Abstract
Pheochromocytomas are neural crest-derived tumors of the adrenal medulla; paragangliomas are extra-adrenal pheochromocytomas. An accurate family and medical history is required to determine the risk for an inherited syndrome. All patients with a pheochromocytoma or paraganglioma should be offered genetic counseling. The qualifications for genetic testing are depending on age at diagnosis, tumor location, biochemistries, malignant behavior, and family history, in order to balance mutation detection rate and cost of testing.
Keywords
pheochromocytomas, paragangliomas, MEN2, medullary thyroid carcinoma (MTC), rearranged during transfection, Von Hippel–Lindau syndrome (VHL), neurofibromatosis type 1 (NF1)
Pheochromocytomas are rare neural crest-derived tumors of the adrenal medulla. By definition, a pheochromocytoma results in hypersecretion of catecholamines, which can cause headache, palpitations, elevated blood pressure (hypertension), and excessive perspiration, as well as other nonspecific symptoms. Pheochromocytomas have an incidence of approximately one in 100,000–300,000 in the general population. Although most pheochromocytomas are benign, they can cause major morbidity and death due to uncontrolled hypertension precipitated by stressful events such as anesthesia or pregnancy.
Extra-adrenal pheochromocytomas are referred to as paragangliomas. They are histologically identical, but occur outside of the adrenal medulla, anywhere in the sympathetic or parasympathetic paraganglia. Paragangliomas arising from the sympathetic paraganglia commonly hypersecrete catecholamines and are located mainly in the chest, abdomen, and pelvis. Parasympathetic paragangliomas are typically located within the head and neck (particularly the carotid body) and usually do not secrete excess catecholamines. Therefore, parasympathetic paragangliomas, if symptomatic, may be associated with a mass effect causing a visible or palpable neck mass, headaches, vocal cord disturbance, or cranial nerve deficit such as tongue weakness, shoulder drop, hearing loss, tinnitus, or problems with balance. The terms pheochromocytoma and paraganglioma are often used interchangeably in the medical literature; however, genetic risk assessment is different for pheochromocytoma and paraganglioma. In addition, terms such as ectopic pheochromocytoma, chemodectoma, nonchromaffin tumor, and glomus tumors are being phased out as these are anatomically nonspecific. The preferred terminology, which is used in this chapter, is pheochromocytoma for intra-adrenal tumors, and paraganglioma for extra-adrenal tumors, in addition to specifying the anatomic location (e.g., carotid body paraganglioma).
Pheochromocytomas have historically been referred to as “the 10% tumor,” given previous estimates that the rates of extra-adrenal location, malignancy, and heredity were each 10%. However, recent evidence suggests that the rate of an underlying hereditary condition may be closer to 30–40% if one includes patients with a familial presentation as well as those with an occult germline mutation with an apparently sporadic (nonfamilial) presentation, which may account for up to half of all hereditary cases. Therefore, lack of family history should not preclude referral for genetic assessment. The hereditary causes of pheochromocytoma and paraganglioma include multiple endocrine neoplasia types 2A and 2B (MEN2A/2B), von Hippel–Lindau syndrome (VHL), the familial paraganglioma/pheochromocytoma syndromes (SDHA, SDHB, SDHC, SDHD, SDHAF2, TMEM127, MAX), and neurofibromatosis type 1 (NF1). Pheochromocytoma has rarely been reported in patients with multiple endocrine neoplasia type 1 (MEN1). In addition, a proportion of familial cases of pheochromocytoma and paraganglioma have no known underlying genetic basis, suggesting the existence of additional susceptibility loci and/or limitations in current molecular genetic testing techniques. Currently, family history is the strongest independent predictor of a germline mutation in individuals with pheochromocytoma or paraganglioma; approximately 90% with a family history have an identifiable gene mutation.
Multiple endocrine neoplasia type 2
Multiple endocrine neoplasia type 2 (MEN2) is characterized by a very high lifetime risk of developing medullary thyroid carcinoma (MTC), given a penetrance of >95% in untreated patients. Pheochromocytoma is associated with two of the clinical subtypes of hereditary MTC, MEN2A, and MEN2B. Familial MTC (FMTC) is now thought to be a phenotypic variant of MEN2A rather than a separate subtype. The genotype-phenotype association of RET gene mutations has increasingly been recognized as the main stimulus of penetrance disease estimates. It is important to recognize that patients previously classified as having FMTC may still have a risk for developing additional endocrinopathies. In addition to MTC and pheochromocytoma, MEN2A is also associated with risk for primary hyperparathyroidism, and rarely individuals can develop a pruritic skin lesion (cutaneous lichen amyloidosis) or Hirschsprung disease. MEN2B is associated with a distinct physical appearance, which includes enlarged lips, a “bumpy” tongue, and eversion of the eyelids resulting from mucosal neuromas, as well as long, flexible limbs and joints, often referred to as a “Marfanoid” body habitus. The prevalence of MEN2 has been estimated at one in 35,000 individuals.
MEN2 is inherited in an autosomal dominant manner, meaning that those affected with MEN2 have a 50% chance of passing the condition onto their children with each pregnancy, with males and females having an equally likely chance to be affected. Most cases of MEN2A are inherited (approximately 10% of patients are the first affected person in their family, i.e., de novo ), whereas the majority of MEN2B patients do not have an affected parent and represent spontaneous or de novo mutations.
