Andrea V. Page, W. Conrad Liles
Immunomodulators
Antimicrobial agents and vaccines are the traditional strategies employed for treatment and prevention of infectious diseases. Although both approaches have been remarkably successful, many infectious diseases continue to pose difficult clinical problems. Treatment may be hampered by defects of the immune system resulting from an underlying disease or immunosuppressive medications, and enhancement or reconstitution of the host immune response may be a prerequisite to long-term cure. In contrast, it is the aggressive host immune response that mediates inflammation and tissue damage in syndromes such as sepsis, and in this case, downregulation of the host immune response, at least in the initial stages of the disease, may be beneficial.
An immunomodulator is a biologic or nonbiologic agent that alters the host immunoregulatory response. This response results from the actions and interactions of a complex network of cells and soluble mediators from both the innate and acquired arms of the immune system. As a result, immunomodulators can affect or influence a wide variety of targets. Immunomodulators currently in use can be divided into six main groups: (1) naturally occurring cytokines, many of which are produced by recombinant DNA technology, including the colony-stimulating factors (CSFs), interferons (IFNs), interleukins (ILs), and thymic hormones; (2) monoclonal antibodies that block the action of proinflammatory cytokines, components of the complement cascade, or the pathogen itself; (3) immunoglobulins, used either as replacement therapy in immunoglobulin-deficient individuals or as true immunomodulators to upregulate or downregulate the immune response; (4) glucocorticosteroids; (5) synthetic compounds with immunomodulatory activity, such as the 3-hydroxy-3-methylglutaryl coenzyme A (HMG-CoA) reductase inhibitors (statins), pentoxifylline, imiquimod, and thalidomide; and (6) immunomodulatory cell-based therapies, including mesenchymal stromal/stem cells (MSCs).
This chapter focuses on agents that have been used in an effort to manipulate the immune system for the treatment or prevention of infection in humans. Many potentially useful immunomodulators have been investigated in vitro or in experiments involving animal models of infection. However, because of the complexity of the host immune response, in vitro data may not correlate with in vivo results, and animal models have inherent limitations compromising their applicability to human disease. Therefore, this chapter is limited to immunomodulatory agents that have been investigated in clinical trials in humans. As knowledge of the molecular pathogenesis of inflammation, immunity, and infection evolves, novel immunomodulatory therapeutics and refinement of current immunomodulatory approaches are expected to emerge and change both the management and outcome of challenging infectious diseases.
Colony-Stimulating Factors
The CSFs are a group of naturally occurring glycoprotein cytokines that regulate the production, differentiation, survival, and activation of hematopoietic cells. Erythropoietin stimulates red blood cell production and is widely employed clinically for the treatment of anemia. Thrombopoietin plays a key regulatory role in the growth and differentiation of megakaryocytes. IL-5 stimulates the growth and survival of eosinophils and basophils. Stem cell factor (SCF, formerly called Steel factor or c-kit ligand) and IL-3 (formerly called multicolony-stimulating factor or hematopoietic cell growth factor) are stimuli for multiple hematopoietic cell lineages. Granulocyte colony-stimulating factor (G-CSF), granulocyte-macrophage colony-stimulating factor (GM-CSF), and macrophage colony-stimulating factor (M-CSF) act as growth factors for specific cell types in the myeloid series and have attracted considerable interest as immunomodulators.
Granulocyte Colony-Stimulating Factor
G-CSF is a glycoprotein that acts almost exclusively on neutrophils (polymorphonuclear leukocytes) and neutrophilic precursors to promote cell growth, differentiation, and function.1 It is produced predominantly by monocytes/macrophages, fibroblasts, and endothelial cells and serves as a potent stimulus to both increase and accelerate neutrophil production.1 The essential role of G-CSF in normal regulation of neutrophil development has been clearly demonstrated in studies of G-CSF knockout mice, which develop chronic neutropenia, with a 50% reduction in the number of granulocyte precursor cells in the bone marrow; fail to develop sepsis-related neutrophilia; and demonstrate a diminished capacity to control experimental Listeria monocytogenes infection.2 Endogenous G-CSF also influences the functional activity of developing and mature neutrophils, enhancing the inducible oxidative (respiratory) burst, phagocytosis, and chemotaxis, and delaying spontaneous apoptosis.3–5
Recombinant G-CSF is available for clinical use in three forms: filgrastim, a nonglycosylated polypeptide produced in Escherichia coli; pegfilgrastim, a pegylated form of filgrastim with a long half-life and single-dose administration; and lenograstim, a glycoprotein produced in Chinese hamster ovary cells that bears the greatest resemblance to the naturally occurring molecule. A number of filgrastim biosimilar agents have recently been approved for use, one in the United States and at least five in Europe, and long-term outcome data on safety and efficacy are still being collected.6 For the purposes of this chapter, therefore, only the three original (reference) forms of G-CSF are discussed.
