Fig. 1
Histology of pheochromocytoma. a Pheochromocytoma demonstrates a typical nested architecture (H&E, original magnification 100×). b At high power, the pheochromocytoma cells demonstrate a granular amphophilic cytoplasm and moderate nuclear pleomorphism (H&E, original magnification 400×). c Chromogranin immunohistochemistry demonstrates diffuse, strong granular cytoplasmic staining in neoplastic cells (Chromogranin IHC, original magnification 400×). d S100 labels the non-neoplastic sustentacular cells which outline the nests of cells (S100 IHC, original magnification 400×) (images courtesy of Associate Professor Anthony Gill, Anatomical Pathology Department, Royal North Shore Hospital, and University of Sydney)
At present, there are no reliable histological biomarkers that predict malignant tumor behavior. After initial interest in the Ki-67 proliferative index (Bialas et al. 2013; Brown et al. 1999; Clarke et al. 1998; Elder et al. 2003; Jovanovic et al. 2012; Ohji et al. 2001; Semba et al. 2000), a more recent study has not been supportive (Ocal et al. 2014). The Pheochromocytoma of the Adrenal Gland Scaled Score (PASS) was initially developed as a predictor of malignant potential. Calculated out of a maximum of 20 points, it utilizes the following histological features: vascular invasion (1 point), capsular invasion (1), periadrenal adipose tissue invasion (2), large nests or diffuse growth (2), necrosis (2), high cellularity (2), tumor cell spindling (2), cellular monotony (2), increased mitotic figures (2), atypical mitotic figures (2), profound nuclear pleomorphism (1), and hyperchromasia (1). A score of <4 was reported as being likely benign, while ≥4 increased the likelihood of malignancy. Not all studies supported a correlation between PASS and clinical outcome, however (Agarwal et al. 2012; Jovanovic et al. 2012; Mlika et al. 2013; Ocal et al. 2014; Thompson 2002). New biomarkers that predict malignant tumor behavior are required.
While at present the majority of PCs are still considered sporadic tumors, studies of the genetics of tumorigenesis have led to recognition that over 30 % are associated with predisposing germline mutations, as part of various familial syndromes (Dahia 2014; Favier et al. 2014). Re-arranged in transfection (RET) mutations in MEN2 syndromes are associated with the development of PC. Individuals who are known to carry a RET mutation should undergo regular screening for PC from a young age, discussed below, with the goal of identifying presymptomatic disease that is readily surgically resectable, thus hopefully improving outcomes. Most MEN2-associated PCs are benign, but for those who do develop metastatic disease, treatment options are limited.
2 The Phenotype of MEN2-Associated Pheochromocytomas
Approximately 40 % of MEN2 patients will develop PC, although the likelihood varies with the particular RET mutation (Brandi et al. 2001; Raue et al. 1994). Medullary thyroid cancer (MTC) is typically the first identified feature of MEN2, while PC is rarely the presenting condition, reportedly diagnosed before MTC in only 12–25 % of cases (Modigliani et al. 1995b; Rodriguez et al. 2008; Welander et al. 2011).
MEN2-associated PCs generally arise in the adrenal glands, while paragangliomas (PGLs) are rare (Welander et al. 2011). As with other hereditary PCs, MEN2-associated PCs are more commonly multifocal and bilateral than sporadic PCs. Hereditary PCs also tend to be diagnosed earlier than sporadic PCs. Among the hereditary PC syndromes, those associated with causative genes in the kinase pathway, such as in MEN2 syndromes and neurofibromatosis, present later than those associated with causative genes in the oxygen sensing pathway, such as succinate dehydrogenase subunit B (SDHB)-associated hereditary PC/PGL syndromes and von Hippel–Lindau (VHL) disease (40 vs. 31 years p < 0.001) (Eisenhofer et al. 2011b).
MEN-2-associated PCs secrete epinephrine as the predominant catecholamine, along with its metabolite metanephrine, and lesser amounts of norepinephrine and normetanephrine. (Modigliani et al. 1995a) This biochemical secretion profile contrasts to sporadic, VHL-, SDHB- and SDHD-associated PCs, in which secretion of norepinephrine typically predominates (Benn et al. 2006; Neumann et al. 2002; Opocher et al. 2005). Catecholaminergic symptoms are characteristically more pronounced in MEN2-associated PCs, compared to VHL-associated PCs, due to the association of increased epinephrine with greater anxiety, palpitations, and episodic hypertension (Frank-Raue et al. 1996; Kaltsas et al. 2004; Pacak 2007). PC is malignant in MEN2 in less than 5 % of cases (Gimm et al. 2004), in contrast to PCs in the SDHB-associated hereditary PC/PGL syndrome where malignancy is more common (Favier et al. 2014; Gimenez-Roqueplo et al. 2012).