A definitive diagnosis of MEN2 in cases of apparently sporadic MTC or pheochromocytoma and in patients with an equivocal family history usually depends on the identification of a germline mutation in the rearranged during transfection ( RET ) proto-oncogene. RET is a 21-exon proto-oncogene located on chromosome 10q11.2 and encodes a receptor tyrosine kinase. The RET receptor interacts with the glial-derived neurotrophic factor family of ligands. Ligand binding induces receptor dimerization, autophosphorylation of intracellular tyrosine kinase residues, and activation of signaling cascades that ultimately lead to promotion of cell growth and survival. Virtually all MEN2-associated RET mutations are missense mutations, which cause constitutive activation of the RET receptor leading to unregulated cell growth and survival. MEN2-associated mutations are almost always located in exons 10, 11, or 13–16 of the RET proto-oncogene, although mutations in exons 5 and 8 have been reported on rare occasions. Strong genotype-phenotype correlations exist such that the codon in which the mutation occurs can be used to predict MEN2 subtype, risk for pheochromocytoma and hyperparathyroidism, and age at onset and aggressiveness of MTC. Mutations in the extracellular, ligand-binding domain (exons 5 and 8) and the first intracellular tyrosine kinase domain (exons 13 and 14) are mainly associated with low risk for pheochromocytoma and lower penetrant and less aggressive medullary thyroid cancer. Mutations in the extracellular cysteine-rich domain, which mediates dimerization (exons 10 and 11), are mainly associated with MEN2A and have a moderate aggressiveness of medullary thyroid cancer. Finally, the two specific mutations causing MEN2B, A883F, and M918T are located in the second intracellular tyrosine kinase domain and cause the earliest onset and most aggressive form of MTC ( Table 16.1 ).
Level | RET codon | Associated clinical subtypes | Relative risk for pheochromocytoma | Relative risk of hyperparathyroidism | Age of prophylactic thyroidectomy |
---|---|---|---|---|---|
MOD | 768 790 791 804 891 | Usually FMTC | Low | Low | Consider by age five, but may be delayed * |
609 611 630 | Usually FMTC | Low | Low | ||
618 620 | MEN2A or FMTC | Moderate | |||
H | 634 | MEN2A | High | High | By three to five years |
HST | 883 918 | MEN2B | High | No increased risk | <12 months |
* Thyroidectomy can be delayed as long as:
- •
Annual calcitonin level is not elevated
- •
Annual thyroid ultrasound is normal
- •
MTC is not aggressive in the family
- •
Patient preference
Pheochromocytomas occur in up to 50% of individuals with MEN2A and MEN2B. Pheochromocytomas occur most commonly with codon 634 (MEN2A) and codon 918 (MEN2B) RET proto-oncogene mutations, and with lesser frequency in kindreds with mutations of codons 532–534, 630, 777, and 912. Pheochromocytomas appear to be more commonly seen in association with the high-risk mutations for MTC.
Pheochromocytomas tend to develop after MTC is identified; however, there are well-documented examples of MEN2-related pheochromocytomas being responsible for the initial manifestations of this syndrome. Even though fewer than 3% of cases of apparently sporadic pheochromocytoma occurring before age 50 years are due to germline mutations of the RET proto-oncogene, RET testing of certain patients with apparently sporadic pheochromocytoma should still be done given the important clinical implications of making an accurate diagnosis.
The pattern of catecholamine production in MEN2 differs from that seen in other hereditary forms of pheochromocytoma. MEN2-related pheochromocytomas usually secrete epinephrine/metanephrines and may or may not secrete noradrenergic catecholamines. This biochemical phenotype results in an early clinical presentation characterized by attacks of palpitations, nervousness, anxiety, and headaches, rather than the more common pattern of hypertension seen with sporadic or other hereditary tumors. Some symptoms may be so subtle that the patient will be unaware of them and not bring any symptoms to medical attention. Bilateral pheochromocytomas occur in approximately half of patients with MEN2 with high risk RET mutations; their development is frequently asynchronous, with separation by as much as 15 years. Malignant pheochromocytomas and paragangliomas are extremely rare in MEN2. Malignant pheochromocytomas are generally associated with large infiltrating tumors. There have been reports of sympathetic paragangliomas in MEN2, although most of these have been found in the adrenal region and may actually represent a tumor that has developed in an adrenal rest, recurrence of a previously excised adrenal medullary tumor, or seeding from a malignant pheochromocytoma. Therefore, young patients presenting with apparently sporadic adrenergic pheochromocytoma should be offered testing for RET mutations, whereas patients with entirely noradrenergic pheochromocytomas, paragangliomas, or malignant tumors are unlikely to benefit from RET testing.