When administered in vivo, recombinant human G-CSF initially causes a transient decrease in the peripheral blood neutrophil count, followed by a sustained dose-dependent increase that persists while serum G-CSF concentrations remain elevated.7 After a single subcutaneous dose of G-CSF, the highest neutrophil count is reached in approximately 12 hours (for filgrastim) or 48 hours (for pegfilgrastim), after which there is a slow decline over 2 to 3 days in patients receiving filgrastim or over 1 week or longer in those receiving pegfilgrastim.8 The increase in neutrophil count results from an enhanced rate of granulopoiesis combined with a shortened maturation time, culminating in increased release of immature neutrophil precursors from the bone marrow. In addition, recombinant G-CSF may also produce slight increases in monocyte and lymphocyte counts and relatively modest declines in platelet counts.9
Because of its potent ability to increase neutrophil counts, G-CSF has emerged as an important therapeutic option for a variety of neutropenic conditions. In primary (congenital) neutropenic disorders, such as severe congenital neutropenia, cyclic neutropenia, and idiopathic neutropenia, the absolute neutrophil count (ANC) may remain less than 0.5 × 103/mm3 for months or years, thereby placing patients at risk of recurrent and severe bacterial and fungal infections. In this patient population, routine prophylactic administration of G-CSF significantly increases the ANC, accompanied by concomitant reduction in infection, antibiotic use, and hospitalization.10 As a result, there has been widespread consensus that prolonged G-CSF therapy represents the standard of care for primary neutropenic disorders.11 It should be noted, however, that clinical experience with G-CSF in congenital neutropenia has been largely limited to filgrastim and lenograstim, and that some investigators have reported more adverse events and reduced efficacy with pegfilgrastim in this patient population.12
The most common indication for use of G-CSF is the treatment of neutropenia after cancer chemotherapy, in which the risk of infection is related to both the degree and duration of neutropenia, and opportunistic bacterial and fungal infections continue to be a major cause of morbidity and mortality. The risk of an episode of severe (absolute neutrophil count < 0.5 × 103/mm3) or febrile neutropenia during a first cycle of chemotherapy has been estimated at 24% in the absence of G-CSF, and each episode of febrile neutropenia has been shown to carry an in-hospital mortality rate of approximately 10%.13,14 Therefore, G-CSF has been used to both prevent and shorten febrile neutropenic episodes.15 G-CSF administration for primary prophylaxis of neutropenia has been studied in multiple patient populations. However, because individual randomized controlled trials (RCTs) enrolling patients with specific malignancies may be underpowered to detect any potential effect on clinically relevant outcomes such as mortality, most of the recent data are derived from meta-analyses and systematic reviews, some of which combine data from studies using either G-CSF or GM-CSF. A reduction in the incidence of infection when G-CSF is administered for primary prophylaxis in adult patients who are undergoing chemotherapy for solid tumors or lymphoma has been demonstrated in one such meta-analysis.16 Importantly, a reduction in both infection-related and all-cause mortality was also reported. However, a second meta-analysis including only those studies of patients with lymphoma found no reduction in mortality during the chemotherapy period, although the reduction in episodes of febrile neutropenia and documented infection remained significant.17 Similarly, a large meta-analysis of 148 studies of a mixed population of adults and children undergoing chemotherapy for solid tumors, hematologic malignancies, or as conditioning regimens before hematopoietic stem cell transplantation and randomized to receive either primary prophylactic G-CSF/GM-CSF or placebo/no treatment demonstrated an overall reduction in febrile neutropenia and documented infections, but no difference in infection-related or all-cause mortality.18 In this analysis, the median rate of febrile neutropenia in patients not receiving CSF support was 44.2%, highlighting the importance of chemotherapy-induced neutropenia. This study has also been one of the few meta-analyses to specifically examine the effect of CSFs in leukemia, reporting a reduction in febrile neutropenia and documented infection in this subgroup of patients in which individual studies have not always shown benefit.19 Meta-analyses and systematic reviews of CSF use for primary prophylaxis in leukemia have been hampered by significant heterogeneity among included studies but have shown (1) reduced infection in children with acute lymphoblastic leukemia (ALL) but no survival benefit and (2) a reduction in the duration of neutropenia and hospitalization but no reduction in documented infections (including bacteremias and invasive fungal infections) or mortality in adults with acute myelogenous leukemia (AML).20,21,22 A large multicenter study reported fewer sterile site bacterial and Clostridium difficile infections in children receiving G-CSF for primary prophylaxis in AML; however, mortality was not addressed.23 Finally, in a meta-analysis of primary prophylactic G-CSF/GM-CSF before neutrophil engraftment during autologous or allogeneic hematopoietic stem cell transplantation, CSF use was associated with a reduction in documented infection but not infection-related mortality.24 No increase in acute or chronic graft-versus-host disease was observed, although an increased incidence of both has been associated with G-CSF use in individual retrospective studies, without impact on overall survival.25
The available evidence therefore suggests that the use of CSFs for primary prevention in patients undergoing chemotherapy for solid tumors, hematologic malignancies, or as conditioning therapy before hematopoietic stem cell transplantation can reduce the incidence of febrile neutropenia and proven infections and may, in certain patient subgroups, reduce infection-related and all-cause mortality. An expert panel from the European Organisation for Research and Treatment of Cancer (EORTC) has recommended that the use of G-CSF as primary prophylaxis be reserved for adult patients with solid tumors or lymphoproliferative disorders receiving chemotherapy regimens associated with a 20% or greater risk of febrile neutropenia or for patients receiving chemotherapy regimens associated with a 10% to 20% risk of febrile neutropenia who have additional risk factors that predispose to febrile neutropenia, including previous episodes of the same.15 Although the most effective and appropriate use of G-CSF in patients undergoing autologous or allogeneic hematopoietic stem cell transplantation is the subject of ongoing debate, the most recent clinical practice guidelines from the European Society for Medical Oncology (ESMO) support G-CSF use following bone marrow transplantation, but not peripheral blood stem cell transplantation.26 There is insufficient evidence to declare the superiority of a CSF versus antibiotic strategy in primary prophylaxis of febrile neutropenia or to recommend that the two strategies be combined.27,28
