonspecific and innate host defense mechanisms represent an effective, broad-based surveillance program that serves as a transitional defense system, holding a foreign invader at bay while setting the stage for the host to develop specific adaptive immunity. These mechanisms provide crucial initial and continuing encounters against all microorganisms. The broad nature of the nonspecific and innate host defense mechanisms includes the general nonspecific effects resulting from physical barriers (e.g., intact skin, mucous membranes, microbicidal peptides, mucus, biofilm, cilia, peristalsis, resident microbiota, lysozyme, complement). The focus of this chapter is on the earliest events in the host-pathogen interaction process; the general, nonspecific interactions that mitigate invasion; and the processes for the detection and possible clearance of an invading organism by the host. If infection is established, how do the initial interactions create an environment for the adaptive immune system to respond?
The study of innate immunity is a rapidly evolving field, and over the past decade two areas of research have significantly changed the perception of host-pathogen interactions. First, we have learned that what was viewed as physical, nonspecific barriers involve interactions with the natural microbiota, the host commensal microbes that act in concert with the host to maintain a state of wellness.2 The microbiome, the myriad of organisms, and interactions with the human host are being unraveled. The details of these interactions are delineated in Chapters 1 and 2. Second, significant progress has been made defining the details on the genetics of susceptibility to disease.3 The susceptibility, morbidity, and mortality related to almost every pathogen and to infection in general are influenced significantly by the host’s genetic makeup. This was evident years ago when it was shown that if a child’s parent died of an infectious disease, such as pneumonia, the child has increased probability of dying of an infection (Fig. 4-1).4 Genetic associations between increased susceptibility to diseases have been mapped to sequence polymorphisms and frank mutations in many aspects of the nonspecific, innate and adaptive immune pathways. These genetic changes have been identified in Toll receptor genes,5,6–9 complement,10,11 cytokine and chemokines or their receptors,12–15 degrees of limited human leukocyte antigen (HLA) diversity,16,17 and cellular receptor specificities.18,19 Through excellent epidemiology and whole-genome sequencing, combined with the dissection of immunity by technologies such as germ line mutagenesis, the knowledge about disease susceptibility will expand and alter our perceptions.20
Natural Physical Barriers to the Entry of Microorganisms Into the Body
The morphologic integrity of body surfaces is an important and effective first line of defense. The epithelial coverings and linings share many similar properties, such as production of defensins,21,22 cathelicidins,23 and populations of γδ cells24,25 and unique defense molecules such as lysozyme.26,27 Table 4-228–30 lists the major human host defense proteins that have been characterized. Although we have these nonspecific barriers, we have also come to appreciate the vast nature of the microbe communities that colonize each of us. On a per cell basis, we are more bacterial than human.2 The interactions between the microbiome and host defense proteins, which give the appearance of a seemingly symbiotic relationship, is most evident when evaluating the interactions in gut mucosa.