MEN2-associated pheochromocytomas are often detected by routine biochemical screening or present symptomatically due to palpitations, headache, tachycardia, or sweating. Plasma-free metanephrines have the highest sensitivity and specificity for detecting pheochromocytoma, particularly for those with hereditary syndromes, and are the preferred method of surveillance. However, measurement of 24-h urine catecholamines and metanephrines and serum catecholamines are also frequently used. Importantly, biochemical screening for pheochromocytoma should occur before any type of surgical intervention or pregnancy in patients with MEN2; the consequences of an undiagnosed pheochromocytoma in these situations can be life threatening. For MEN2 patients with biochemical evidence or convincing symptoms of pheochromocytoma, imaging studies such as CT or MRI are useful for determining whether one or both adrenal glands are involved. Patients with a pheochromocytoma should be treated with alpha- and sometimes beta-blockade before undergoing surgery. There is really no role for adrenal biopsy in patients with suspected pheochromocytoma, and biopsy carries the risk of adrenergic crisis.
The typical age at onset of MEN2-associated pheochromocytomas is in the third decade of life, which is approximately 10–20 years younger than the typical age of sporadic pheochromocytoma development. Surgery is the treatment modality of choice, and due to the high frequency of bilateral tumors, bilateral adrenal surgery is often required at some point. Bilateral total adrenalectomy renders the patient dependent on replacement doses of corticosteroid drugs for life and at risk for acute adrenal insufficiency (Addisonian crisis), and therefore a cortex-sparing adrenalectomy should be considered whenever technically possible in all patients. In situ preservation of the intact functional adrenal cortex, while removing most of the adrenal medulla and all of the associated pheochromocytoma (cortical-sparing adrenalectomy) would be the ideal operation. While associated with higher rates of recurrence (because it is impossible to remove all medullary tissue when preserving some cortex), this approach minimizes the risk of adrenal insufficiency, which is an appropriate balance with regard to risk for recurrence given the very low malignant potential for MEN2-related pheochromocytomas. In one of the largest studies of patients with hereditary pheochromocytomas, 96 patients underwent adrenalectomy, including 39 patients who underwent intentional cortical-sparing adrenalectomy. In these patients, only one (3%) patient developed acute adrenal insufficiency, two (7%) patients developed recurrent disease in the adrenal remnant, and 21 of 27 patients with ≥3 years of follow-up were steroid independent. Adrenalectomy, total or cortical-sparing, can be safely performed via the laparoscopic (transabdominal or retroperitoneoscopic) or open approach, ideally by high-volume adrenal surgeons, given the association between surgeon volume and patient outcomes. Patients who do undergo bilateral total adrenalectomy should wear medical alert bracelets indicating that hydrocortisone could be life-saving in emergency situations.
Because nearly all MEN2 patients eventually develop MTC, patients with a germline RET mutation are generally recommended to undergo early intervention for MTC. For the highest risk mutations, early detection of MTC is difficult and the treatment options for locally advanced and metastatic disease are limited. Given the acceptably low morbidity and mortality associated with thyroidectomy, early thyroidectomy has become widely accepted as standard of care. Guidelines are available to determine the earliest age at which prophylactic thyroidectomy would be appropriate in mutation carriers. Mutations associated with the least aggressive forms of MTC are “moderate risk” (MOD) mutations and occur in RET codons 609, 611, 618, 620, 630, 768, 790, 791, 804, or 891; these were previously described as Level A and B mutations. MTC associated with these codons is typically later onset, less aggressive with rare reports of MTC-related death, and may not be fully penetrant. However, there are rare cases of more aggressive MTC occurring with mutations in these codons. Genetic testing and initiation of MTC screening for RET mutation carriers is still recommended by three to five years of age; however, thyroidectomy can be delayed as long as: thyroid ultrasound and measurement of serum levels of calcitonin, performed on at least a yearly basis, remain without evidence of MTC; the MTC in other family members is not aggressive; and, perhaps most importantly, the patient prefers to delay surgery. “High risk” (H) mutations (codons 634 and 883) are associated with moderately aggressive MTC and thyroidectomy is recommended by age five years, based on serum calcitonin levels. The “highest risk” (HST) mutation is associated with the most aggressive form of MTC and includes the MEN2B-related mutations on codon 918. Individuals with an HST mutation should undergo prophylactic thyroidectomy by twelve months of age, with some experts advocating for even earlier surgery (Table 17.1). However, this rarely occurs, as newborns do not have the phenotypic appearance of MEN2B, and the mutation is spontaneous in almost all affected infants. These recommendations are based on consensus guidelines published by expert panels, and they have evolved over time and are likely to continue to evolve as additional data on long-term outcomes are collected.
Von Hippel–Lindau syndrome (VHL)
In addition to MEN2, VHL is a common cause of hereditary pheochromocytoma and should be one of the first syndromes to consider, particularly if the patient is very young (VHL accounts for nearly half of pheochromocytomas presenting before age 20 years), or if the tumor has noradrenergic catecholamine secretion. Overall, VHL accounts for approximately 11% of apparently sporadic pheochromocytomas. Most VHL-associated pheochromocytomas are benign and intra-adrenal, however (in contrast to MEN2), extra-adrenal paragangliomas and malignant tumors may occur beyond that of a truly anecdotal patient. VHL is also inherited in an autosomal dominant manner and has a prevalence of about one in 36,000 persons, with an estimated 20% of cases being de novo mutations. As such, a significant proportion of patients with VHL do not have a family history of disease.