Secondary prophylaxis refers to the use of a CSF to prevent neutropenia in patients who developed a neutropenic complication, typically an episode of febrile neutropenia, during a previous cycle of chemotherapy. Because the risk of a subsequent episode of febrile neutropenia may be 50% or more without colony-stimulating factor support, and because each episode of febrile neutropenia is associated with significant mortality, it is not surprising that secondary CSF prophylaxis reduces episodes of febrile neutropenia and hospitalization in a mixed population of patients with solid and liquid tumors and reduces mortality in older patients with non-Hodgkin’s lymphoma.29,30 The 2010 EORTC guidelines therefore recommend evaluation for secondary prophylaxis with G-CSF during all subsequent cycles of chemotherapy.15
G-CSF is also often used with antibiotics for treatment (as opposed to primary or secondary prevention) of febrile neutropenia. Several randomized trials and meta-analyses have been undertaken to clarify the added benefit of G-CSF in this situation, and the results have been surprisingly consistent: G-CSF, when administered as therapy for an established episode of febrile neutropenia, shortens the duration of both neutropenia and hospitalization but does not reduce overall mortality.31,32,33 Given the lack of demonstrable improvement in outcome, guidelines from EORTC suggest that G-CSF be reserved for those with life-threatening infections or who are not responding to appropriate antimicrobial therapy.15
Finally, it is important to address the emerging literature on differences in efficacy among the various G-CSF preparations, once thought to be clinically interchangeable, in patients with cancer. Neutrophils stimulated with lenograstim in vivo best maintain their phenotype and function when studied ex vivo, while those stimulated with filgrastim demonstrated the greatest perturbation in function.34 Consistent with this finding, use of lenograstim, as compared with filgrastim, resulted in fewer febrile episodes after initial neutrophil reconstitution (ANC > 0.5 × 103/mm3) following high-dose cyclophosphamide-induced neutropenia before peripheral blood stem cell mobilization in patients with multiple myeloma.35 In other clinical settings, however, pegfilgrastim has demonstrated superiority. Meta-analysis of five randomized controlled trials comparing once-daily filgrastim to single-dose pegfilgrastim for the primary prevention of chemotherapy-induced neutropenia in a mixed population of patients with solid organ and hematologic malignancies revealed a reduction in episodes of febrile neutropenia with the use of pegfilgrastim.36 Primary prophylactic pegfilgrastim has also been associated with a lower rate of hospitalization, both overall and neutropenia related, than has either filgrastim or sargramostim (GM-CSF).37 This difference may arise from the dosing schedule: a single dose of pegfilgrastim can provide relatively long-lasting protection from neutropenia, whereas daily-dose regimens of filgrastim may be stopped early. In keeping with this hypothesis, patients receiving daily-dose filgrastim for fewer than 7 days had a higher risk of hospitalization than those receiving it for at least 7 days. The most recent EORTC guidelines recommend optimization of dose, duration, and time of initiation of therapy with any G-CSF preparation to best prevent febrile neutropenia but also acknowledge that pegfilgrastim may have some advantage in terms of convenience and duration of action.15
G-CSF has been used in other neutropenic patient populations, in whom its benefits have been less clear. In HIV-infected patients, mild-to-moderate neutropenia commonly occurs as a result of medications, opportunistic infections, and the direct impact of HIV on neutrophil production and function.38 Neutropenia has been associated with an increased risk of bacterial infections and an increase in mortality in the setting of culture-confirmed bloodstream infections.39 However, neutropenia has not been shown to increase overall mortality in HIV-infected patients, and G-CSF has not been rigorously studied for the prevention of neutropenia-associated infection in patients receiving the current standard of antiretroviral therapy (ART).40
In hepatitis C virus (HCV) infection, with or without HIV coinfection, interferon-induced neutropenia has not been associated with an increased risk of bacterial infection.41,42 Although some preliminary reports suggested that more patients can achieve a sustained virologic response (SVR) when full-dose interferon therapy is continued with G-CSF support, a systematic review that included 19 studies found only weak evidence for improved SVR in patients with G-CSF support versus interferon dose adjustment.43 A randomized trial published after the review and hampered by low enrollment and high withdrawal rates did not detect a difference in SVR between HIV/HCV coinfected patients with interferon-induced neutropenia treated with G-CSF and those treated with interferon dose reduction.44 As a result, routine use of G-CSF is not recommended in HCV-infected patients with interferon-induced neutropenia.45
G-CSF has also received interest as a potential therapeutic immunomodulator in a number of non-neutropenic disease states. Although the goal of G-CSF therapy in this patient population was to enhance the microbicidal activity of existing phagocytes, the clinical results have been largely negative. In non-neutropenic patients with severe community-acquired pneumonia, treatment with G-CSF in addition to standard antimicrobial therapy reduced neutrophil apoptosis and increased absolute neutrophil counts, expression of cell surface markers of neutrophil activation, and release of anti-inflammatory cytokines.46 However, a systematic review of six RCTs enrolling a total of 2018 patients with pneumonia found no reduction in 28-day mortality or length of stay in the intensive care unit.47
Similarly, G-CSF is of no proven benefit in non-neutropenic adults with sepsis. In critically ill postoperative patients, G-CSF produced enhanced oxidative burst activity and phagocytosis when neutrophils were studied ex vivo.48 However, no improved neutrophil function was detected after administration of G-CSF to patients with the systemic inflammatory response syndrome (SIRS), and no mortality benefit could be detected in studies of sepsis secondary to pneumonia, severe sepsis secondary to melioidosis, or undifferentiated septic shock.49–51,52 In a meta-analysis of 12 RCTs evaluating non-neutropenic patients with sepsis who were randomized to receive either G-CSF/GM-CSF or placebo, administration of a colony-stimulating factor increased the likelihood of resolution of infection but not in-hospital or 28-day survival.53