A study of 85 patients with MEN2-associated PCs included 70 patients with MEN2A and 15 with MEN2B (Thosani et al. 2013). The median age at diagnosis of PC was 32 years. The initial manifestation of MEN2 was MTC in 60 % of patients, synchronous MTC and PC in 34 % and PC alone in 6 %. Seventy % of patients had bilateral PC and 82 % of these PCs were synchronous tumors. The median time between MTC and PC diagnosis was 5.4 years and the median time between diagnosis of first and second PC in those with metachronous PC presentation was 9.4 years (range 1.8–20.7 years). The commonest PC-associated RET mutation in MEN2, as discussed below, is RET codon 634, such that 50 % of these patients will develop PC. Other mutations associated with PC include codons 918, 883 (both associated with MEN2B), 630, and 666. There is a lower penetrance of PC in patients with exon 10 RET mutations (e.g., codons 609, 611, 618, and 620). Among the RET codon 634 mutation carriers in Thosani’s study, the presence of PC was not associated with a later stage of MTC at the time of MTC diagnosis. Interestingly, the median survival of codon 634 MEN2 patients with PC was 499 months versus 444 months for those without PC (p < 0.05, albeit with a short follow- time for the latter group in this study). PC was unlikely to be a cause of death as only 2 patients in the study died of PC; these deaths were related to hypertensive crises either under anesthetic or with IV contrast administration. There were no cases of metastatic PC among the MEN2-associated PCs in this study.
3 The Molecular Genetics of Pheochromocytomas in Multiple Endocrine Neoplasia 2
PCs and PGLs represent the most heritable of all tumor types. The inherited predisposition to PC/PGLs was first recognized in 1993 and initially genetic mutations were thought to cause only 10 % of all PC/PGLs. Since then, a larger number of predisposition genes have been identified, such that more than 30 % of all patients presenting with PC and/or PGL will be found to carry a germline mutation (Dahia 2014; Favier et al. 2014).
To date, more than fourteen genes have been identified that show germline mutation in association with the development of PC/PGL. As well as the RET gene in MEN2 syndromes, mutations have been found in neurofibromatosis type 1 (NF1), Von Hippel–Lindau (VHL) disease, succinate dehydrogenase subunits A, B, C, and D (SDHA, SDHB, SDHC, and SDHD, collectively SDHX), succinate dehydrogenase assembly factor 2 (SDHAF2), prolyl hydroxylase domain protein enzyme 1 and 2 (PHD1 and PHD2), transmembrane protein 127 (TMEM127), MYC-associated factor X (MAX), kinesin family member 1B beta (KIF1Bß), endothelial PAS domain protein 1 (EPAS1, encoding HIF2 α), and fumarate hydratase (FH) genes. Interestingly, these genes encode proteins with diverse cellular function, but result in histologically identical tumors. A key paper by Dahia et al. (2005) divided tumors into two groups. Cluster 1 mutations were associated with upregulated hypoxia inducible factors (HIF) and other hypoxia responsive genes, whereas Cluster 2 mutations resulted in activation of protein kinase signaling pathways. Cluster 1 includes VHL, SDHx, and PHD1 and PHD2, while cluster 2 comprises a more heterogenous group of genes including RET, NF1, TMEM127, and MAX (Burnichon et al. 2012; Dahia et al. 2005; Qin et al. 2010).
MEN2 results from a gain of function mutation in the RET proto-oncogene on chromosome 10q11.2, a 21 exon gene that encodes a tyrosine kinase receptor. This gene was initially discovered in 1985 (Takahashi et al. 1985). The receptor consists of an extracellular domain, a transmembrane domain, and an intracellular domain. Activation by ligand binding induces dimerization and downstream signaling via P13K-AKT and MAPK-ERK kinase pathways to modulate cell growth and differentiation (Treanor et al. 1996). RET mutations result in constitutive activation of the tyrosine kinase (Welander et al. 2011). These mutations are clustered in exons 8, 10, 11, 13, 14, 15, or 16.