TABLE 4-2
Secreted Antimicrobial Peptides (ADPs) and Proteins
ADP/PROTEIN | CELLULAR SOURCE | SPECIAL CHARACTERISTICS |
α-Defensins | ||
HNP1-HNP4 | Neutrophils, eosinophils | Broad spectrum of antimicrobial activity; chemoattracts monocytes, T cells, dendritic cells; inhibition of complement activation; activation of mast cells |
HD-5–HD-6 | Intestinal tract, HD-5 (female reproductive tract) | Released as propeptide by Paneth cells |
β-Defensins | ||
hBD-1 | Keratinocytes, airway epithelia, urogenital tract | Antimicrobial activity compared with other β-defensins |
hBD-2 | Keratinocytes, airway epithelia, intestinal tract | Mainly active against gram-negative bacteria and fungi; chemoattracts dendritic cells, T cells, neutrophils, mast cells |
hBD-3 | Keratinocytes, airway epithelia | Potent broad spectrum of antimicrobial activity |
hBD-4 | Airway epithelia, keratinocytes (mRNA) | Potent activity against Pseudomonas aeruginosa, less activity against Escherichia coli and gram-positive bacteria |
hBD-6 (propeptide) | Epididymis, testis, airway epithelia | E. coli |
hBD-18 | Epididymis, testis, sperm, pancreas | E. coli |
hBD-28 | Epididymis, testis | Antimicrobial activity |
Cathelicidin LL-37 | Leukocytes, airway epithelia, urogenital tract, keratinocytes | Antimicrobial activity, immunomodulatory functions |
Ribonucleases | ||
Eosinophil cationic protein (ECP) | Eosinophils | Antibacterial and antiviral activity |
Eosinophil-derived neurotoxin (EDN) | Eosinophils | Antiviral activity, chemoattracts and activates dendritic cells |
Angiogenin (RNase 5) | Leukocytes, epithelial cells, fibroblasts | Antimicrobial activity and angiogenic activity |
RNase 7 | Keratinocytes, airway epithelia | Antimicrobial activity |
RNase 8 | Placenta | Antimicrobial activity |
S100 proteins | ||
Psoriasin (S100A7) | Keratinocytes, airway epithelia, urogenital tract | Potent antibacterial activity against E. coli |
Calprotein (S100A8/A9) | Leukocytes, skin | Active at high concentrations against Candida albicans |
Calcitermin (S100A12) | Airway secretions | Active against gram-negative bacteria |
Complement | Hepatocytes (blood) | Microbial lysis, chemotaxis, immunomodulatory functions, immune clearance |
Mannose-binding lectin/protein (MBL/MBP) | Liver | Binds to carbohydrates on the surface of viruses, bacteria, fungi, protozoa to activate the complement system or act directly as an opsonin |
Fibronectin | Hepatocytes (blood) | Blocks the attachment of many microorganisms, especially P. aeruginosa |
Histatins | Salivary gland exocrine cells | Antifungal activity |
Lactoferrin/lactoferricin | Neutrophils, body fluids | Inhibits bacterial growth by its ability to sequester iron; toxic to several viruses |
Bactericidal/permeability-increasing protein (BPI) | Neutrophils, epithelial cells | Active against gram-negative bacteria, neutralizes LPS |
Dermcidin (DCD-1) | Sweat glands | Anionic peptide, exclusively produced by human eccrine sweat glands |
Antileukoprotease (ALP) | Keratinocytes, airway epithelia, gut, body fluids | Broad-spectrum antimicrobial activity; inhibits various proteinases (e.g., elastase, cathepsin G, trypsin); contributes to anti-HIV effect of saliva |
Cryopyrin | Macrophages, neutrophils | Antimicrobial activity |
Lysozyme | Skin, airway epithelia, body fluids, tears | Broad antibacterial activity by degrading peptidoglycans |
Elafin | Neutrophils, epithelial cells | Weak antimicrobial activity |
Phospholipase A2 (PLA2) | Neutrophils, macrophages, Paneth cells, tears | Antimicrobial activity, especially against gram-positive bacteria |
Peptidoglycan recognition proteins | Neutrophils, various epithelial cells | Antimicrobial activity, especially against gram-positive bacteria |
Neutrophil gelatinase-associated lipocalin (NGAL) | Neutrophils, various epithelial cells | Antimicrobial activity through its ability to bind bacterial ferric siderophores |
Hepcidin | Liver | Mainly acts as an iron-regulatory hormone (limits iron availability to invading microorganisms) |
Surfactant proteins | Mucosal surfaces | Antimicrobial activity |
Granulysin | Cytolytic T cells, natural killer cells | Antimicrobial activity |
Histones | Neutrophils, gut, placenta | Bactericidal activity, LPS-neutralizing activity |
Adrenomedullin | Epithelial cells, sweat and sebaceous glands, body fluids | Antimicrobial activity |
Azurocidin (CAP37) | Neutrophils | Mainly against gram-negative bacteria, increases vascular permeability, binds to endotoxin, attracts monocytes |
Cathepsin G | Neutrophils | Proteolytic-independent antibacterial activity |
Proteinase 3 | Neutrophils | Active against bacteria and fungi |
Platelet-derived AMPs | Platelets | Seven AMPs have been isolated from platelets |
Chemokines | Many cell types | Many chemokines have antimicrobial activity |
Human epididymis 2 (HE2) family | Epididymal | Family of sperm-binding proteins with antimicrobial activity |
Dermcidin | Skin eccrine sweat glands | Antimicrobial activities, keratinocyte activation |
Tamm-Horsfall protein | Kidney | Binds microbes |
AMP, adenosine monophosphate; HIV, human immunodeficiency virus; LPS, lipopolysaccharide.