In patients with diabetic foot infections, a meta-analysis of five small RCTs, enrolling 167 patients in total, demonstrated that G-CSF did not hasten resolution of infection but did lead to fewer surgical interventions, including amputations.54 However, disease severity among the enrolled patients varied greatly, from mild cellulitis to limb-threatening osteomyelitis, and guidelines from the Infectious Diseases Society of America concluded that there was insufficient evidence to recommend the routine use of G-CSF in these patients.55
G-CSF is now an established method of mobilizing large numbers of CD34+ hematopoietic stem cells from bone marrow to peripheral blood (peripheral blood stem cells [PBSCs]) in healthy donors for use in hematopoietic stem cell transplantation (HSCT). The ability to obtain large numbers of neutrophils has rekindled interest in granulocyte (neutrophil) transfusions for the treatment of serious opportunistic bacterial and fungal infections in patients with neutropenia or inherited disorders of neutrophil function, including chronic granulomatous disease and leukocyte adhesion deficiency, in which G-CSF therapy alone is inadequate. A murine model of pulmonary aspergillosis during neutropenia demonstrated a significant survival benefit with daily granulocyte transfusions and provides the rationale for human studies.56 Recent evidence in both adults and children has largely been limited to small, single-center retrospective analyses without comparator groups. In such studies, granulocyte transfusions appear to be safe and well tolerated, with transfusion-associated fever and chills most commonly reported and severe adverse events such as acute lung injury and human leukocyte antigen (HLA) alloimmunization less frequent.57,58 One recent RCT enrolled 74 patients with febrile neutropenia and pulmonary or soft tissue infiltrates (largely fungal in origin) but did not detect a survival benefit of granulocyte transfusions at either 28 or 100 days.59 Notably, however, G-CSF was administered to both treatment and control arms, neutrophil reconstitution was equivalent between groups, and the study was hampered by logistical issues in the harvesting and administration of the granulocytes. The RING study (Safety and Effectiveness of Granulocyte Transfusions in Resolving Infection in People with Neutropenia; ClinicalTrials.gov identifier NCT00627393), a multicenter RCT of high-dose (≥ 4 × 1010 cells per collection) granulocyte transfusions with an expected completion date of June 2013 should provide key information on safety and efficacy.
Table 48-1 provides a complete list of approved indications for G-CSF and other recombinant cytokines in North America and Europe.
TABLE 48-1
North American and European Approved Uses of Cytokines to Prevent or Treat Infection
CYTOKINE | INDICATIONS |
G-CSF (filgrastim) | After myelosuppressive chemotherapy for nonmyeloid malignancies |
After induction or consolidation chemotherapy for AML | |
Myeloid reconstitution after HSCT | |
Severe chronic neutropenia | |
Neutropenia in advanced HIV disease* | |
Pegylated G-CSF | After myelosuppressive chemotherapy for nonmyeloid malignancies |
GM-CSF (sargramostim)† | After induction chemotherapy for AML in patients >55 yr |
After transplantation of autologous PBSC | |
Myeloid reconstitution after autologous HSCT for Hodgkin’s lymphoma, non-Hodgkin’s lymphoma or ALL | |
Following allogeneic bone marrow transplantation from an HLA-matched related donor | |
HSCT failure or engraftment delay | |
IFN-α | Treatment of chronic HBV infection |
Treatment of chronic HCV infection | |
Treatment of condyloma acuminatum | |
Treatment of HIV-related Kaposi’s sarcoma | |
Pegylated IFN-α | Treatment of chronic HCV infection |
Treatment of chronic HBV infection | |
IFN-γ | Chronic granulomatous disease |
* Canada and Europe only.
† United States only.
ALL, acute lymphoblastic leukemia; AML, acute myelogenous leukemia; G-CSF, granulocyte colony-stimulating factor; GM-CSF, granulocyte-macrophage colony-stimulating factor; HBV, hepatitis B virus; HCV, hepatitis C virus; HIV, human immunodeficiency virus; HLA, human leukocyte antigen; HSCT, hematopoietic stem cell transplantation; IFN, interferon; PBSC, peripheral blood stem cell.
Adverse Effects
G-CSF is usually well tolerated. The most common adverse effect is mild-to-moderate bone and/or musculoskeletal pain, which is estimated to occur in at least 25% of recipients but can be controlled with nonsteroidal anti-inflammatory drugs (NSAIDs).1,9,60 Other commonly reported adverse effects include mild local erythema at injection sites, headache, anemia, splenomegaly, thrombocytopenia, and asymptomatic elevations of lactate dehydrogenase and alkaline phosphatase. As a result of G-CSF–induced upregulation of osteoclast activity, osteopenia or osteoporosis has been found in approximately one half of the patients who received long-term G-CSF therapy.11 More rarely, G-CSF has been associated with cutaneous neutrophilic vasculitis (Sweet’s syndrome), progression of autoimmune disorders, cancer chemotherapy–induced lung toxicity, severe pulmonary edema, and splenic rupture.9
In severe chronic neutropenia, there is a dose- and duration-dependent association between long-term G-CSF use and the risk of myelodysplastic syndrome (MDS) and AML.61 The overall cumulative incidence of MDS/AML after 15 years of G-CSF therapy was 22% and increased to 34% in patients who required higher-than-usual doses of G-CSF. However, a causal relationship has not been demonstrated. The risk of MDS/AML is consistent with that of other high-risk inherited bone marrow syndromes, and the risk appears to plateau after 10 years. Patients with cyclic and chronic idiopathic neutropenia are at lower risk of evolution to MDS/AML. Concern has also been raised about secondary MDS/AML in patients receiving G-CSF support during treatment for a primary malignancy, and caution has been advised for the use of G-CSF in children with ALL for this reason. In a systematic review of 25 RCTs enrolling patients with solid tumors or lymphoma, both the relative and absolute risks of MDS/AML with G-CSF use were statistically significant at 1.92% and 0.41%, respectively.62 Mortality, however, was significantly reduced in patients who had received G-CSF support, with relative and absolute risk reductions of 0.9% and 3.4%, respectively. There is currently no evidence that G-CSF is associated with hematologic malignancies in otherwise healthy individuals.