4 Genotype and Phenotype Correlations in MEN2-Related Pheochromocytomas
Systematic analysis of kindreds affected by MEN2 has led to recognition of the strong genotype –phenotype relationship between RET mutations and specific clinical manifestations, including the age of onset and aggressiveness of MTC, and the likelihood of PC and primary hyperparathyroidism. This genotype–phenotype correlation has permitted the development of risk stratification guidelines that advise the timing of prophylactic thyroidectomy in asymptomatic RET mutation carriers, as discussed in Chap. 9, with the goal to remove the thyroid gland prior to the development of MTC. Different RET codon mutations are associated with variable penetrance of PC and primary hyperparathyroidism, and this can guide the age at which screening for PC and primary hyperparathyroidism should commence. The most recent American Thyroid Association (ATA) guidelines (Wells et al. 2015) have updated the 2009 guidelines (Kloos et al. 2009) which divided RET mutations into four levels of risk (A < B < C < D). ATA-D mutations (codons 918 and 883) are the most severe, being associated with MEN2B and the development of aggressive MTC in childhood, such that thyroidectomy is recommended within the first year of life for carriers. The 2015 guidelines refer to the highest risk category as “ATA-HST.” MEN2B is associated with the development of PC in around 50 % of cases, while primary hyperparathyroidism is not seen. The high risk ATA-C group comprises RET codon 634 mutations. Codon 634 mutations are associated with an MEN2A phenotype, characterized by the early development of aggressive MTC, and a high risk of development of PC and hyperparathyroidism. The 2015 guidelines refer to this category as ATA-H. By contrast, the lower risk ATA-B and ATA-A groups have been grouped into a single category of ATA-MOD, which consists of a number of RET codon mutations associated with a more variable phenotype. The ATA-MOD group is associated with the development of less aggressive medullary thyroid cancer, such that prophylactic thyroidectomy may be delayed beyond the age of 5 if certain strict clinical and biochemical criteria are met (Kloos et al. 2009). PC is seen less frequently in individuals with ATA-A and ATA-B (ATA-MOD) risk group RET mutations. Interestingly, PC can present at widely different ages in different members of “low PC risk” FMTC families with the same mutation (e.g., RET codon G533C). This has led the latest guidelines to recommend removal of the separate FMTC category which should be regarded now as a “low PC risk” variant of MEN2A.
Collaborative studies reporting the clinical data of MEN2-affected kindreds continue to provide an evidence base to guide age-dependent screening for PC. The highest risk RET mutations for the development of PC include 918, 634, and 630. Individuals with MEN2B carrying RET codon 918 mutations have a reported incidence of PC of around 50 %. Individuals carrying RET codon 634 mutations have been similarly reported to develop PC in around 50 % of cases, most frequently in the 3rd or 4th decade of life (Thosani et al. 2013). Guidelines published by the ATA proposed annual screening for PC commence at 11 years for the higher risk codon 918, 634, and 630 mutations (ATA-HST and ATA-H, and at 16 years for other mutations ATA-MOD) (Kloos et al. 2009; Machens et al. 2005). Biochemical screening for PC is discussed below.
5 Diagnosis of Pheochromocytoma
The diagnosis of PC is based on the clinical suspicion, biochemical testing, and then imaging studies (if biochemistry is positive), leading to surgery and histological evaluation (Lenders et al. 2014). Gene carriers in MEN2 families must not undergo general anesthetic for any surgery without prior PC screening. Episodic secretion of catecholamines by PC mean that the metabolites metanephrine and normetanephrine are more sensitive integrated measures of catecholamine production. RET mutation carriers should be screened at least annually for PC, with biochemical screening using either plasma metanephrines and normetanephrines (drawn from a supine patient after an overnight fast), or 24-h urinary fractionated metanephrines and normetanephrines (Eisenhofer et al. 2003). The recommended method of catecholamine measurement is via liquid chromatography with mass spectrometry (Eisenhofer and Peitzsch 2014; Weismann et al. 2014). Controversy persists regarding the relative merits of plasma versus urinary metanephrine measurements in screening for PC (Lenders et al. 2014), and in practice plasma and urine testing are complementary. Catecholamine levels have been shown to correlate poorly with tumor size, unlike plasma or urine catecholamine metabolite levels, which show a strong correlation (Eisenhofer et al. 2004b).
As discussed above, there are distinct patterns of plasma catecholamine o-methylated metabolites in patients with the hereditary PC syndromes. MEN2-associated PCs express phenylethanolamine N methyltransferase (PNMT), the enzyme that converts norepinephrine to epinephrine, hence the association with predominant epinephrine secretion and elevated metanephrines (Pacak et al. 2009). NF1-associated PCs show a similar profile, while the hypoxia gene (VHL and SDHB/D)-associated PCs produce elevated normetanephrines and methoxytyramine levels (Eisenhofer et al. 2011a).