Data from Harder J, Glaser R, Schroder JM. Human antimicrobial proteins—effectors of innate immunity. J Endotox Res. 2007;13:317-338; Ganz T. Defensins: antimicrobial peptides of innate immunity. Nat Rev Immunol. 2003;3:710-720; Chen H, Zhiman X, Peng L, et al. Recent advances in the research and development of human defensins. Peptide. 2006;27:931-940; and Pazigier M, Hoover DM, Yang D, et al. Human β-defensins. Cell Mol Life Sci. 2006;63:1294-1313.
Skin
The intact skin forms an effective mechanical barrier to invasion by microorganisms partly because it is composed of tightly associated epithelial cells covered by a highly cross-linked keratin layer. As noted, the skin possesses an array of antimicrobial properties that form a protective shield consisting of a battery of broad-spectrum defensive chemicals, principally peptides, synthesized as precursors and processed by specific proteases into mature active forms that target microbial membranes in a manner similar to disinfectants. These help keep pathogenic organisms out, and the microbiota are also checked by these functions. The antimicrobial peptides include cathelicidins, β-defensins, and dermicidin.22,23,29,31–34 Not only do they function directly to kill microbes, they also act as chemokines to attract migrating phagocytic cells. β-Defensin, LL-37 (an antimicrobial peptide), cathelicidin levels, and hexadecenoic acid are depressed in atopic skin, a condition in which microbial suprainfection is common.35,36
The relative dryness and mild acidity (the so-called acid mantle, pH 5 to 6) of skin, combined with normal skin microbiota act to form an effective prohibitive environment. Inflamed skin is more permeable to water and is more hospitable to colonization. It has been speculated that oily skin may retard evaporation of water, resulting in increased numbers of colonizing microorganisms. The acidity of the skin results from the breakdown of lipids into fatty acids by skin microbiota. Sebum triglycerides are partially hydrolyzed, liberating fatty acids. The continual desquamation of skin scales also aids in the elimination of microorganisms. Because few organisms have the ability to penetrate the skin, they usually gain access by some physical means, such as an arthropod vector, trauma, surgical incision, or intravenously placed catheter.
Mucous Membranes
Because of the inherent moisture with which they are associated, mucous membranes support a broader spectrum and larger number of microorganisms than does skin. Most epithelial cells possess the same peptide shield as skin (see Table 4-2)29,37,38; however, body secretions, including saliva, cervical mucus, prostatic fluid, and tears, are endowed with unique antimicrobial properties. Two of the more potent antimicrobial substances are lysozyme and N-acetylmuramyl-l-alanine amidase (NAMLAA). Both substances are particularly effective against gram-positive bacteria because they hydrolyze the amino acid backbone of peptidoglycan.39
Local secretions also contain immunoglobulins, principally IgG and secretory IgA, which act primarily to agglutinate microorganisms, block the attachment of organisms to receptors on host cells competitively, or both. The body produces more secretory IgA than total IgG. The IgA immunoglobulin is made relatively resistant to proteolysis by binding with a unique polypeptide known as the secretory component and protein Fv.40 There is also evidence that mucosal IgA can bind intracellular pathogens or products as they are transported through the cell.41
Mucosal secretions also contain significant amounts of iron-binding proteins. Iron is a critical element for most microorganisms, and fluids that potentially are exposed to microbes are enriched with iron-binding proteins that act to keep this important factor from the microorganisms. In contrast, microorganisms that routinely colonize skin and mucosal surfaces have evolved mechanisms to acquire iron despite these proteins.42,43
Respiratory Tract
The respiratory tract has a formidable array of antimicrobial defense mechanisms. The inhaled particles must survive and penetrate the aerodynamic filtration system of the upper airway and tracheobronchial tree. The airflow in these areas is turbulent, causing large particles to come in contact with the mucosal surfaces and face the full array of those defense mechanisms. Humidification also causes hygroscopic organisms to increase in size, aiding trapping.