In addition to pheochromocytoma, VHL is characterized by hemangioblastomas in the retina and central nervous system, renal cysts and renal cell carcinoma, pancreatic cysts and pancreatic endocrine tumors, endolymphatic sac tumors, and papillary cystadenomas of the epididymis and broad ligament. Some families with VHL have been reported to have developed only pheochromocytomas and/or paragangliomas; however, it is not clear whether these families were simply ascertained before the onset of other VHL-related manifestations. VHL is classified into two main types, based on the risk for pheochromocytoma; VHL type 1 is characterized by a low risk of pheochromocytoma, whereas VHL type 2 is characterized by a high risk of pheochromocytoma. VHL type 2 is further divided based on risk for renal cell carcinoma; type 2A is associated with a low risk of renal cell carcinoma, type 2B is associated with a high risk of renal cell carcinoma, and type 2C is defined as pheochromocytoma occurring without other manifestations of VHL disease ( Table 16.2 ). VHL is highly variable, both within and between different families, in terms of the age at onset, number and types of tumors, and severity of the disease. VHL is thought to be fully penetrant by age 60, although the most common age of onset is in the second to third decade of life. Like MEN2, VHL-associated pheochromocytomas are often detected on routine biochemical screening (preferably through measurement of plasma-free metanephrines and normetanephrines) or due to patient-reported symptoms, and they are often bilateral. Diagnosis and treatment of VHL-associated pheochromocytoma is similar to that of MEN2-associated pheochromocytomas; however, the possibility of extra-adrenal tumors (i.e., paragangliomas) and malignancy must be considered in patients with VHL.
VHL subtype | Associated tumor types | Most common gene mutation type | ||
---|---|---|---|---|
HB | RCC | PGL/PCC | ||
Type 1 | + | + | – | Deletions, truncations |
Type 2A | + | – | + | Missense (Y98H, Y112H) |
Type 2B | + | + | + | Missense |
Type 2C | – | – | + | Missense (L188V, V84L, S80L) |
VHL is caused by germline inactivating mutations of the VHL tumor suppressor gene, which is comprised of three exons and is located on chromosome 3p25. The most well-defined role of the VHL protein is in its participation in the regulation of the hypoxia-inducible factor (HIF) pathway. Normally, HIF is active in hypoxic states to promote oxygen-independent ATP synthesis and angiogenesis and its alpha subunit is degraded in normoxic states. VHL, in complex with elongins B and C, is involved in proteolytic degradation, ultimately resulting in reduced or abnormal function of the VHL protein, thus permitting increased HIF1 and HIF2 activity. VHL’s involvement in the HIF pathway likely contributes to the highly vascular nature of VHL-associated tumors. However, failure to properly degrade HIF does not seem to be an important mechanism in the development of VHL-associated pheochromocytomas.
Sequencing and large deletion/duplication testing detects nearly 100% of VHL-associated mutations, making genetic testing the most definitive and cost-effective method to diagnose or rule out VHL syndrome in patients presenting with VHL-related diseases such as pheochromocytoma. Rare cases of VHL with an undetectable mutation are documented and are likely due to mutations acquired during embryogenesis resulting in somatic mosaicism. Unlike MEN2, in which a few recurring mutations account for the majority of the disease, over 500 VHL gene mutations have been described, and there is considerable variation in the number, location, and types of mutations involved. Despite this, there are genotype-phenotype correlations in VHL and there are a limited number of recurring mutations that occur either in hypermutable sequences (delPhe76, N78S/h/T, P86L, R161Ter, C162Y/F/W, R167Q/W, and L178P) or as founder mutations, such as the Black Forest Y98H mutation in German families. The majority (96%) of VHL patients with pheochromocytoma (i.e., VHL type 2) have missense mutations, whereas 95% of null or truncating mutations do not cause VHL-associated pheochromocytoma (i.e., VHL type 1). Mutations at codon 167 are responsible for approximately 46% of VHL type 2 families. Four mutations, L188V, V84L, S80L, and F161Q, have been associated with the pheochromocytoma-only subtype (type 2C), and three mutations, L128F, S111C, and R161Q, have been associated with a risk of pheochromocytoma without renal cell carcinoma (i.e., type 2A) ( Table 16.2 ). Importantly, not all laboratories offer both sequencing and large deletion testing for the VHL gene. Large deletion testing is necessary to achieve a near 100% mutation detection rate.
If a diagnosis of VHL is confirmed, the patient should be referred for appropriate tumor surveillance studies. The recommended surveillance strategy varies depending on patient age and whether there are any symptomatic complaints, but typically involves: (1) ophthalmologic evaluation, at least yearly and biannually during puberty; (2) annual abdominal ultrasound of the kidneys starting around 10 years of age and switching to MRI abdominal surveillance around age 20; (3) baseline MRI of the brain and spine starting around puberty and repeating every one to three years; and (4) annual audiology exam to monitor hearing. This monitoring should be implemented in all VHL gene mutation carriers, even if they appear to have a pheochromocytoma-only phenotype. Surveillance for pheochromocytoma in type 2 families involves annual measurement of plasma-free metanephrines and normetanephrines. Families who appear to be type 1 based on family history and genetic test results may not need to undergo as rigorous screening for pheochromocytoma as type 2 families; however, screening should be performed prior to any surgical procedure or pregnancy/delivery.