In practical terms, MTC has generally developed and resulted in elevated plasma calcitonin levels by the time PCs present clinically in MEN2. Plasma-free metanephrine measurement has a sensitivity of 96–100 % and a specificity of 87–92 %, while 24-h urine fractionated free metanephrine measurement has a sensitivity of 92–99 % and specificity of 64–72 % in the diagnosis of PC (Sawka et al. 2003). Certain drugs are known to cause false elevations in catecholamines, including tricyclic antidepressants, beta blockers, phenoxybenzamine, and monoamine oxidase inhibitors. It is best to avoid these and retest subjects where possible. Medical conditions, including renal impairment and states of increased sympathetic activity such as heart failure and hypoglycemia, can also cause a physiological elevation in catecholamines. A number of provocation tests have been trialed in the past, including glucagon stimulation tests, clonidine suppression tests, and histamine stimulation tests (Lenders et al. 2010; Young 1997), but these have become largely redundant since advent of fractionated metanephrine testing. Serum chromogranin A is elevated in 48 % of patients with PC (Cotesta et al. 2005). Diagnostic utility of chromogranin A is, however, constrained by poor specificity due to its elevation in diverse conditions, including other neuroendocrine tumors, hyperparathyroidism, hyperthyroidism, gastrointestinal disorders including hepatitis, colon cancer and pancreatic disorders, cardiovascular disease, inflammatory diseases, renal failure, other cancers and the use of proton pump inhibitors (Modlin et al. 2010).
Once a biochemical diagnosis has been established, or in cases when there are symptoms of structural compression, anatomical and often functional imaging tests are required. Structural imaging for diagnosis of PC and PGL includes the use of CT and/or MRI. CT is usually the first choice for imaging of the chest, abdomen, and pelvis, whereas MRI is superior for imaging head and neck PGLs, more relevant to the hereditary PGL syndromes (Lenders et al. 2014). PCs enhance avidly after CT contrast administration and display a prolonged contrast washout phase with Hounsfield unit scores usually >30 (Ilias et al. 2007). CT has a sensitivity of 93–100 % for detecting tumors >0.5 cm diameter. An example of adrenal CT imaging in a man with known MEN2A syndrome who was found to have elevated urinary metanephrines on routine annual biochemical screen is shown in Fig. 2a. A second example of CT imaging in a patient presenting clinically with pheochromocytoma in MEN2A is shown in Fig. 2b. MRI is equally sensitive and may provide detail of the relationship between the tumor and its vasculature (Jacques et al. 2008). MRI is also recommended if there is an allergy to CT contrast dye, if there are surgical clips present, and to limit radiation exposure when imaging children. Most PCs will have heterogeneous, multiple high-intensity areas seen on T2 weighting, although this is not a universal finding. The specificity of CT and MRI varies in studies from 50 to 90 % (Mittendorf et al. 2007). False positivity can occur, especially when there has been previous surgery in the same location. Functional imaging is then needed to complement anatomical imaging.