When a particle is deposited, the mucociliary blanket transports the invading offender away from the lung. Coughing aids this expulsion. This system is amazingly efficient; 90% of deposited material is cleared in less than 1 hour.44 In addition, the bronchial secretions contain various antimicrobial substances, such as lysozyme, NAMLAA, and β-defensins such as hBD-1 and hBD-2,45 RNase 7 and pulmonary lectins,46 and surfactant collectins.38,46,47 The airway epithelium also contributes to the resistance to infection. On exposure to pathogens, lung epithelial cells express S100A, antileukoprotease,29 and large amounts of major vault protein (MVP). In cells infected with Pseudomonas aeruginosa, MVP I localizes in lipid raft sections of cell membranes and helps mediate bacterial clearance.48
After a particle reaches the alveoli, physical expulsion becomes much less effective and the alveolar macrophages and tissue histiocytes play a more prominent role in protecting the host. These phagocytic cells are assisted in their defense by the collectin surfactants SP-A and SP-D, which bind to and opsonize diverse organisms, including gram-negative bacteria, fungi, and Pneumocystis jirovecii (formerly known as Pneumocystis carinii).49
Like all defense mechanisms, these nonspecific mechanisms can be overcome by the introduction of large numbers of invading organisms (e.g., a contaminated respirator), particularly if the host is exposed for an extended period. Their effectiveness is decreased by air pollutants (e.g., cigarette smoke), mechanical respirators, tracheostomy, concomitant infection, allergenic agents, and, in some cases, genetic defects (e.g., cystic fibrosis) and inhibitory factors of some pathogens.47,50
Intestinal Tract
The acid pH of the stomach and the antibacterial effect of the various pancreatic enzymes, bile, and intestinal secretions are effective, nonspecific, antimicrobial defense factors. Paneth cells of the small intestine, located in the crypts of Lieberkühn, secrete antimicrobial substances, such as β-defensins, lysozyme, and type II phospholipase A.2,51 As with other mucosal surfaces, the intestinal tract expresses microbial pattern recognition receptors on a number of cell types.52 Understanding how these components work to exclude pathogens while maintaining commensal species is important for maintaining the health of the gut. For example, the development of natural immunity, the cross-reacting antibodies against blood group antigens, and potential pathogens such as Neisseria meningitidis is dependent on this process. The microbiome has been shown to keep the intestinal environment in a constant state of “appropriate” immune activation, thus triggering antimicrobial proteins, tissue repair factors, and immunoglobulin A, resulting in the maintenance of the intestinal barrier and gut immunity.2,51,53 Microbial colonization in germ-free mice triggers the expression of a secreted C-type lectin with known antibacterial properties. The protein in humans, HIP/PAP (hepatocarcinoma-intestine-pancreas/pancreatic-associated protein),54 represents a form of innate response to help maintain a symbiotic relationship.55 Peristalsis and the normal loss of epithelial cells also act to purge the intestinal tract of harmful microorganisms. Alteration of these parameters can lead to increased susceptibility of the host to infection. For example, Salmonella and Mycobacterium tuberculosis infections are more common in achlorhydric patients and slowing peristalsis with belladonna or opium alkaloids prolongs symptomatic disease caused by gastrointestinal pathogens such as Shigella species. A number of disease states have been shown to be accompanied by the movement, or translocation, of gram-negative bacterial products across the epithelial barrier and into the circulation.56 This is often accompanied by a loss of tight junctions between enterocytes or the loss of enterocytes altogether. This may be tied to loss or impairment of a subset of T cells, Th17 cells, which secrete the enterocyte growth factor cytokine Th17.57
