© Springer International Publishing Switzerland 2017
Eliza B. Geer (ed.)The Hypothalamic-Pituitary-Adrenal Axis in Health and Disease10.1007/978-3-319-45950-9_3Glucocorticoids: Inflammation and Immunity
(1)
Signal Transduction Laboratory, National Institute of Environmental Health Sciences, Department of Health and Human Services, National Institutes of Health, 111 T. W. Alexander Dr., Research Triangle Park, NC, USA
Abstract
Glucocorticoids are universally prescribed as the drug of choice for the treatment of inflammatory and autoimmune disorders. These stress hormones act through their cognate glucocorticoid receptor to regulate transcription of various target genes. The mechanisms of glucocorticoid action are often cell type dependent and involve the regulation of thousands of genes. Glucocorticoids have a tremendous impact on the immune system during inflammation including effects on the plasticity, survival, and function of immune cells. This chapter highlights the dynamic effects of glucocorticoids in regards to both physiological and pathological conditions during inflammation. We address issues involving classical and alternative mechanisms of glucocorticoid inhibition, the effect on innate and adaptive immunity, glucocorticoid tissue-specific actions, and their role in target immune cells.
Keywords
GlucocorticoidSteroidsStress hormonesInflammationGene expressionTransrepressionTransactivationInnate immunityAdaptive immunityResistanceIntroduction
Glucocorticoids are primary stress hormones that function throughout the body to regulate a diverse array of physiological systems. Glucocorticoids (GCs) derived their name from early observations of their effect in regulating glucose metabolism [1, 2]. Currently, the actions of this class of steroids extend beyond the mobilization of amino acids and gluconeogenesis and are known to play important roles in the control/regulation of various biological processes . In fact, glucocorticoids are required for life, as the absence of these stress hormones results in death prior to or at the time of birth. Glucocorticoids influence a number of physiological systems including the immune system [3] where in addition to exerting both pro- and anti-inflammatory actions, this stress hormone has a potent role in development and homeostasis of T lymphocytes [4]. Additionally, these stress hormones impact development [5], where glucocorticoids are known to play a key role in the maturation of the fetal lung [6]. Furthermore, glucocorticoids have a role in the brain where they have been shown to regulate arousal and cognitive functions controlled by the hippocampus, amygdala, and the frontal lobes of the brain [7]. The pleiotropic actions of glucocorticoids occur through binding to its cognate receptor, the glucocorticoid receptor (GR), which is expressed in nearly every cell in the body. Glucocorticoids act through its receptor to regulate transcription of various target genes , however as will be discussed later, nongenomic effects have also been described.
Human GR protein is encoded by the NR3C1 gene located in chromosome 5 (5q31) and is a member of the nuclear receptor superfamily of ligand-dependent transcription factors [8]. Like other steroid receptors, GR is modular in structure containing an N-terminal regulatory domain (NTD), a central DNA-binding domain (DBD), a hinge region, and a C-terminal ligand-binding domain (LBD) [9]. In the absence of hormone, GR resides in the cytoplasm in a complex with other proteins including heat shock protein 90, heat shock protein 70, and FKBP52, the latter being an immunophilin molecule involved in protein folding and trafficking. Upon ligand binding, GR is released from its cytoplasmic complex and translocates into the nucleus where it interacts with specific targeting sequences termed glucocorticoid-response elements (GREs ) to regulate thousands of genes. The nature of the GR-occupied GRE results in either induction or repression of target gene expression. Additionally, GR can undergo a conformational change upon binding to the GRE that leads to the recruitment of cofactors and/or coregulators to modulate, and thereby alter the transcriptional rates of target genes [10]. Along with the nature of the GRE and the recruitment of cofactors and/or coregulators, several other factors can also influence GRs ability to regulate gene transcription including chromatin structure, epigenetic regulators, and its physical interaction with other transcription factors such as nuclear factor kappa B (NF-kB) and activator protein 1 (AP-1).
GR, while derived from a single gene, has multiple functionally distinct isoforms due to alternative splicing and translational initiation mechanisms [11]. Alternative splicing accounts for 2 discrete receptor isoforms (GRα and GRβ) that differ at their carboxyl termini, while alternative translation initiation results in 8 additional receptor subtypes, each with a progressively shorter NTD. GRα has been the primary and most extensively studied glucocorticoid receptor, as the GRβ splice variant does not bind GCs [12]. However, expression of GRβ has been associated with glucocorticoid resistance and tissue specificity, as GRβ has been shown to antagonize the activity of GRα [13, 14]. In contrast to GRβ, the 8 unique translational isoforms of GRα have similar binding affinities for glucocorticoids and can interact with GREs. Similar to GRβ, the expression of these various translational GRα isoforms varies widely across tissues. Thus, the existence of numerous GR isoforms is thought to be a major factor contributing to the diverse array of tissue-specific actions of glucocorticoids in the body.