Granulocyte-Macrophage Colony-Stimulating Factor
GM-CSF is a 127-amino-acid glycoprotein that is available in various recombinant forms, including sargramostim (produced in yeast), molgramostim (produced in E. coli), and regramostim (produced in Chinese hamster ovary cells), which differ slightly in amino acid sequence and degree of glycosylation. The principal sources of endogenous GM-CSF are T lymphocytes, monocyte-macrophages, fibroblasts, and endothelial cells.1 GM-CSF raises peripheral blood counts and stimulates the function of neutrophils, monocytes, and eosinophils.1 Unlike G-CSF, GM-CSF does not play an essential role in neutrophil development: GM-CSF knockout mice have normal numbers of peripheral blood cells and bone marrow progenitors. However, GM-CSF knockout mice have impaired leukocyte function: they develop alveolar proteinosis as a result of impaired macrophage clearance of surfactant and have high rates of bacterial and fungal pneumonia.63,64
In the United States, GM-CSF is approved for human use only as sargramostim and has been employed primarily as an alternative to G-CSF to prevent neutropenia and its infectious risks in oncology patients after myelosuppressive chemotherapy.1 There are no biosimilar agents, and sargramostim is not available in Canada or Europe. In healthy volunteers, GM-CSF stimulates the release of mature neutrophils from the bone marrow, resulting in a rise in ANC to 3.5 times baseline levels.65 GM-CSF also enhances the induced neutrophil oxidative burst in vitro and results in eosinophilia in vivo.
Despite these findings, GM-CSF does not appear to be clinically equivalent to G-CSF for the prevention or treatment of neutropenia in patients with malignancies. After myelosuppressive chemotherapy, patients who received GM-CSF experienced a longer duration of neutropenia (14 vs. 12 days) than did those who received G-CSF.66 In a mixed population of patients undergoing chemotherapy for solid tumors or hematologic malignancies, GM-CSF reduced the incidence of febrile neutropenia and documented infections, but less so than G-CSF.18 Neither growth factor reduced all-cause or infection-related mortality. Compared with G-CSF, hospitalization rates for patients receiving GM-CSF for the prevention of neutropenia have been variously reported to be lower (infection-related), equivalent (febrile neutropenia-related), or higher (neutropenic complications including both fever and infection) depending on the outcome studied.31,37,67 In a meta-analysis of patients with lymphoma, G-CSF, but not GM-CSF, reduced the incidence of neutropenia and documented infection; however, few included studies used GM-CSF exclusively.17 In patients post-HSCT, the population in whom GM-CSF has been most widely studied, GM-CSF, but not G-CSF, reduced the incidence of documented infection. Once again, however, neither reduced all-cause or infection-related mortality.24 Prolonged use of GM-CSF after allogeneic HSCT appears to confer some antitumor activity (an effect related to GM-CSF–mediated increases in myeloid dendritic (antigen-presenting) cells and interleukin-12–stimulated cytotoxic T cells) and does not exacerbate graft-versus-host disease (GVHD).68,69 When used for treatment of an established episode of febrile neutropenia, GM-CSF, like G-CSF, does not reduce mortality.70 On balance, evidence does not support the use of GM-CSF in preference to G-CSF for either prophylaxis or treatment of chemotherapy-associated neutropenia. GM-CSF is not included in recent ESMO or EORTC guidelines and has limited approved indications in the United States (see Table 48-1).15,26
Nonetheless, like G-CSF, GM-CSF has been studied for use in non-neutropenic patients. GM-CSF–primed monocytes from patients with septic shock increased their oxidative burst activity in response to bacterial stimuli ex vivo, as compared with untreated cells from the same patients.71 In patients with the systemic inflammatory response syndrome but without hemodynamic instability, a 72-hour course of GM-CSF in addition to standard therapy resulted in multilineage leukocyte activation and a greater number of patients with clinical or microbiologic resolution of infection.72 However, GM-CSF had no impact on organ failure or 28-day mortality. Similarly, an RCT of a 5-day course of GM-CSF in 18 patients with sepsis and pulmonary dysfunction found no difference in the incidence of acute respiratory distress syndrome (ARDS), extrapulmonary organ failure, or 30-day mortality, despite increased superoxide production by peripheral blood neutrophils.73 An RCT of 38 patients with severe sepsis or septic shock and sepsis-induced immunosuppression as defined by monocytes with decreased HLA class II expression documented a shorter duration of mechanical ventilation and improved APACHE II scores with GM-CSF treatment.74 Once again, 28-day mortality was unchanged. Finally, when 132 critically ill patients with acute lung injury (ALI) or ARDS, most commonly due to sepsis and pneumonia, were randomized to receive either GM-CSF for 14 days or placebo, no difference in ventilator-free days or 28-day mortality was found between the groups.75 Given the small numbers of enrolled patients, all of these studies should be regarded as preliminary and hypothesis generating, and conclusions regarding the utility of GM-CSF in adults with sepsis should be withheld pending larger RCTs adequately powered to detect an impact on survival.