The importance of a symbiotic intestinal microbiome has long been appreciated and has been highlighted by the success of fecal transplants in patients with intractable Clostridium difficile infections.58 The normal bowel microbiota compete for nutrients (1012 organisms/g of feces), and this plays a crucial protective role. Altering this symbiotic normal intestinal microbiota, such as with broad-spectrum antibiotics, can lead to overgrowth of inherently pathogenic organisms (e.g., Salmonella typhimurium) or suprainfection with ordinarily commensal organisms (e.g., Candida albicans). As always, the interfering competitive capacity of the normal microbiota can be overcome by the introduction of large numbers of pathogenic organisms. The probability of development of salmonellosis is related directly to the number of Salmonella organisms ingested. One exception to this is Shigella, which requires very few organisms to establish infection.
Genitourinary Tract
Urine has been considered sterile. Evidence is emerging that there is a spectrum of uncultivated bacteria present in the bladders of adult females.59 The contribution of these organisms to keeping the bladder pathogen free remains to be determined. In this regard, urine is bactericidal for some strains of bacteria, mostly because of its pH, although factors such as hypertonicity, urea, and other solutes play a role. Tamm-Horsfall protein is a glycoprotein produced by the kidneys and excreted in large amounts in urine (approximately 50 mg/L). Because many bacteria are avidly bound to it, the protein acts as a sponge and is a natural host defense mechanism against tissue colonization and subsequent infection by preventing these bacteria from gaining a foothold on the cellular lining of the urinary tract.60
The lower urinary tract is rinsed with urine four to eight times each day, eliminating potential pathogenic organisms, unless they are capable of firmly attaching to epithelial cells of the urinary tract (e.g., Neisseria gonorrhoeae, certain strains of E. coli). Urinary retention or lack of complete bladder emptying impedes this flushing process.
The length of the male urethra (20 cm in an adult) also provides passive protection, and bacteria seldom gain access to the bladder in men unless introduced by instrumentation. The female urethra is much shorter (5 cm in an adult) and is traversed more readily by microorganisms, which is one reason why urinary tract infections are 14 times more common in women than in men.
The hypotonic state of the kidney medulla presents an unfavorable milieu for most microorganisms. This can be decreased by increased glucose urine levels secondary to hyperglycemia. This is a factor that accounts for the increased incidence of pyelonephritis in diabetic patients.
The vagina has an additional unique mechanism of protection. Under hormonal influence, especially estrogen, the vaginal epithelium contains increased amounts of glycogen that Döderlein’s bacilli and other commensals metabolize into lactic acid. Döderlein’s bacilli is an all-encompassing term used to describe acidogenic gram-positive rods, especially lactobacilli, residing in the vagina. Normal vaginal secretions contain 108/mL of these bacteria. They establish an acid environment that is unfavorable to most pathogenic microorganisms. The vaginal secretions of women with nonspecific vaginitis or vaginosis, which is a perturbation of the normally protective vaginal ecosystem, and of patients with other sexually transmitted diseases are characterized by an elevated pH.61
Eye
Constant bathing of the eye by tears is an effective means of protection. Foreign substances continually are diluted and washed away via the tear ducts into the nasal cavity. Tears also contain large amounts of lysozyme, lactoferrin, and lipocalin.62
Innate Immunity and the Inflammatory Response
When the normal barriers are breached and infectious agents enter tissues, a range of host factors and cell types become mobilized. Although many of these initial response proteins (see Table 4-2) are always present, it is their rapid quantitative increase that constitutes the inflammatory reaction. Clinically, this results in fever and malaise that typically accompany the early phases of infection and represent, in broad terms, the events that occur during infection before the establishment or boosting of the specific adaptive immune response. Traditionally, the factors that have correlated with the inflammatory response include (1) trigger molecules, which constitute signal systems that invoke the adaptive immune system, and (2) effector molecules, which are involved in inflammation, antimicrobial scavenging, and killing (see Table 4-2).