Glucocorticoids are also known to have potent immunosuppressive and anti-inflammatory actions , thus being vital in the treatment of autoimmune and inflammatory diseases and are one of the most widely prescribed drugs in the world. In this review, we will focus on the how glucocorticoids modulate and interact with the immune system, along with its effect on combating inflammation. Additionally, we will discuss how glucocorticoids affect the response and behavior of different immune cells in the management of inflammatory diseases.
Inflammation as a Natural Host Defense Mechanism and Glucocorticoid Regulation
Inflammation is an innate defensive mechanism that protects us from damaging stimuli such as pathogens and harmful irritants. Inflammation is a complex biological process that initially involves increased blood flow and movement of immune cells, especially granulocytes and macrophages along with other molecular mediators, from the blood to the site of injury. This acute response sets the stage for the healing process by combating the initial source of inflammation through the removal of necrotic cells and damaged tissue in a coordinated response involving both the immune and vascular systems. As the inflammation process continues, a shift in the type of cells present at the site of injury results in the repair or healing of the tissue.
Classic signs of inflammation include pain, redness, swelling, warmth, and loss of function or immobility at the site of damage. Additionally, inflammation may result in more global symptoms such as fever, chills, fatigue, and general stiffness. Pain expressly plays an important role in the ability of glucocorticoids to regulate inflammation. Cytokines and inflammatory mediators released into the blood from the damage site activate peripheral pain receptors. Pain signals sent to the brain result in the activation of the hypothalamic–pituitary–adrenal (HPA) axis which, in turn, induces glucocorticoid secretion. GCs inhibit the synthesis of cytokines and inflammatory mediators to counter the extent of the inflammation. Despite the initial unfavorable effects of this condition, inflammation is extremely important and beneficial to human health as infections and wounds, or any damage tissue, would not heal without this homeostatic response.
As with all physiological responses in the body, inflammation needs to be regulated especially in conjunction with other host defense systems . Too little inflammation can result in progressive and detrimental tissue destruction, while excessive inflammation can lead to a host of diseases including allergies, autoimmune disorders, chronic inflammatory diseases, and even cancer. Glucocorticoids regulate and reduce the inflammatory response by entering cells and suppressing the transcription of proteins that promote inflammation. In the absence of glucocorticoids, persistent inflammation can lead to dysregulation of converging pro- and anti-inflammatory factors at the site of injury resulting in abnormalities and pathogenesis [15].
Since glucocorticoids are known to be essential for the regulation of the inflammatory response, they also act to reduce the extent of an overactive immune system. Thus, glucocorticoids are among the most widely prescribed drugs for the treatment of asthma, allergies, and autoimmune diseases. This class of steroid hormones initiates a multitude of diverse signaling pathways that hold inflammation in check and counter a rampant immune system, limiting the excessive damage that can occur to the host cells and surrounding tissue [16]. A major barrier in employing glucocorticoid therapy in the clinic has been our lack of understanding of the molecular mechanisms that resolves inflammation. At one level, the mechanism of glucocorticoid action in counteracting inflammation may appear simplistic as this class of drug (GC) acting on its receptor (GR) modulates gene transcription to inhibit the extent of inflammation. However, the heterogeneity of glucocorticoid receptor isoforms and the cell-type specific biological responses suggest that GR’s ability to prevent inflammation is not a simple endeavor but a complex series of events. Thus, there are many ways glucocorticoids exert their anti-inflammatory effects.
Classical Mechanisms of Glucocorticoid Inhibition of Inflammation
Glucocorticoids utilize a variety of processes simultaneously to control inflammation; from the activation of anti-inflammatory genes, to suppressing proinflammatory cytokines and chemokines, to moderating key proinflammatory regulators such as NF-kB and AP-1. Several fundamental mechanisms have been elucidated for these actions of GR. First, direct binding of GR to GREs in DNA can enhance the transcription of anti-inflammatory genes (transactivation). Glucocorticoid-induced transactivation of genes such as IL-10, IL-1 receptor antagonist, and mitogen-activated protein kinase phosphatase-1 (MKP-1) is known to increase gene expression and thus protein expression of these anti-inflammatory molecules [17, 18]. Second, direct binding of GR to negative GREs (nGRE ) in DNA can suppress transcription (transrepression) of various proinflammatory cytokines and modulators such as iNOS, COX-2, IL-1β, and TNF [17, 18]. Finally, GR binding directly to transcription factors like the p65 subunit of NF-kB or AP-1 can prevent downstream transcription of proinflammatory mediators to control the extent of inflammation [19]. This latter mechanism of transcriptional repression, known as tethering, where GR does not directly bind DNA response sequences, has been shown to be key in GRs ability to regulate inflammation [20–22]. Additionally, cross-talk between GR and other transcription factors can occur through the binding to composite or overlapping response elements [23]. Interestingly, while the repression of NF-kB by GR has long been considered a crucial determinant in reducing the expression of specific proinflammatory targets, a recent study by Altonsy et al. showed the cooperative association between the GR and NF-kB enhanced the expression of TNFAIP3 , a potent anti-inflammatory gene and inhibitor of NF-kB, suggesting a greater complexity in the cross-talk of these two molecules [24].