Similarly promising but inconclusive findings have emerged from studies of GM-CSF in sepsis in the pediatric population. A meta-analysis of treatment studies using G-CSF or GM-CSF in critically ill neonates found no survival benefit (14-day mortality) with the addition of CSF support to standard antimicrobial therapy, except in the small subgroup with severe neutropenia.76 In a relatively large RCT of 280 low-birthweight, preterm infants, a 5-day course of prophylactic GM-CSF started within 72 hours of birth did not reduce the incidence of sepsis or mortality (within 14 days or up to hospital discharge), even among the 21% of participants who were neutropenic.77 Finally, in a small study of 14 critically ill children with failure of at least three major organs and immunoparalysis (as defined by blunted tumor necrosis factor [TNF] production in response to lipopolysaccharide [LPS] ex vivo), patients who received GM-CSF had significantly fewer nosocomial infections than those who received the standard of care without CSF support.78
No definitive benefit to GM-CSF treatment has been found in the many other infectious diseases for which its use has been proposed. Preclinical studies suggested that GM-CSF might reverse HIV-associated defects in neutrophil recruitment and chemotaxis, but the results of clinical studies have been inconsistent.79,80 Based on ex vivo studies that demonstrated enhanced antifungal activity in human neutrophils, GM-CSF has been used, both alone and in combination with IFN-γ, as adjunctive therapy for refractory invasive fungal infections in neutropenic and non-neutropenic patients.81,82 Finally, a small RCT of 58 patients with nontraumatic generalized peritonitis who received GM-CSF for 4 days in addition to protocol-specified broad-spectrum antimicrobial therapy for at least 5 days reported fewer infectious complications in those receiving GM-CSF therapy.83
Adverse Effects
Compared with G-CSF, GM-CSF–associated toxicity in adults is more frequent and more severe, possibly because of stimulation of proinflammatory responses in monocytes/macrophages. Fever is the most common adverse effect, often accompanied by myalgias and malaise, and occurs in more than 20% of recipients.1 First-dose reactions, consisting of dyspnea, hypoxemia, hypotension, tachycardia, flushing, musculoskeletal pain, nausea, and vomiting, have been reported in 5% of patients receiving GM-CSF.84 Adverse effects are more common when GM-CSF is administered intravenously and with doses greater than 3 µg/kg. High doses (20 µg/kg/day) of GM-CSF have been reported to cause a generalized capillary leak syndrome.
Macrophage Colony-Stimulating Factor
M-CSF is a glycoprotein that was first cloned and produced in recombinant form in 1985. It is produced endogenously by monocyte-macrophages, fibroblasts, and endothelial cells. M-CSF acts specifically on cells of the monocyte-macrophage lineage to stimulate their production and enhance their functional activity.1 Clinical experience with M-CSF has been limited, and it is not currently approved for human use.
Interferons
IFNs are a class of cytokines distinguished by their intrinsic antiviral properties but produced in response to a variety of stimuli, including intracellular pathogens and bacterial toxins. IFNs play complex, pivotal roles in the host immune response, often augmenting the effects of other cytokines. Via JAK-STAT and other intracellular signaling pathways, IFNs act on T cells, natural killer (NK) cells, monocytes, macrophages, and dendritic cells to regulate apoptotic and antiapoptotic genes, influence cell-surface receptor expression, and activate virus-specific genes.85
There are three main classes of IFNs with clinical applications: IFN-α and IFN-β (type I IFNs) are produced primarily by leukocytes and fibroblasts, respectively; IFN-γ (type II IFN) is produced by NK cells and T lymphocytes. Whereas IFN-α and IFN-β are primarily antiviral interferons, IFN-γ activates macrophages and contributes to host defense against intracellular pathogens.
Interferon-α
The antiviral activity of IFN-α is discussed in Chapter 4. The term IFN-α actually refers to a class of molecules, of which there are 12 subtypes. Currently, IFN-α is available for clinical use as recombinant interferon-alfa-2a and interferon-alfa-2b, which differ by a single amino acid; interferon-alfa-n3, which is a mixture of subtypes of IFN-α purified from human leukocytes; and interferon-alfacon-1, a bioengineered form of IFN-α based on a “consensus” amino acid sequence of the most common forms of naturally occurring IFN-α. Pegylated forms of interferon-alfa-2a and interferon-alfa-2b have extended biologic half-lives.
IFN-α is most widely used as treatment for chronic hepatitis B and C virus infections (see Chapters 119, 148, and 156); however, it is also an effective therapy for various manifestations of human papillomavirus infection, including condyloma acuminata (genital warts) and recurrent respiratory papillomatosis.86,87
As an immunomodulatory agent, IFN-α has been studied as an adjunct to conventional antimicrobial agents. A combination of topical trifluorothymidine and IFN-α was reported to be effective in three HIV-infected individuals with refractory cutaneous herpes simplex virus infection, and combined use of IFN-α with foscarnet was reportedly successful in treating a single case of severe acyclovir-resistant perianal herpes simplex virus infection in an individual with advanced HIV disease.88,89 In virologically suppressed patients with HIV on ART, fewer patients who received pegylated IFN-α-2a had virologic failure when ART was subsequently stopped for 12 weeks than did historical control subjects.90 In a phase II, multicenter RCT of 259 patients with HIV who were failing current ART therapy (based on a detectable and increasing viral load), pegylated IFN-α-2b administered subcutaneously and once weekly for 4 weeks resulted in a significant decrease in plasma HIV RNA levels (mean 0.53 log10 copies/mL) despite an unchanged ART regimen.91 CD4+ T cell counts were also unchanged, but 15% of patients discontinued IFN therapy due to adverse events. IFN-α appears to have few long-term adverse effects on chronic HIV infection, and pegylated IFNs, both alfa-2a and alfa-2b, are used in combination with ribavirin for the treatment of HCV in HIV-infected individuals.92 Finally, preliminary studies have suggested that IFN-α may have clinical benefit in human T-cell lymphotropic virus (HTLV)-1-associated myelopathy and pulmonary tuberculosis, the latter in combination with standard antimycobacterial therapy.93,94
The major adverse effects of systemic IFN-α therapy are dose dependent and influenza like, with fever, headache, myalgias, arthralgias, nausea, and anorexia common. These symptoms are usually alleviated by pretreatment with acetaminophen or nonsteroidal anti-inflammatory agents and generally decrease in severity over a period of several weeks. At higher doses, bone marrow suppression; neuropsychiatric effects (depression, paresthesias, or change in mental status most commonly); and elevated serum hepatic aminotransferase levels are observed. Rash and erythema at the injection site are also reported. Intralesional IFN-α can cause local discomfort, inflammation, and mild systemic effects.