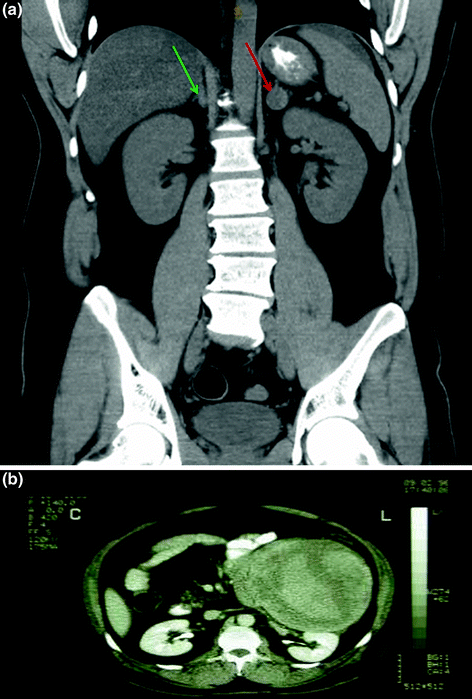
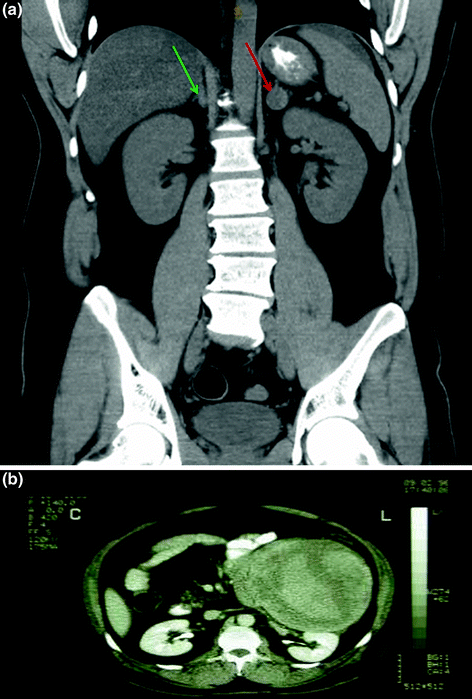
Fig. 2
Computer tomography (CT) of MEN2A-mutated pheochromocytoma. a Non-contrast CT coronal section through the abdomen of a patient with known MEN2A mutation, showing a 20-mm lesion in the left adrenal gland, Hounsfield units 38 (red arrow), and a 10-mm lesion in the right adrenal gland, Hounsfield units 35 (green arrow). b Contrast CT transverse section through the upper abdomen of a 60-year-old man who presented with flank pain, hypertension, and hemodynamic instability, showing a large left-sided retroperitoneal mass with extensive hemorrhage. Histopathology revealed a 2.1-kg pheochromocytoma. The patient was confirmed to have MEN2A syndrome, carrying a RET codon 608 mutation (case previously published in Learoyd et al. 2005; Evans et al. 1997)
Functional studies aid in the detection of extra-adrenal PGLs and of metastatic disease (Taieb et al. 2014). Functional imaging is therefore generally recommended for patients who are at risk of multiple tumors or metastases, who have a large primary tumor >10 cm, or where there is suggestion of recurrent disease (Lenders et al. 2014; Whalen et al. 1992). Historically, functional studies relied upon 123I-metaiodobenzylguanidine (123I-MIBG) scintigraphy. MIBG is a norepinephrine-like compound taken up by the human norepinephrine transporter (hNET) expressed in chromaffin tissue (Fig. 3a). Negative MIBG uptake in a structural lesion does not exclude the diagnosis of PC, particularly if the tumor is <3 cm (Kurisaki-Arakawa et al. 2014). MIBG scanning using either I123 or I131 has sensitivities of 60–95 %; I123 produces better image quality (albeit at higher cost) than I131. MIBG scanning displays high specificity of 95–100 % but is less sensitive for metastatic or extra-adrenal disease. The specificity is complicated by the fact that up to 50 % of normal adrenal glands will demonstrate some physiological uptake of MIBG. Certain drugs will need to be withheld for 2 weeks prior to MIBG scanning including calcium channel blockers, tricyclic antidepressants, sympathomimetics, and combined alpha/beta blockers. MIBG imaging is particularly relevant when I131 MIBG is planned for therapy (Fig. 3b).
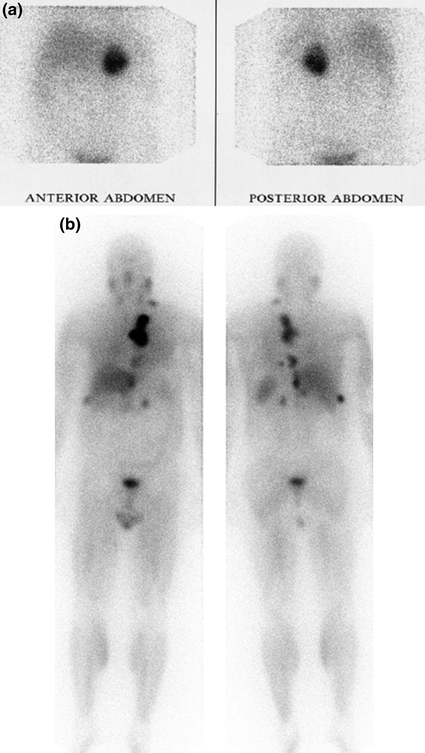
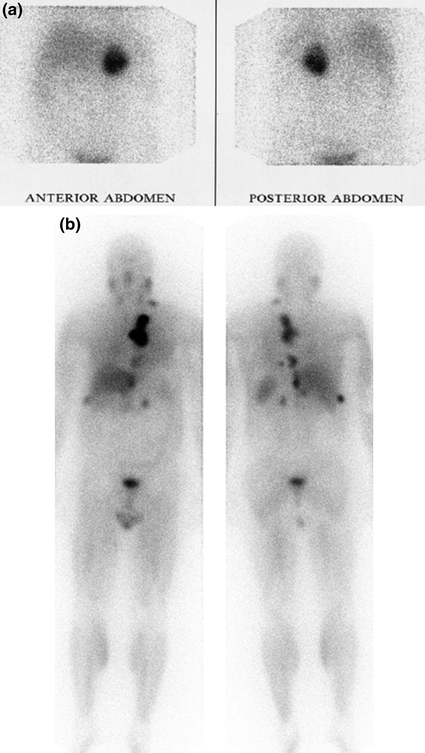
Fig. 3
131I-metaiodobenzylguanindine (I-131 MIBG) scintigraphy of RET-mutated pheochromocytomas. a Planar I-131 MIBG scintigraphy showing left-sided pheochromocytoma, with increased tracer accumulation. b Whole body I-131 MIBG scintigraphy following an 8 GBq therapeutic dose in a 52-year-old man with MEN2A, revealing metastatic deposits of MIBG-avid pheochromocytoma in the lungs, bones, and intra-abdominal viscera (images courtesy of Associate Professor Paul Roach, Nuclear Medicine Department, Royal North Shore Hospital, and University of Sydney)