GR binding directly to either GREs or nGRE s that initiate or suppress gene expression of anti- or pro-inflammatory genes, respectively, is not the only mechanism to consider in GRs ability to control inflammation. While it has been suggested that the number of GREs present play a role in the activation or suppression of gene transcription, it has become increasingly clear that their proximity to the TATA box also is an important factor [25]. Additionally, the recruitment of various coactivators such as TIF2 and SRC-1, corepressors such as NCoR and SMRT, along with various other comodulators can interact with the GR-DNA complex enabling an additional level of transcriptional regulation in GR’s ability to control inflammation [26, 27]. GR may regulate inflammation by reducing mRNA half-life through the GC responsive gene tristetraprolin (TTP) [28, 29]. GR can also be phosphorylate d by various kinases that can affect its stability, its DNA binding capacity, its ability to translocate to the nucleus, and its interactions with other transcription factors and/or molecular chaperones [30]. Thus, the simple concept of one drug (GC) working on its receptor (GR) has evolved to comprise numerous multifaceted mechanisms to control and regulate inflammation.
Furthermore, glucocorticoids have been shown to regulate inflammation in the absence of DNA binding or interactions with other transcription factors. Nongenomic GC–GR interactions were shown to account for the cardioprotective effect of an acute high dose of corticosteroids resulting in the nontranscriptional activation of endothelial nitric oxide synthase (eNOS) [31]. eNOS has been shown to play an important role during inflammation in regulating the expression of proinflammatory molecules such as NF-kB and cyclooxygenase-2 (Cox-2) [32, 33]. Additionally, nongenomic GC–GR mechanisms involving the activation/inhibition of various signaling pathways, including the p42 MAPK and MAPK ERK1/2 pathways, and the activation of proteins with SH3 domains such as Src and Ras that in turn activate the aforementioned kinase pathways, have been shown to occur [34]. Finally, a mechanism of GC anti-inflammatory action still in its infancy involves posttranscriptional gene regulatio n via RNA-binding proteins and microRNAs [35]. Interestingly, the role of these posttranscriptional gene regulation actions is thought not to function specifically in turning on or off genes, but to act more as a rheostat in controlling the appropriate amplitude and duration of the response [36].
As the modulation of gene transcription is the major consequence of glucocorticoid activity, the changes in gene transcription that occur directly via activation/repression of GC target genes, or through tethering to another transcription factor, are the most well studied means in controlling inflammation. However, GR can also modulate gene transcription indirectly through the consequences of the activation of the initial target gene. A classic example of this mode of GR regulation involves the glucocorticoid-induced leucine zipper (GlLZ) gene . GILZ encodes for a potent anti-inflammatory protein with immunosuppressive and cell survival-promoting effects. GILZ was initially identified as a molecule that protected lymphocytes from TCR/CD3-activated cell death [37]. However, subsequently it was shown that GILZ itself did not directly protect lymphocytes from death, but inhibited the ability of the T cell receptor to induce interleukin-2/interleukin-2 receptor expression and NF-kB activity [38]. Specifically, it was shown that GILZ inhibited NF-kB nuclear translocation and DNA binding via direct protein-to-protein interaction of GILZ with NF-kB. Since these initial observations, GILZ has been shown to be a multifunctional protein that can inhibit key immune cell signaling pathways. Recently, GILZ was shown to regulate Th17 responses and to restrain IL-17-mediated skin inflammation [39]. While the anti-inflammatory and immune-modulatory effects of GILZ have been widely described [40, 41], the induction of this protein has also been associated with provoking apoptosis. GILZ expression in human neutrophils promoted apoptosis through the down-regulation of the myeloid leukemia cell differentiation protein Mcl-1, an antiapoptotic protein of the Bcl-2 family [42].