Interferon-γ
Recombinant human IFN-γ is available as interferon-gamma-1b. In vivo, IFN-γ is produced by CD4+, CD8+, and NK cells and targets monocytes, macrophages, and neutrophils, as well as nonprofessional host defense cells such as fibroblasts, hepatocytes, astrocytes, microglia, and endothelial cells.1 IFN-γ plays a critical regulatory role in macrophage-mediated killing and granuloma formation in response to important intracellular pathogens, including Mycobacterium, Leishmania, Rickettsia, Legionella, and Chlamydia species.1 Mice with targeted disruption of either the IFN-γ or IFN-γ receptor genes have increased susceptibility to viruses, M. tuberculosis, Calmette-Guérin bacillus, and L. monocytogenes.95,96 Individuals with autoantibodies against IFN-γ develop an adult-onset immunodeficiency syndrome characterized by multiple opportunistic infections by organisms including Mycobacterium species (tuberculous and nontuberculous, slow and rapid growing), Salmonella species, Cryptococcus neoformans, and varicella-zoster virus.97
Recombinant IFN-γ is approved by the U.S. Food and Drug Administration (FDA) for the prophylactic treatment of individuals with chronic granulomatous disease (see Table 48-1). In an early clinical trial, IFN-γ–treated patients developed significantly fewer infections and required fewer days of hospitalization.98 An observational study of 76 patients with chronic granulomatous disease documented persistent efficacy for up to 9 years when IFN-γ was used in combination with prophylactic trimethoprim-sulfamethoxazole and itraconazole.99 However, long-term use of IFN-γ may not provide added benefit to standard antimicrobial prophylaxis and is often limited by discontinuation due to adverse events.100
Hyperimmunoglobulin E syndrome (hyper-IgE syndrome) is a primary (congenital) immunodeficiency syndrome most commonly caused by mutations in the gene encoding STAT3 and characterized by recurrent skin and pulmonary abscesses, pneumonia, eczema, eosinophilia, and elevated serum IgE levels.101 Lymphocytes of patients with hyper-IgE syndrome have a depressed response to IL-12, resulting in decreased IFN-γ production after challenge with bacterial antigens.102 On the basis of these observations, a small, uncontrolled trial was performed, and it suggested possible improvement in the clinical course of hyper-IgE patients when treated with systemic IFN-γ therapy.103
IFN-γ has also been administered as adjunctive therapy for infections caused by intracellular pathogens in patients without immunodeficiency. Administration of IFN-γ with pentavalent antimony in visceral leishmaniasis was reported to significantly improve the response rate compared with historical control groups treated with pentavalent antimony alone.104 However, a prospective RCT of a 30-day course of pentavalent antimony, with or without adjunctive IFN-γ, failed to demonstrate any treatment benefit for the combination therapy.105 Adjunctive IFN-γ has also been reported to be effective for treatment of cutaneous leishmaniasis when combined with pentavalent antimony.106 When administered in lepromatous leprosy, IFN-γ has been shown to increase the macrophage response to Mycobacterium leprae and to enhance clearance of mycobacteria from skin.107 However, a small RCT involving 21 patients with multibacillary leprosy failed to show a significant beneficial effect on clinical or microbiologic outcomes when IFN-γ was added to standard multidrug therapy.108
When administered as adjunctive therapy to HIV-infected patients with disseminated Mycobacterium avium complex infection, systemic IFN-γ therapy decreased mycobacterial load.109 A study of HIV-negative patients with atypical mycobacterial infection of the lungs reported significant clinical improvement, persisting for 12 months after completion of therapy, when a 5-month course of IFN-γ was combined with standard antimycobacterial therapy.110 An aerosolized form of IFN-γ has been used successfully in patients with antimicrobial-refractory atypical mycobacterial infection of the lungs and in patients with pulmonary tuberculosis.111,112 In a meta-analysis of studies of adjunctive IFN-γ for the treatment of tuberculosis, the aerosolized form was associated with significantly higher rates of sputum clearance both at 1 month and at the end of treatment as compared with standard therapy alone.113 Chest radiograph improvement was also more likely in the IFN-γ-treated group. None of the included studies were of high methodologic quality, however.