Neurofibromatosis type 1
NF1 is also associated with hereditary pheochromocytoma; however, genetic testing is generally not necessary to establish a diagnosis of NF1. Patients with NF1 will have manifestations that are obvious on physical examination, most commonly including café au lait macules, neurofibromas, and axillary and inguinal freckling by the time they are at risk for the development of pheochromocytoma. The National Institutes of Health diagnostic criteria for NF1 include a patient with two or more of the following clinical features: (1) six or more café au lait macules measuring at least five mm in prepubertal individuals and at least 15 mm in postpubertal individuals; (2) two or more neurofibromas; (3) a plexiform neurofibroma; (4) axillary or inguinal freckling; (5) optic glioma; (6) more than one Lisch nodule; (7) sphenoid dysplasia or tibial pseudoarthrosis; (8) a parent, sibling, or child with NF1. By eight years of age, 97% of NF1 patients meet these criteria, and all do so by 20 years of age.
Pheochromocytomas rarely develop in NF1 (approximately 1% risk) and tend to behave in a similar manner as sporadic pheochromocytoma. The average age at onset is in the fourth decade, but they can occur in childhood. There are rare examples of multigenerational pheochromocytomas. Most pheochromocytomas in NF1 produce mainly norepinephrine and therefore most commonly present with hypertension and noradrenergic symptomatology. However, 22% of pheochromocytomas have no symptoms related to excessive catecholamine secretion. Approximately 11–12% of tumors are malignant, 10% are bilateral, and over 94% are located within the adrenal gland.
Hereditary paraganglioma/pheochromocytoma syndromes
Germline mutations in the succinate dehydrogenase complex genes (abbreviated SDHx ), along with TMEM127 and MAX genes, are known to cause hereditary paraganglioma-pheochromocytoma (PGL/PCC) syndromes. Hereditary PGL/PCC syndromes are characterized by susceptibility to multiple paragangliomas of the head and neck, thoracic, and abdominal paraganglia, as well as pheochromocytoma. Most patients with hereditary PGL/PCC and 8–50% of apparently sporadic, young onset paragangliomas are caused by a mutation in the SDHB , SDHD , or SDHC genes.
Of the SDHx genes, SDHB and SDHD are the most common genes responsible for hereditary PGL/PCC syndromes, followed by SDHC , whereas SDHA and SDHAF2 gene mutations have only been observed in a handful of families. The typical age at tumor development in patients with SDHx -related hereditary PGL/PCC syndrome is in the late 20s to early 30s; however, a wide range of ages of onset have been reported and penetrance can be incomplete, particularly for SDHB and SDHC mutation carriers. Each of the SDHx genes is distinguished by the most common location of tumor development, risk for malignancy and the inheritance pattern. The SDHB and SDHD genes were the first to be discovered, are much more commonly mutated than the others, and therefore are the most well characterized of all the SDHx deficiencies.
In SDHB gene mutation carriers, paragangliomas develop most frequently in the abdomen, followed by head and neck locations, and are less common in the chest or adrenal gland. SDHB -related paragangliomas are also associated with a high rate of malignancy reported to approach 38%. The risk for malignancy appears to be highest in those patients with extra-adrenal abdominal tumors (notice the consistent theme here regardless of the underlying disease), risk for malignant biologic behavior (distant metastases) of head/neck paragangliomas or pheochromocytoma is unknown, but overall, uncommon. Malignant behavior may be evident at the time of diagnosis, or may not be apparent for months and sometimes years after initial diagnosis. Similar to sporadic paragangliomas, SDHB -associated paragangliomas that develop in abdominal locations tend to secrete catecholamines, whereas those that develop in the head and neck are usually nonfunctioning. Patients present with either the characteristic symptoms associated with catecholamine excess such as hypertension, headache, excessive perspiration, and palpitations, or they may be asymptomatic due to a nonfunctional tumor. An important management consideration for SDHB carriers is that approximately 10% of abdominal/pelvic tumors are biochemically nonfunctional and only cause symptoms related to mass effect, such as abdominal or back pain, abdominal distention, urinary abnormalities, deep vein thrombosis, or weight loss. The lack of symptoms of catecholamine excess is likely why these SDHB -associated tumors tend to be larger than sporadic tumors. The majority of functional tumors secrete noraderenergic catecholamines and frequently secrete dopamine. Initial estimates of the age-related penetrance of tumor development in SDHB mutation carriers was 30–50% by age 30–35 years, 45% by age 40 years, and 77% by age 50 years. However, these estimates were largely based on affected individuals from high risk families initially ascertained for gene discovery studies and may overestimate the true frequency of tumor development due to selection bias. More recently, studies conducted on mutation carriers identified through predictive genetic testing have estimated the lifetime risk to be approximately 30%, which seems like a more accurate estimate based on clinical experience with these families. The earliest reported diagnosis of a paraganglioma in an SDHB mutation carrier was age 10 years. Many (approximately 72%) SDHB mutation carriers develop only a single tumor and the majority have an apparently sporadic presentation, although the percentage of patients with a de novo mutation has not yet been well established. SDHB mutations are inherited in an autosomal dominant manner.