Alternative Mechanisms of Glucocorticoid Inhibition of Inflammation
The ability of glucocorticoids to regulate and control inflammation goes beyond simply regulating gene transcription of pro- and anti-inflammatory genes. Recently, a unique mechanism of action for anti-inflammatory effects of GCs was reported during the early phase of acute lung injury (ALI) [43]. These authors showed that glucocorticoids attenuate inflammation associated with ALI via up-regulation of the SphK1 gene in macrophages. The up-regulation of sphingosine kinase 1 in the lung resulted in the synthesis of sphingosine 1 (S1P) that in turn binds to the S1P receptor type 1 (S1PR1) and triggers the Rho family-dependent reorganization of the cytoskeleton leading to enhanced barrier function of the endothelium. The protection afforded by glucocorticoids to enhance the barrier function through this mechanism prevents vascular leakage and the massive infiltration of immune cells into the lung as a way of controlling inflammation.
Interestingly, another recent study linked the action of glucocorticoids to the circadian clock to control time-of-day variations and magnitude of pulmonary inflammation [44]. In this study, the authors observed that pulmonary antibacterial responses of neutrophil recruitment via the chemokine and glucocorticoid responsive gene CXCL5 were modulated by a circadian clock mechanism within epithelial club (Clara) cells [45]. Intriguingly, adrenalectomy blocked this circadian neutrophil recruitment and rhythmic inflammatory responses afforded by CXCL5 upon intraperitoneal injection of LPS. Therefore, this study suggests that the therapeutic effects of glucocorticoids can depend on the local circadian circuit regulation of GR function.
Glucocorticoids have also been shown to suppress overactive inflammatory responses by induction of negative feedback regulators such as the interleukin-1 receptor-associated kinase M (IRAK-M; also known as IRAK3). IRAK-M is known to be a critical negative feedback regulator of Toll-like receptor/Interleukin (IL)-1 receptor (TLR/IL-1R) superfamily of signaling molecules that trigger increased expression of multiple inflammatory genes [46]. Miyata et al. have shown that glucocorticoids suppress bacteria-induced inflammation by directly binding to and up-regulating IRAK-M in airway macrophages and epithelial cells [47]. Additionally, these authors show that IRAK-M depletion results in the enhanced expression of proinflammatory mediators. Thus, glucocorticoids can suppress an overactive inflammatory response via negative feedback to tightly control the inflammatory response and maintain homeostasis.
Overview of Glucocorticoids and the Immune System
The first medical use of glucocorticoids some 60 years ago was for the treatment of rheumatoid arthritis [48, 49]. Since then, glucocorticoids have remained the most commonly used anti-inflammatory and immunomodulatory agents. Their therapeutic activity is substantial in a wide spectrum of diseases, including acute and chronic inflammations, autoimmune disorders, organ transplantations, and the treatment of hematologic cancers [50]. Over the years, numerous publications have focused on glucocorticoids effects on the immune system, and much has been discovered about the molecular mechanism by which GCs act. As in other cells, GR is able to regulate gene expression both positively and negatively in immune cells [9, 18] and can control the inflammatory processes either by a direct binding with glucocorticoid-responsive sequences expressed in the promoters of target genes, or by binding other crucial transcription factors, thus inhibiting the propagation of proinflammatory signals [51]. Furthermore, recent studies have described discrepancies between the immunosuppressive and immunostimulatory effects of glucocorticoids [52, 53].
As described earlier, the inflammatory response is the first protective host response elicited by an injury prompting mobilization of the immune system. The inflammatory recruitment of immune cells neutralizes injurious stimuli and restores the function and structure of damaged tissues [54]. The initial manifestation is the release of intracellular contents after cellular necrosis within the inflammatory site that induces the activation of innate immune components. Through invariant pattern-recognition receptors (PRRs ) , innate immunity is promptly activated upon detection of conserved structures known as pathogen-associated molecular patterns (PAMPs) or damage-associated molecular patterns (DAMPs) [55]. Mast cells and resident macrophages exert different effector functions, one of which is the increased production of proinflammatory molecules such as interleukin-1 and TNF-α, free radicals, histamine, nitric oxide, prostaglandins, and leukotrienes. This increase in proinflammatory molecules results in vasodilatation, capillary permeability, growth of new blood vessels, and leukocyte migration into the inflamed region. The ability of these cells to generate a chemotactic gradient to recruit cells into the injured tissue is rapid; thus, granulocytes and monocyte migrate from blood into tissue within minutes of injury. Among granulocytes, neutrophils are the most important cells at this first stage because of their capacity to destroy invading microorganisms through phagocytosis and microbicidal activity. Pathogenic antigens are engulfed by resident dendritic cells that rapidly differentiate, migrate to lymph nodes, and present antigens to T and B lymphocytes, thus priming and propagating the adaptive immune components including cell-mediated immunity, cytokine production, antigen-specific antibody production, and immunological memory [56].