In recent years, positron emission tomography (PET) scanning has been increasingly studied, generally in combination with CT to enhance diagnostic accuracy. In imaging PC/PGL, these studies have evaluated the widely utilized, non-specific tracer 18F-fluorodeoxyglucose (18F-FDG), as well ligands specific to catecholamine metabolic pathways, such as 18F-fluorodopamine and 18-fluoro-dihydroxyphenylalanine (18F-DOPA). 18F-FDG PET/CT is less specific, but is useful in detecting metabolically active lesions, particularly metastatic disease with high glucose metabolism. 18F-FDG PET/CT is also more useful than MIBG in non-functional PGL (Ilias et al. 2003a; Lenders et al. 2014; Timmers et al. 2012). 18F-FDG PET/CT may also be particularly useful for imaging SDHB-mutated tumors (Lepoutre-Lussey et al. 2015).
The PET isotope 18F-fluorodopamine is taken up into catecholamine-producing cells by hNET, and initial studies showed promise with excellent sensitivity in PC/sympathetic PGL imaging; its utility is limited by availability. 18F-fluorodopa enters neuroendocrine cells via an amino acid transporter and has also been reported to be a sensitive PET tracer for PC/PGL (Hoegerle et al. 2002; Ilias et al. 2003b).
PCs and PGLs also express somatostatin receptor subtypes 2, 3, and 5 (Mundschenk et al. 2003; Reubi et al. 1992), an observation utilized in functional imaging studies. Conventional 111-Indium pentetreotide scintigraphy has shown less utility in PC/PGL, while newer PET-specific 68Gallium-somatostatin analogues, such as 68Ga-DOTATATE (Hofman et al. 2015), DOTANOC (Lopci et al. 2013), and DOTATOC, are being explored and show promise in early studies (Rufini et al. 2013). An image of a 68Ga-DOTATATE scan showing a left-sided pheochromocytoma is shown in Fig. 4.
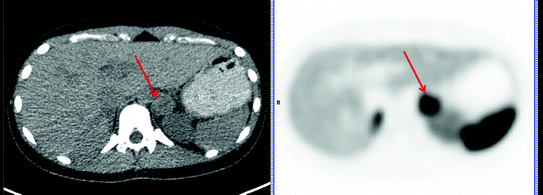
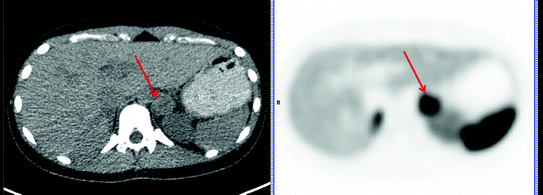
Fig. 4
68Gallium-somatostatin analogues (68Ga-DOTATATE) positron emission tomography (PET) scan. Ga68-DOTATATE PET scan in a patient with MEN2A showing a left-sided pheochromocytoma (red arrow), with physiological uptake in the right adrenal for comparison. Splenic uptake is also physiological (images courtesy of Associate Professor Paul Roach, Nuclear Medicine Department, Royal North Shore Hospital, and University of Sydney)
The “flip-flop” effect that describes tumor heterogeneity, whereby rapidly progressive metabolically active tumor cells are better imaged with 18F-FDG PET/CT, while better differentiated cells show greater avidity for 68Gallium- DOTA- or 18F-fluorodopa, may not be as applicable to MEN-2 associated PCs as it is in the imaging of other neuroendocrine tumors.
6 Treatment
6.1 Perioperative Management of PC
Once a diagnosis of PC is made, surgical resection is the only curative option. Thus, early diagnosis of a solitary lesion is most likely to result in long-term remission. Lifelong follow-up is always required, as currently there are no reliable predictors of malignancy, and a second PC may present years later in MEN2. The latest ATA guidelines recommend that PC surgery should take place prior to thyroidectomy in MEN2 patients where MTC and PC are diagnosed simultaneously. It is also recommended that PC should be removed prior to embarking on pregnancy (Wells et al. 2015).