Germline SDHB mutations have been found to be an independent predictor of poor prognosis in individuals with a malignant pheochromocytoma or paraganglioma. SDHB mutation carriers have a significantly lower five-year survival rate and a shorter metanephrine excretion doubling time than patients with malignant sporadic tumors. In fact, an SDHB germline mutation is the only independent predictor of malignancy in pheochromocytomas and paragangliomas at this time. The high rate of malignancy in SDHB mutation carriers warrants close and aggressive tumor surveillance and treatment. At present, evidence-based guidelines on the diagnosis, surveillance, and management of SDHB -associated tumors are not available. Based on available natural history data, it is reasonable to recommend annual plasma or urinary fractionated metanephrine measurement in addition to cross-sectional imaging studies every 1–2 years in all mutation carriers. In asymptomatic patients, MRI is the preferred screening method due to the minimal radiation exposure associated with it. We favor MRI (as a screening study) for any patient at risk for an abdominal tumor/recurrence when it is anticipated that they will require more than 10 imaging studies and have an expected survival greater than 10 years. Whole body rapid MRI protocols have been developed at some centers, appear to be a promising screening modality, and are less expensive than ordering MRI of each system separately. If a tumor is suspected based on screening studies or symptoms, further diagnostic imaging studies such as targeted CT or MRI can be utilized and have a high sensitivity for detecting pheochromocytoma and paraganglioma. In general, if the tumor is large enough to cause symptoms, it will be seen on cross-sectional imaging. Functional imaging modalities such as 123 I-labeled meta-iodobenzylguanide scintigraphy, which has a lower sensitivity but higher specificity, or studies such as 6-[ 18 F]-fluorodopamine, [ 18 F]-dihydroxyphenylalanine, [ 11 C]-hydroxyephedrine, or [ 11 C]-epinephrine PET scans may also be helpful. The preferred imaging modality may be influenced by genotype; one recent study determined that [ 18 F]-FDG-PET had a sensitivity of almost 100% for the detection of SDHB -related paraganglioma metastases and may also be useful for preoperative staging as well as surveillance after tumor resection. However, functional imaging is not recommended as a routine surveillance test in asymptomatic patients.
Sequencing of the coding region (exons 1–8) and intron-exon junctions as well as large deletion/rearrangement analysis are clinically available for SDHB . The detection rate using these testing methodologies is currently unknown. Large deletions are now believed to occur in a significant proportion of cases. For example, one study found three (12.5%) SDHB deletions in 24 patients who had either familial, multifocal, extra-adrenal, or young age of onset (age 35 years and younger) disease and who had previously tested negative for point mutations in RET , VHL , SDHB , SDHD , and SDHC . Therefore, we advocate that large deletion/duplication testing be utilized in all patients who undergo SDHB testing.
Paragangliomas in SDHD gene mutation carriers tend to develop most often in the head and neck; however, abdominal and thoracic paragangliomas and adrenal pheochromocytomas are also observed at a lower frequency. The vast majority of paragangliomas in SDHD mutation carriers demonstrate benign biologic behavior, although some can be locally invasive and rarely can metastasize. The age-related penetrance of tumors in carriers of a paternally inherited SDHD mutation is estimated at 50% by age 31 years and 86% by age 50 years. Most (approximately 75%) have multifocal tumors such as bilateral carotid body tumors. The earliest reported age of paraganglioma development in an SDHD mutation carrier was 5 years.
SDHD mutations are inherited in an autosomal dominant pattern with parent of origin effects. While any person with an SDHD mutation is at 50% risk of passing the mutation on to each of their children, the gender of the transmitting parent determines whether the child is at risk for tumor development. Individuals who inherit an SDHD gene mutation from their father are at high risk for paraganglioma development; those that inherit a mutation from their mother are not at risk for paraganglioma development. While there are reports of finding a paraganglioma in patients who inherited an SDHD mutation from their mother, these appear to be exceptional, and do not justify routine screening for patients with a maternally transmitted SDHD mutation. The rate of de novo mutations has not yet been established.
There are no consensus guidelines for surveillance of SDHD mutation carriers. As the majority of tumors are nonfunctional and develop primarily in head and neck locations, cross-sectional imaging studies will usually be necessary for tumor detection. However, because functioning abdominal pheochromocytoma and paraganglioma can also occur, annual biochemical screening using urinary or plasma fractionated metanephrine levels is also recommended. Whether abdominal imaging in the absence of biochemical evidence of a functioning pheochromocytoma or paraganglioma is warranted is unclear; however, the utilization of serial whole body MRI for screening asymptomatic patients should be considered in these patients as well.
Most SDHC mutation carriers present with benign tumors of the head and neck, although abdominal, thoracic, intra-adrenal, and malignant tumors have been observed on multiple occasions. In one large published cohort of unselected patients diagnosed with pheochromocytoma/paragangliomas, SDHC mutations were found in 1.85% of index cases, which comprised 8.3% of all the mutations identified. SDHC mutations are inherited in an autosomal dominant manner and appear to be incompletely penetrant, though precise lifetime risk estimates have not yet been established. The rate of de novo mutations is currently unknown.