The resolution of acute inflammation is a dynamic, limited, and finely regulated process that depends upon the crosstalk between innate and adaptive compartments that restore homeostasis after the elimination of harmful agent. An excessive immune response that continues to counteract the persistence of the injurious stimulus triggers a domino effect that leads to chronic inflammation. For this purpose, in the management of numerous mechanisms that control the development and maintenance of inflammation and autoimmune diseases, glucocorticoids have been the most potent drugs of choice, affecting nearly every cell of the immune system, depending on their state of differentiation or activation [57, 58].
Glucocorticoid Effects on Innate Immunity
The innate immune system is the first line of defense that acts rapidly after encountering noxious agents without the reliance on antibody or other acquired responses. For this reason, the effects of glucocorticoids on innate immune cells must be immediate (in terms of minutes) thus contributing to the resolution of inflammation. Among innate immune cells, glucocorticoids strongly influence the plasticity, survival, and function of monocytes and macrophages according to the plasma GC concentration and the state of cell activation [59]. To enhance the clearance of pathogens, dead cells, and toxins, low GC concentrations enhance antigen uptake, scavenger function, and phagocytosis. For this purpose, the induction of the opsonins MFG-E8, Mertk and protein S [60, 61], the up-regulation of mannose receptor MR/CD206 [62], the scavenger receptor CD163 [63], and the increase of IFN-γ-induced FcR [64] have been observed. Glucocorticoids target macrophages to ensure survival in response to LPS-induced sepsis and to suppress inflammation associated with contact allergy [65, 66]. These results suggest that low concentrations of glucocorticoids have an immune-stimulatory effect on macrophage function in the presence of inflammatory stimuli, whereas high concentrations of this stress hormone exert inhibitory functions on macrophages. High dose actions abrogate the production of proinflammatory mediators as numerous cytokines are down-regulated, the secretion of many chemokines is inhibited, the expression of adhesion molecules such as beta-2 integrin is reduced, and antigen presentation and expression of HLA molecules are decreased by GCs [53, 59, 67].
Consistent with the immunomodulatory properties, some studies have shown that steroid hormones induce highly phagocytic monocyte-derived macrophages. Glucocorticoid exposure functions to reprogram monocyte differentiation through changes in intracellular components that regulate cytoskeletal reorganization following adhesion. The enhanced phagocytic activity and increased expression of the anti-inflammatory cytokine IL-10 observed in these cells support the hypothesis that glucocorticoids do not cause a global suppression of macrophages effectors, but result in the differentiation of a specific anti-inflammatory phenotype which seems to be actively involved in the resolution of inflammatory conditions [68–70].
Furthermore, glucocorticoids exert many of their anti-inflammatory effects through the regulation of granulocyte trafficking. GCs can induce apoptosis and degranulation of basophils and eosinophils . However, at the same time GCs promote the survival and expansion of neutrophils increasing the release of bone marrow precursors [71–75]. In the presence of glucocorticoids, the flow and movement of granulocytes appear tightly regulated to that of monocytes. In an inflammatory scenario, endothelial cells increase the expression of adhesion molecules that bind to their cognate receptor on granulocytes thus allowing cellular transmigration into inflammatory sites. To reduce cellular infiltration , GCs promote shedding of L-selectin and E-selectin from neutrophils [76], suppress the synthesis of many chemokines including IL-8, Mip-1β, Mip-3β, Mcp-2, Mcp-3, Mcp-4, RANTES, TARC, and eotaxin, and increase IL-1RII expression, a decoy receptor which limit the deleterious effects of IL-1 [77].
Natural or synthetic glucocorticoids can also alter natural killer (NK) cell activity. Acting as regulatory cells, this homogenous population of innate lymphocytes interacts with various components of the immune system suppressing the immune response [78]. Nevertheless, glucocorticoid treatment is able to reduce NK cell cytolytic activity by the reduction of histone promoter acetylation for perforin and granzyme B. In contrast, glucocorticoids increase histone acetylation in regulatory regions for INF-γ and IL-6. The increase in histone acetylation is associated with increased proinflammatory cytokine mRNA and protein production upon cellular stimulation and epigenetic modifications [79]. These immunologic effects demonstrate how glucocorticoids epigenetically reduce NK cell cytolytic activity, while at the same time prime NK cells for proinflammatory cytokine production that can act as a powerful tool in cancer immunotherapy [80].
Coupling Innate to Adaptive Immunity
While innate immunity provides the first line of defense against pathogens, adaptive immunity, also known as acquired immunity , is also involved during inflammation. Adaptive immunity creates immunological memory after an initial exposure to antigen, resulting in an enhanced antigen-specific response upon subsequent exposure. It is now appreciated that the innate immune response shapes the acquired immune response. The link between the innate and adaptive components involves soluble cytokines and chemokines, and cellular interactions between antigen-specific lymphocytes and antigen-bearing dendritic cells (DCs) . In response to a plethora of stimuli, DCs change from immature cells specialized for antigen capture, processing, and presentation, into mature cells that migrate to draining lymph nodes to interact with naive T cell. On this basis, it is evident that innate immune receptors on DCs play a pivotal role in determining the type of adaptive immune response triggered.