Preoperative medical treatment is required to prevent perioperative catecholaminergic crisis. The goals are to treat hypertension, expand the contracted plasma volume, and stabilize the heart rate in order to minimize hemodynamic disturbance both during induction of anesthesia and manipulation of the tumor and also following its resection. This should first be done using alpha-adrenergic receptor blockade (Pacak 2007; Weingarten et al. 2010; Young 1997). There are no randomized controlled trials comparing the non-selective alpha-adrenergic blocker phenoxybenzamine to a selective alpha-1 blocker, such as prazosin, doxazosin, or terazosin. The non-competitive, irreversible alpha blockade provided by phenoxybenzamine does risk greater postoperative hypotension and, for this reason, some centers prefer use of a shorter-acting, competitive, selective alpha-1 blocker such as doxazosin. At least 7–14 days of alpha blockade treatment are typically required to achieve target blood pressure control of <130/80 mmHg lying and <100 mmHg systolic standing and to facilitate adequate blood volume expansion. Phenoxybenzamine is usually commenced at 10 mg twice daily, increasing every 2–3 days as tolerated up to a maximum of 1 mg/kg. Prazosin is administered as 2–5 mg two to three times a day, terazosin 2–5 mg/day, and doxazosin 2–8 mg/day (Pacak 2007). Beta blockade must never be started before adequate alpha blockade has been achieved, but may be required to control reflex tachycardia that develops, particularly with epinephrine-secreting tumors (Crago et al. 1967; Sibal et al. 2006; Wark and Larkins 1978). Cardioselective beta1 adrenoreceptor blockers are preferred, such as atenolol 12.5–25 mg two to three times a day or metoprolol 25–50 mg three to four times a day. Combined alpha and beta blockers should be avoided, as the ratio of alpha:beta favors beta blockade and may trigger a catecholaminergic crisis. Labetalol, for example, provides an alpha:beta ratio of 1:5. If blood pressure and/or heart rate is unable to be controlled with these agents, or if patients have major side effects from alpha and beta blockade, other options for preoperative management include calcium channel blockers, such as amlodipine 10–20 mg, nifedipine 30–90 mg daily, or verapamil 180–450 mg daily (Lenders et al. 2014; Pacak 2007). Alternatively, calcium channel blockers may be used as additional agents in patients already alpha blockade. Use of calcium channel blockers as first-line therapy has been successful in patients with very mild hypertension, but this approach is discouraged unless the patient is very intolerant of alpha blockade.
To avoid postoperative hypotension, the goal is to achieve plasma volume expansion during the period of alpha blockade, both by encouraging increased oral sodium intake, and possibly with intravenous saline loading in the day prior to surgery. Practice varies between institutions, and randomized trials supporting this therapy are lacking. Blood pressure should be carefully monitored during surgery and in an intensive care unit postoperatively (Lenders et al. 2014; Pacak 2007).
6.2 Surgical Approach
It is crucial that patients are referred to a center experienced in adrenal surgery. Most such centers use minimally invasive (laparoscopic) adrenalectomy for PCs, although an open approach may be required for tumors larger than 6 cm in order to ensure complete resection and to avoid capsule rupture within the abdomen (Lenders et al. 2014). Laparoscopic approaches have only been compared in single center series and generally show better outcomes including less pain, less blood loss, fewer days in hospital, without difference in mortality or recurrence (Agarwal et al. 2012; Shen et al. 2010). Perioperative mortality is approximately 1 % (Agarwal et al. 2012; Shen et al. 2010). A newer retroperitoneal approach has shown markedly less postoperative pain resulting in shorter hospital stays (Dickson et al. 2011; Epelboym et al. 2014; Walz et al. 2006; Yuan et al. 2014). Partial adrenalectomy has also been used in highly experienced centers, to spare the cortex and allow patients with bilateral disease or inherited syndromes to retain partial adrenocortical function (Iihara et al. 2003). This warrants further assessment in larger studies of MEN2-affected individuals, where malignant PC is unlikely but bilateral disease very common, and where adrenal insufficiency is inevitable after bilateral adrenalectomy. The cumulative recurrence rate for MEN2-associated PCs after adrenal-sparing surgery at 5 and 10 years is 38.5 %, including both ipsilateral and bilateral recurrence (Asari et al. 2006).