Many recent studies have focused on the potential utility of IFN-γ for the treatment of invasive fungal infections. An RCT of two doses of adjunctive IFN-γ for the treatment of HIV-associated cryptococcal meningitis demonstrated significantly faster fungal clearance of the CSF with IFN-γ therapy versus amphotericin and 5FC alone.114 There was no difference in mortality or adverse events between the groups. Published case reports document the successful use of IFN-γ, in combination with G-CSF, GM-CSF, or granulocyte transfusions to treat or stabilize refractory invasive fungal infections in patients with leukemia or persistent and prolonged neutropenia.115,116 IFN-γ was also reported to be safe and well tolerated when used in combination with standard antifungal therapy to successfully treat disseminated fungal infections in seven renal transplant patients.117
Adverse effects of systemic IFN-γ administration include fever, myalgias, and headache, which occur in 15% to 50% of treated individuals. Although IFN-γ–associated influenza-like symptoms are typically mild, decrease over time, and can usually be managed with prophylactic antipyretics, these adverse effects are largely avoided if IFN-γ is administered via inhalation.1 Although reversible neutropenia can occur, clinically significant hematologic abnormalities are infrequent, even in patients who have received IFN-γ therapy for years.1 IFN-γ may exacerbate multiple sclerosis; therefore, caution should be exercised when using systemic IFN-γ therapy in patients with noninfectious inflammatory diseases. In addition, concern persists regarding the possibility of exacerbating GVHD if IFN-γ is administered to allogeneic HSCT recipients. Further study is necessary to document the safety of IFN-γ in this particular patient population.
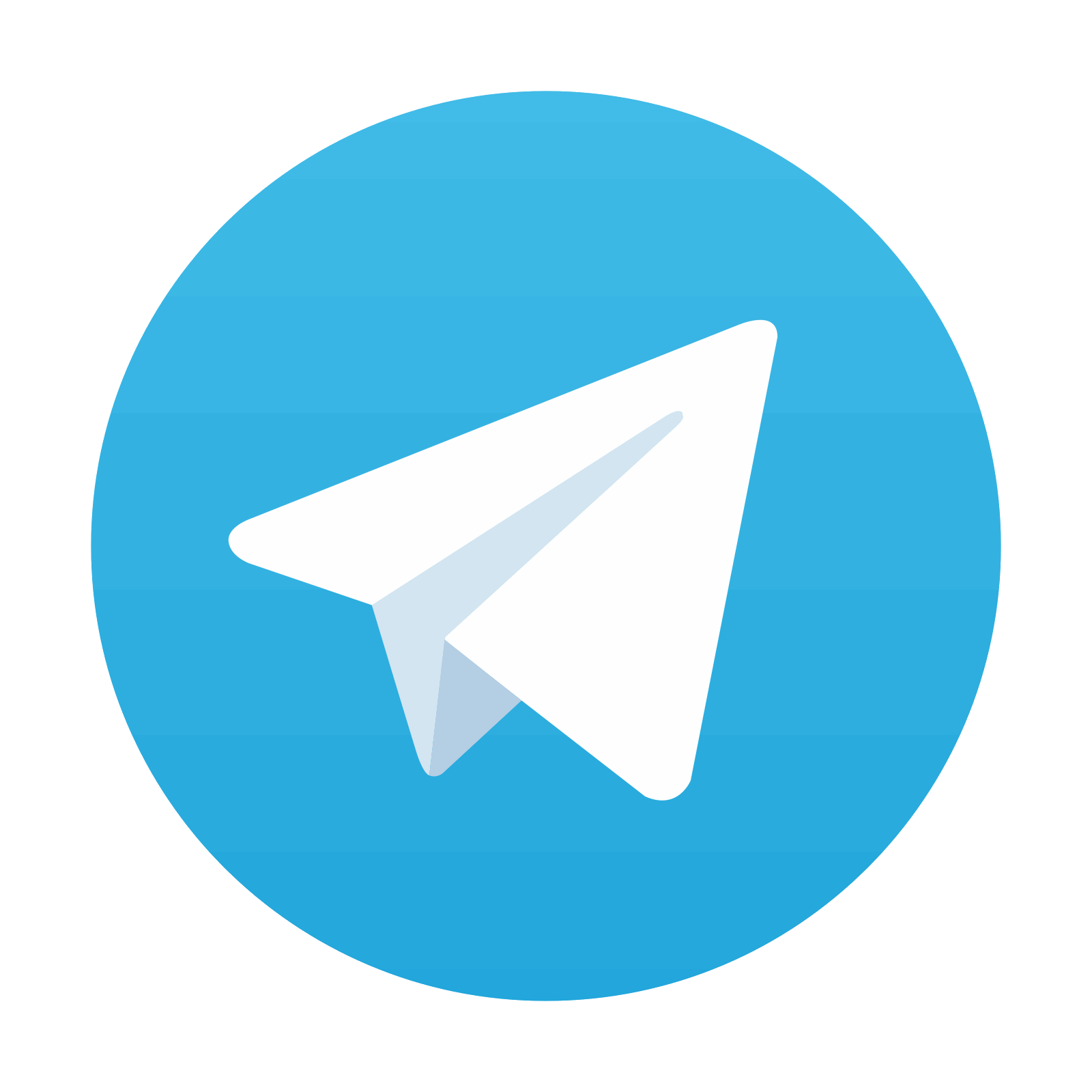
Stay updated, free articles. Join our Telegram channel

Full access? Get Clinical Tree
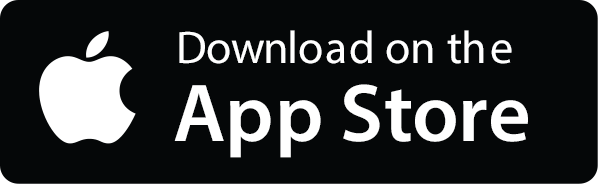
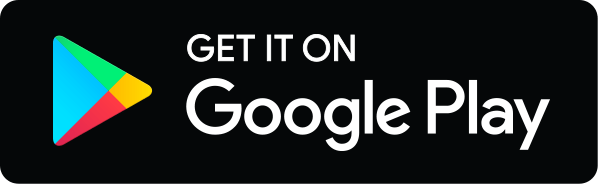