Impairment of DC maturation and function is one of the immunosuppressive effects of glucocorticoids [81]. Synthetic GC treatment interferes with the lifecycle of DCs both in vitro and in vivo [82]. After in vitro maturation with LPS and CD40L, DCs treated with the synthetic glucocorticoid methylprednisolone exhibit enhanced antigen uptake, but down-regulated expression of CD80, CD86, and CD54, and decreased production of TNF-α, IL-6, and IL-12, thus inhibiting the induction of primary T cell responses. Similar results were observed when TNF-α was used to activate DCs [83]. In this study, a different synthetic glucocorticoid dexamethasone, inhibited DC expression of MHC I and II, costimulatory molecules (including B7.1 and B7.2), and the ICAM-1/LFA-1 complex, thus promoting the formation of tolerogenic DCs [82, 84–86]. Tolerogenic DCs are able to drive uncommitted T cells toward the Treg subtype [87] and promote the conversion of CD4+ T cells into IL-10 producing type 1 Tregs (Tr1) [88–90]. Moreover, tolerogenic DCs inhibit the proliferation of allospecific T cells [91, 92], preventing acute graft rejection in mice [93], decreasing the number of IFN-γ producing CD4+ T cells, and promoting NK cell function toward an alternative activated phenotype unable to secrete IFN-γ [94].
Besides their capacity to modulate and induce effector T cell responses, tolerogenic DCs are defined based on the expression of various surface markers such as Ig-like transcript (ILT) molecules [95], transcriptional regulators like glucocorticoid-induced leucine zipper (GILZ) [40, 96, 97], and enzymes such as retinaldehyde dehydrogenase (RALDH) [98] or NO synthetase-2 (NOS-2) [99], all contributing to their functional properties. Additionally, dendritic cells can also facilitate communication between the immune system and the endocrine system. A recent study described a novel DC population in the pituitary gland that produces cytokines, controls LPS-dependent ACTH secretion, and expresses factors for glucocorticoid release [100]. These data suggest that pituitary DCs relay an immune challenge (such as LPS) to the HPA axis by secreting proinflammatory cytokines, which stimulates the anterior pituitary gland to release ACTH. Therefore, DCs are distinguished not only by their role in linking the innate and adaptive immune responses but also in directing communication between the immune and endocrine systems.
Glucocorticoid Effects on Adaptive Immunity
Autoimmune diseases are associated with the generation of an adaptive immune response mounted against self-antigens. During development, most lymphocytes bearing high affinity receptors for self-antigens are deleted, but not all self-reactive lymphocytes are eliminated. The activity of self-reactive lymphocytes is regulated by peripheral tolerance, an active immunosuppressive process that involves clonal anergy and clonal suppression. A failure in peripheral tolerance allows the activation of self-reactive T or B cell clones, thus eliciting (or inducing) cell-mediated or humoral responses against self-antigen. As potent immunosuppressors, synthetic GCs are extensively used for the treatment of various autoimmune and chronic inflammatory conditions [101]. GCs target several aspects of adaptive immunity, including thymocyte maturation , as well as T and B cell proliferation, survival, and differentiation.
Glucocorticoids and Thymocyte Development
The thymus is the key organ for T cell maturation, and glucocorticoids play an important role in thymocyte selection and survival . The selection process drives immature CD4–CD8− “double negative” thymocytes to CD4+CD8+TCRlow “double positive” thymocytes, which represent about 80 % of the cells in the thymus. At this stage double positive thymocytes are extremely sensitive to glucocorticoid-induced apoptosis, but escape apoptosis when both TCR and GR signal simultaneously, according to the “mutual antagonism” model [102]. Thymocytes that are unable to process a functional TCR undergo GC-induced apoptosis, since the TCR signal cannot counteract GR signaling, while only thymocytes expressing a TCR with high affinity for self-peptides undergo negative selection due to the inability of GR signaling to overcome the strong TCR-dependent signal. Finally, only those thymocytes exerting a moderate avidity for self-antigens will survive, suggesting interplay between TCR and GC signals. The grade of affinity between TCR and self-peptides is crucial for the survival of a mature T cell repertoire expressing either the CD4 or CD8 receptor.