Insights into how the host senses an invader and which microbial components the host detects have come from many unexpected directions. Through many elegant experiments, investigators have shown that a set of genes known as the Toll gene family is involved in embryonic development and antifungal responses in fruit flies. The importance of this discovery was appreciated fully with the identification of the lipopolysaccharide receptor as a Toll-like receptor (TLR) gene.63 This discovery provided the direct evidence that the TLR genes are responsible for sensing and triggering the initial response. The Toll gene family is conserved in all species from plants to humans and performs similar functions for all multicellular creatures.64 This detection and triggering of responses to fungal, bacterial, and viral products has been preserved across extensive evolution and is referred to as the innate immune system. There are a number of cellular receptor families that recognize pathogen-associated molecular patterns (PAMPs), and the detection of and response to these PAMPs are the essential basis of innate immunity. In addition to the TLR family, these include 64,65 the nucleotide-binding oligomerization domain (NOD)-like receptor (NLR) family,66,67 C-type lectin-like receptor (CLR) family,68,69 and retinoic acid–inducible gene I (RIG-I)–like receptor.70
Chemokines and Chemotaxis
Critical to the innate response is the ability of leukocytes to move to the site of pathogen invasion. Two main families of chemokines, part of the first set of response factors induced by the innate immune system, direct such leukocyte migration.71,72 Members of the CXC family, whose first two cysteines are separated by a single amino acid, stimulate chemotaxis of polymorphonuclear neutrophils (PMNs), eosinophils, monocytes, dendritic cells, natural killer (NK) cells, and T lymphocytes. Members of the CC family, whose first two cysteines adjoin each other, stimulate all these leukocytes except PMNs, which do not express receptors for CC chemokines. More than 45 chemokines, 11 receptors for CC chemokines, and 6 receptors for CXC chemokines have been described. The defect in the chemokine receptor CCR5 is best known for providing resistance to HIV infection. However, loss of this chemokine receptor has also been shown to be associated with increased susceptibility to symptomatic West Nile virus infection.73 This effect is related in some way to the loss of known chemokine effects on upregulation of adhesion molecules, stimulation of leukocyte migration, and lowering the threshold for T-cell activation. Cytokines, especially tumor necrosis factor-α and interleukin (IL)-1, also increase expression of adhesion molecules on endothelial cells, PMNs, NK cells, and monocytes, which aid in the binding and transmigration of these cells into sites of inflammation.
The interactions of chemokines on target cell populations are extraordinarily complex. Resting T cells express few chemokine receptors, but these are strongly upregulated by IL-2 in conjunction with stimulation via the antigen receptor. The most vigorous immigration of T cells to inflammatory sites occurs in conjunction with acquired immunity rather than during the innate response.