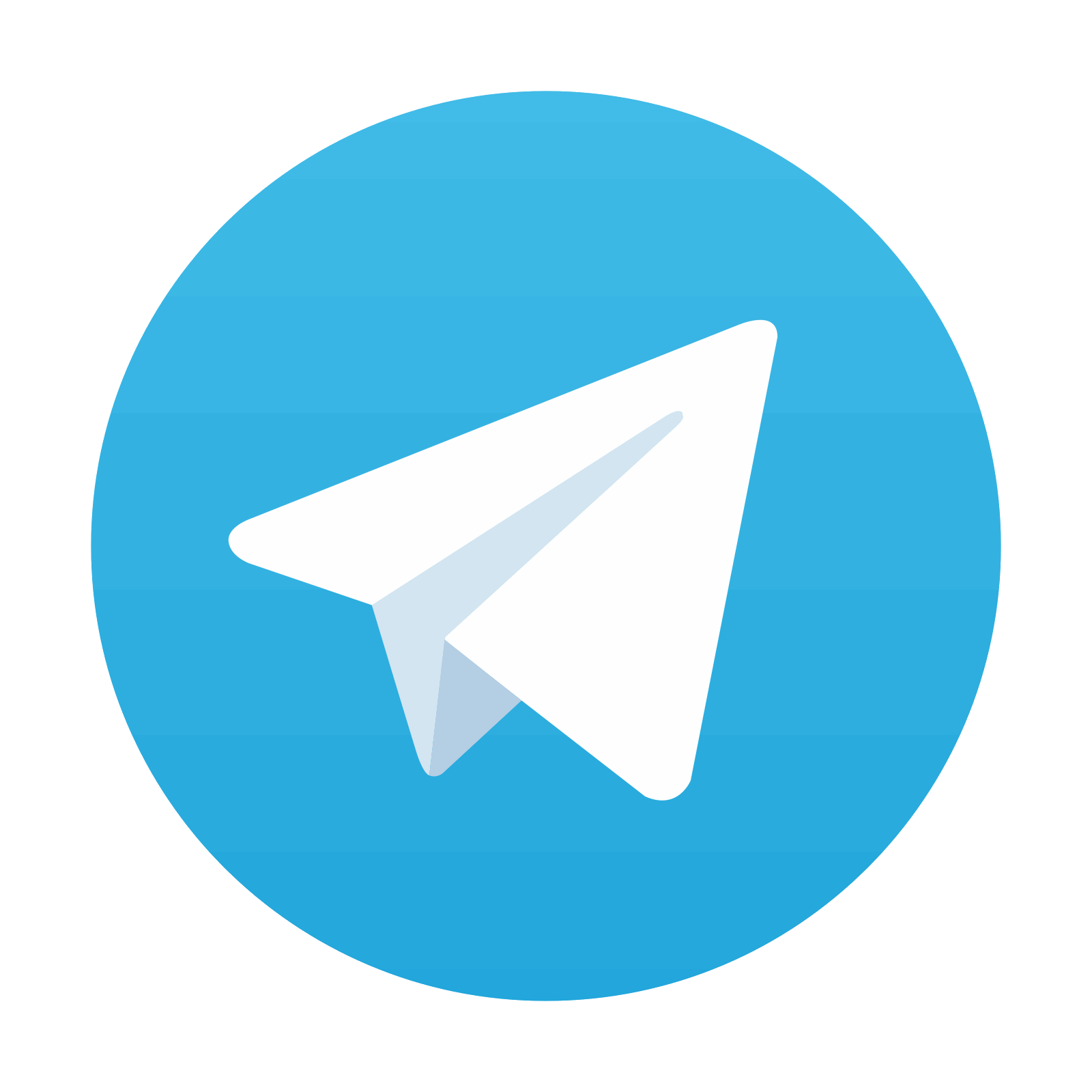
Stay updated, free articles. Join our Telegram channel

Full access? Get Clinical Tree
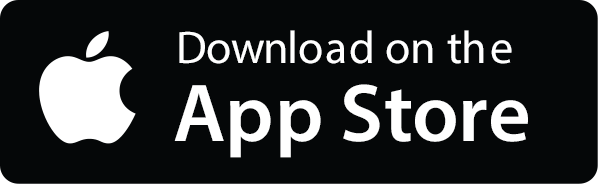
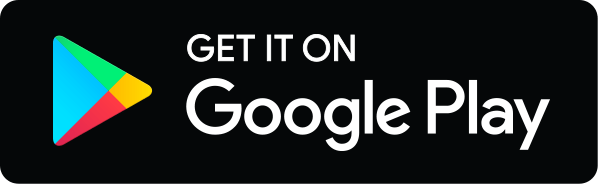