A number of in vitro experiments implicate glucocorticoids in regulating T cell number, survival, and TCR repertoire, although the in vivo evidence is still contradictory regarding the correlation between GR expression and thymocyte sensitivity to glucocorticoid-induced death. Adrenalectomy induces thymic hypertrophy [103], mice overexpressing GR in T cells exhibit a reduced number of double positive thymocytes, despite the fact that these cells express lower level of GR compared to thymocytes in other developmental stages. In GILZ-overexpressing transgenic mice, CD4+CD8+ thymocyte number is significantly decreased and ex vivo thymocyte apoptosis is increased [104]. In contrast, intrathymic T cell development and selection proceed normally in mice expressing antisense GR in the thymus and in fetal mice from GR-KO mice [105, 106]. However, these studies suggest the molecular and cellular mechanisms that regulate thymocyte maturation need further investigation.
Moreover, corticosterone synthesis has been suggested to occur in the thymus and there is debate about how locally produced GCs may regulate thymocyte development as well as may affect the initiation of age-associated thymic involution [107].
Glucocorticoid Function in T Cells
Although immature T cells are extremely sensitive to undergoing apoptosis, cell death can also occur in mature T cells either by a glucocorticoid-directed action, or by the involvement of factors that mediate activation-induced cell death , i.e. inhibiting IL-2-mediated activation. According to the activation state and the timing of hormone exposure, T cells can be sensitive or resistant to glucocorticoid-induce cell death. Moreover, mature T cells are susceptible to mutual antagonism between GR and TCR. Mice lacking GR in T cells (GRLckCre) or carrying a point mutation which inhibits GR dimerization and DNA binding (GRdim) demonstrate that inhibition of activation-induced cell death depends on direct binding of the GR to two nGRE s in the CD95 (APO-1/Fas) ligand promoter [108]. In contrast, overexpression of the SWI3-related gene (SRG3) protein in peripheral T cells renders them sensitive to GC-induced apoptosis through the GR–SRG3 complex formation, suggesting that SRG3 may play a critical role in controlling GC-mediated apoptosis of developing thymocytes. Studies have also shown that a dominant negative SRG3 decreases GC sensitivity in thymoma cells. In addition, mice overexpressing the SRG3 protein appear to be more susceptible to stress-induced deletion of peripheral T cells than WT mice, which may result in an immunosuppressive condition [109].
In addition inducing apoptosis, glucocorticoids also affect T cell polarization. Since endogenous or exogenous glucocorticoids attenuate IL-12 synthesis, T cell response is shifted from the Th1 to Th2 phenotype [110]. GCs inhibit both T-bet and GATA-3 transcriptional activity through two different mechanisms. T-bet does not only function as an activator of IFN-γ expression, but also interacts with the GATA-3 transcription factor, inhibiting Th2 cytokine gene expression. Consistent with these results, GCs inhibit both Th1 and Th2 master regulator factors , however long-term treatment favors Th2 expansion [111]. In addition, glucocorticoids induce T polarization toward Th2 phenotype through an increase of Itk expression, a Tec kinase inducing T helper 2 differentiation via negative regulation of T-bet [112, 113].
The effects of GCs on a third subset of IL-17-producing effector T helper cells, called Th17 cells, are still a matter of debate. Dexamethasone inhibits anti-CD3/anti-CD28-stimulated IFN-γ, IL-4, IL-17A, IL-17F, and IL-22 in various cell clones [114]. Interestingly, IL-17A and IL-17F, but not IL-22, lead to resistance of GC-induced apoptosis in in vitro-differentiated Th17 cells despite immunocytochemistry confirming glucocorticoid receptor translocation to the nucleus following treatment [114]. Mice lacking GILZ exhibit severe inflammation and a proinflammatory cytokine expression pattern in the imiquimod model of psoriasis, and DCs lacking GILZ produced greater IL-1, IL-23, and IL-6 in response to imiquimod stimulation in vitro [39]. These studies assessing glucocorticoid-dependent inhibition of IL-17 synthesis are in stark contrast with other studies describing Th17 sensitivity upon GC administration [115–117].
While the role of GC and Th17 is controversial, how glucocorticoids affect Treg function is much clearer. Both in humans and mice, treatment with dexamethasone increases the frequency of Treg cells, suggesting GC-mediated immune suppression is achieved, in part by enhancing Treg cell number or activity [118, 119] and by promoting the development of IL-10-producing T cells, an inducible peripheral Treg subpopulation [120]. There are several mechanisms that have been proposed to explain GC-mediated increase in Treg frequency. First, dexamethasone inhibits IL-2-mediated activation of T effector cells, increasing the proportion of Treg cells [118]; moreover, Treg cells were relatively more resistant to Dex-induced cell death and they were further protected by IL-2 [121]. Second, glucocorticoids synergize with TGF-β in FoxP3 induction [122, 123].