SDHB, SDHC, SDHD , and SDHA encode proteins that comprise the four subunits that make up the succinate dehydrogenase complex, an enzyme involved in the primary mechanisms of ATP synthesis within the mitochondria, the electron transport chain, and the Krebs cycle. Succinate dehydrogenase is responsible for oxidizing succinate to fumerate within the Krebs cycle. The SDHC and SDHD subunits are responsible for anchoring the succinate dehydrogenase enzyme to the mitochondrial membrane, while SDHB and SDHA form the enzyme’s catalytic core. Germline mutations of SDHB , SDHD , SDHC , or SDHA are thought to result in disassembly of the succinate dehydrogenase enzyme, causing reduced enzymatic function leading to accumulation of succinate and oxaloglutarate within the cell. In addition to being key intermediaries of the Krebs cycle, succinate and oxaloglutarate are also cofactors of the polyhydroxylase enzymes involved in HIF degradation. Therefore, the downstream effect of abnormal SDHB, SDHD, SDHC, or SDHA subunit function is an increase in available HIF, thus leading to a similar mechanism of tumorigenesis as is seen in VHL.
While SDHA has long been known for its role in Leigh syndrome, a severe neurodegenerative disorder caused by biallelic germline SDHA mutations, heterozygous SDHA mutations have only recently been implicated in the development of pheochromocytoma/paragangliomas. To date, only a handful of patients have been described; and given that pheochromocytomas and paragangliomas have not been a reported feature in family members of patients with Leigh syndrome, heterozygous SDHA mutations appear to confer low-penetrant susceptibility.
The SDHAF2 gene (also known as SDH5 ) was implicated in hereditary PGL/PCC syndromes in 2009. Mutations in this gene have only been identified in two families, which have exclusively developed benign head/neck paragangliomas. The SDHAF2 gene produces a protein that facilitates posttranslational modification of the SDHA protein.
Shortly after the discovery of the SDHAF2 gene, mutations in TMEM127 were identified in a cohort of sporadic and autosomal dominantly inherited pheochromocytomas. Subsequently, additional studies have identified TMEM127 mutations in extra-adrenal paragangliomas, bilateral pheochromocytomas, and rarely in patients with malignant pheochromocytomas or paragangliomas. The gene is thought to be a negative regulator of mTOR, with a kinase receptor signaling transcription signature similar to that of NF1 and RET gene mutations. This is in contrast to the expression profiles seen in VHL or SDHx gene mutations, which are characteristic of hypoxia response. Most recently, whole-exome sequencing (WES) identified mutations in the MAX gene in individuals with pheochromocytoma, including those with bilateral and malignant disease. The MAX (MYC-associated factor X) gene encodes a protein involved in the regulation of cell proliferation, differentiation, and apoptosis. TMEM127 and MAX mutations appear to be quite rare.
The utility of WES was once again proven successful in the recent identification of mutations in the FH gene predisposing to pheochromocytomas and paragangliomas. Mutations in FH are associated with hereditary leiomyomatosis and renal cell cancer, but no associations with pheochromocytomas or paragangliomas were previously appreciated. FH mutations have been identified in patients with malignant pheochromocytomas or paragangliomas. The authors proposed, based on immunohistochemistry staining patterns in both the SDHB-deficient tumors and FH-deficient tumors, that the mechanism for tumorigenesis involved a hypermethylation phenotype leading to genome-wide alterations in DNA methylation.
FH , TMEM127 , and SDHA mutations are inherited in a classic autosomal dominant pattern. All of the families reported to date with mutations in SDHAF2 and MAX have exhibited an inheritance pattern consistent with maternal imprinting (e.g., similar to SDHD , tumors develop only in carriers of a paternally inherited mutation). However, phenotype characterization of additional families with mutations in these genes is required before making any definitive conclusions. Importantly, further research on large population-based cohorts needs to be performed before penetrance estimates, malignant potential, and multifocality can be accurately predicted in carriers of these newly described gene mutations. Studies of TMEM127 , MAX , and FH mutations in unselected patients with pheochromocytomas/paragangliomas suggest a mutation frequency in the range of 1% for each gene. Newly emerging associations with the KIF1B , EGLN1 , and HIF2A genes are currently being investigated.
It is now well established that there is a small but real increased risk for gastrointestinal stromal tumors (GISTs) in patients with SDHx mutations, and renal carcinoma with SDHB mutations. Germline SDHB , SDHC , and SDHD mutations have been identified in families with both GISTs and paragangliomas (referred to as the Carney–Stratakis dyad) and germline mutations in all four SDH genes have been found in patients with early-onset apparently sporadic GIST. GISTs in SDHx carriers tend to lack KIT and PDGFRA mutations (wild type), to be wild type (i.e., not associated with an acquired KIT mutation), show loss of heterozygosity for the corresponding SDHx gene as expected according to the two-hit hypothesis, and also demonstrate loss of immunostaining for SDHB. SDHB mutations have been found in 4.4% of patients with apparently isolated familial, early-onset, or bilateral renal cell carcinoma, and renal tumors in SDHB mutation carriers show biallelic inactivation of the SDHB locus and loss of immunostaining of the SDHB protein, consistent with SDHB having a causative mechanism in these tumors. Similarly, there is growing evidence for a role of SDHx in pituitary tumors, which have been reported in a few cases. There are several case reports of papillary thyroid cancer in SDHx carriers, but SDHx does not seem to be causative, but rather an incidental finding. Several other neoplasms have been reported in SDHx mutation carriers; however, it is not clear that the reported incidence exceeds that of the frequency in the general population.