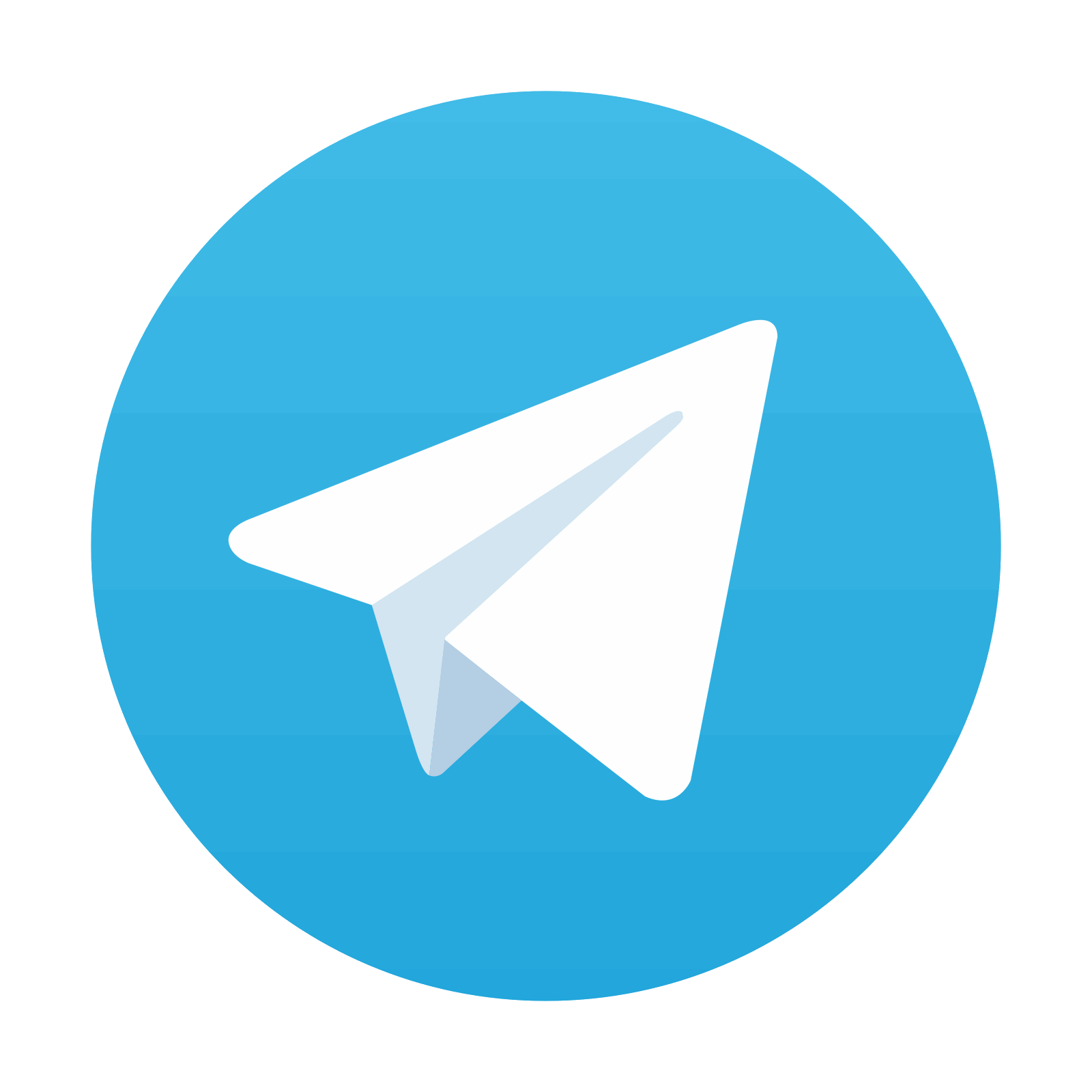
Stay updated, free articles. Join our Telegram channel

Full access? Get Clinical Tree
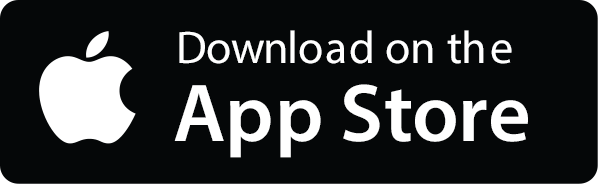
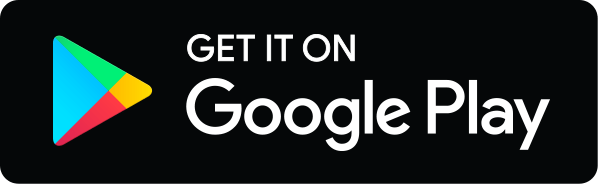