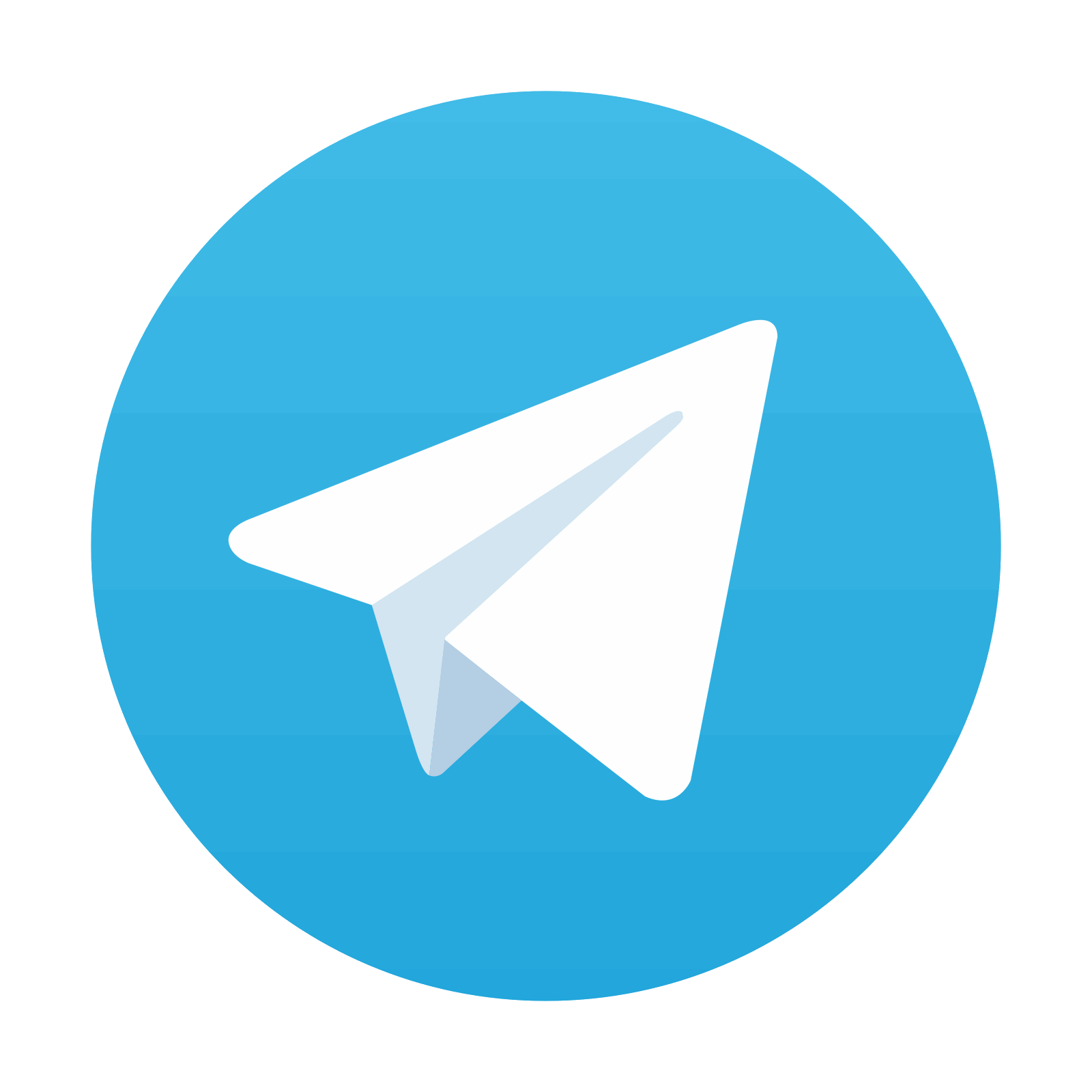
Stay updated, free articles. Join our Telegram channel

Full access? Get Clinical Tree
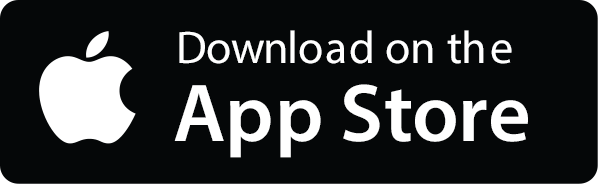
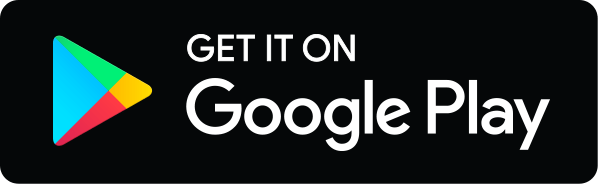