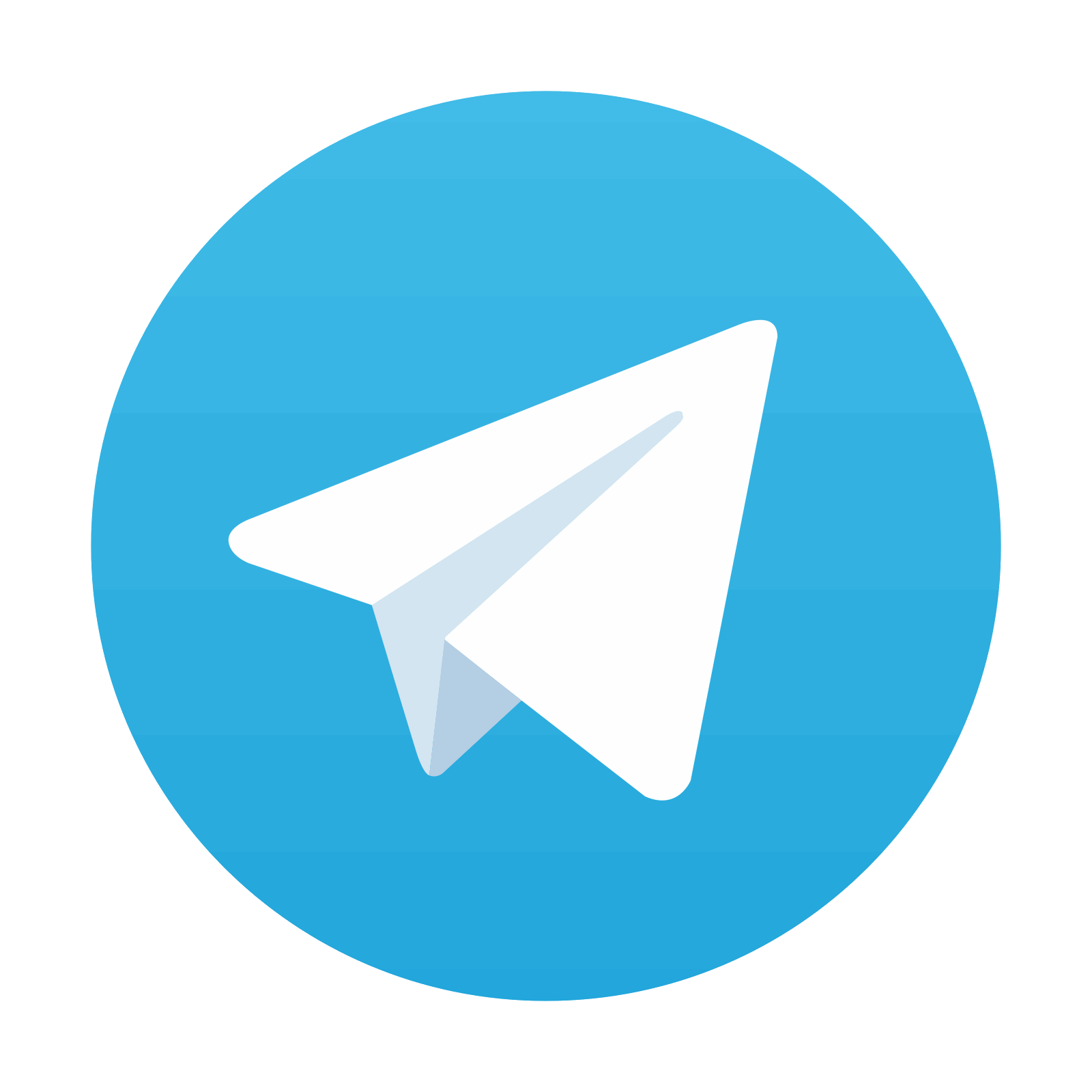
Stay updated, free articles. Join our Telegram channel

Full access? Get Clinical Tree
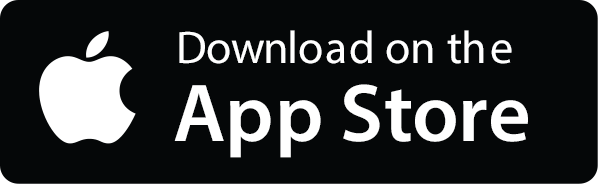
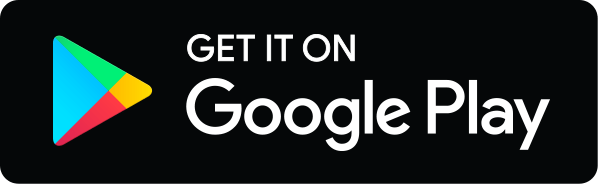