Abstract
Developmental anomalies of the thyroid gland (thyroid dysgenesis) underlie the majority of cases of congenital hypothyroidism. Only a small number of monogenic defects have been shown to result in athyreosis or orthotopic thyroid hyperplasia, whereas the commonest developmental anomaly, thyroid ectopy, remains unexplained. Ectopy may result from multiple genetic or epigenetic hits in the germline and/or at the somatic level. This chapter gives a brief overview of the monogenic defects in candidate genes that have been identified so far and of the syndromes that are known to be associated with thyroid dysgenesis. In addition, we discuss gain-of-function mutations of the thyroid hormone stimulating receptor ( TSHR ) gene, which are a rare cause of congenital hyperthyroidism.
Keywords
congenital hypothyroidism, development, thyroid dysgenesis, PAX8, TTF1/NKX2.1, TTF2/FOXE1, TSHR, GLIS3, NKX2.5
Introduction
Permanent primary congenital hypothyroidism (CH), as estimated from systematic biochemical screening of newborns, occurs in about one in 2500 births, making it the most common congenital endocrine disorder. Approximately two-thirds of the cases are due to abnormalities in the development of the thyroid gland during organogenesis (which are collectively called thyroid dysgenesis), the remainder being due to functional disorders. Classically, thyroid dyshormonogenesis leads to goiter, although exceptional cases with thyroid atrophy have been described, blurring the distinction between dyshormonogenesis and dysgenesis. Although pseudodominant inheritance has been reported, thyroperoxidase deficiency, the commonest form of dyshormonogenesis in most populations, is typically inherited in an autosomal recessive fashion and is accordingly more common in populations with a high degree of consanguinity.
In contrast to dyshormonogenesis, the mechanisms underlying CH due to thyroid dysgenesis remain largely unknown, and the proportion of patients in whom a genetic cause has been identified remains extremely small. Indeed, this condition was traditionally considered as sporadic until a systematic survey in France revealed that 2% of patients identified by neonatal screening had an affected relative. This figure is 15-fold higher than what would be predicted by chance alone and clearly suggests a genetic mechanism in those cases . However, it should not be construed as evidence that there is a genetic component in all patients with CH from thyroid dysgenesis; that is, the 2% with a positive family history and the 98% without may be two discrete populations. It is also noteworthy that a positive family history does not always imply a common genetic mechanism, as illustrated by a pedigree with two athyreotic individuals, only one of whom had mutations inactivating the TSH receptor. The fact that CH caused by thyroid dysgenesis, especially ectopy, has a marked female predominance is also incompatible with simple Mendelian inheritance. A greater prevalence among less genetically diverse populations, such as Caucasians, may suggest a genetic susceptibility. However, the major argument against Mendelian mechanisms is the observation that at least 92% of monozygotic twin pairs are discordant for this condition. This suggests that early postzygotic mutations or epigenetic modifications account for the vast majority of cases. A two-hit model integrating the discrepant observations of a higher-than-expected percentage of familial cases and the almost universal discordance of monozygotic twins has been proposed but remains speculative at this stage. Therefore, the remainder of this chapter mostly reviews the single gene disorders that have been shown to cause CH due to thyroid dysgenesis, but the reader should be aware that these only account for a small proportion of cases. Mutations that activate the TSH receptor are also briefly reviewed, since one of their modes of presentation is congenital hyperthyroid goiter.
Before embarking on a description of these single-gene disorders, the reader should also be aware that thyroid dysgenesis is a heterogeneous condition. Because ultrasound examination generally fails to reveal its most common form (ectopic thyroid), the gold standard for differentiating between the various forms of thyroid dysgenesis remains radionuclide scintigraphy with 99m sodium pertechnetate or 123 iodine. In 50% of the cases, this will reveal only a small round or oval mass of ectopic thyroid tissue, usually at the base of the tongue, suggesting an arrest in thyroid migration during embryogenesis; in about 9% of newborns with thyroid ectopy, two clusters of cells are visible. In the remainder, no uptake of isotope will be detectable. In this situation, an undetectable plasma thyroglobulin documents true athyreosis (a term to be preferred to agenesis, because there may have been a thyroid at some point during embryogenesis that later disappeared). We have suggested describing the situation in which there is no detectable uptake but a measurable thyroglobulin as “apparent athyreosis.” In only a small proportion of cases of overt CH (less than 5%) will imaging reveal a small thyroid gland in the normal location and with the normal bilobed shape (“orthotopic” hypoplasia) and in an even smaller proportion the absence of one thyroid lobe (generally the left) and sometimes of the isthmus. It is humbling that, after almost two decades of intensive research, the monogenic defects described later have been mostly documented in patients with the rarest variant of thyroid dysgenesis, that is, orthotopic thyroid hypoplasia, and that most cases of the commonest variant, ectopic thyroid, remain unexplained. Ectopic thyroid tissue seldom needs to be resected, which has hampered the search for somatic mutations. In only one case have mutations in the coding regions of the candidate genes been excluded. On the other hand, a transcriptome analysis revealed the presence of calcitonin mRNA in lingual thyroids. This was confirmed by RT-PCR and immunohistochemistry and challenges the long-held dogma that parafollicular cells originate only from the lateral thyroid anlagen.
We give herein an overview of the mutations in genes that have an impact on thyroid development. For molecular analysis of a potentially affected patient, the clinician should contact individual research groups (see References) or consult https://www.nextgxdx.com/ .
TSH receptor gene mutations (loss of function)
The TSH receptor (TSHR) mediates all the effects of TSH on thyroid growth and function but is not required for thyroid migration. It is encoded by a gene that was sequenced in 1989 and mapped to chromosome 14q31 in 1990. Mutations resulting in inactivation of the TSHR were first reported in 1995 by Sunthornthepvarakul et al. in three sisters with asymptomatic hyperthyrotropinemia, normal serum-free thyroxine (fT 4 ), and a thyroid gland of normal size and normal radioiodide uptake. Since then, many different inactivating TSHR gene mutations have been reported ( http://gris.ulb.ac.be ). Detailed information on the characteristics of the TSHR gene and of all the other genes discussed in this chapter is given in Table 8.1 . The phenotype of homozygotes and compound heterozygotes is very variable, ranging from asymptomatic hyperthyrotropinemia, as in the three sisters described originally, to severe CH with “apparent athyreosis,” as defined above. Careful ultrasonography reveals a small thyroid of normal shape and in the normal position. The contrast between the small amount of thyroid tissue and the elevated plasma thyroglobulin (TG), which has been observed in the affected neonates, is thought to be due to “leakage” of TG from disorganized follicles, as seen in the hyt / hyt mouse with a naturally occurring mutation that inactivates Tshr . Sequencing of TSHR should therefore be considered in patients with the phenotypes described above, especially if there is parental consanguinity or a family history suggestive of autosomal recessive transmission.
Gene (abbreviation) | Chromosomal location | Genomic size (in kb) | Exons | Transcript length (nt) starting at the “A” of the first A TG | Amino acids |
---|---|---|---|---|---|
Thyrotropin receptor (TSHR) | 14q31 | 190 | 10 | 2295 | 764 |
Paired box gene 8 A (PAX8) | 2q12-14 | 62 | 12 | 1353 | 450 |
Thyroid transcription factor 1 (TTF1, TITF1, NKX2.1 or T/EBP) | 14q13 | 3.7 | 3 | 1206 | 401 |
Thyroid transcription factor 2 (TTF2, TITF2, FOXE1 or FKHL15) | 9q22 | 3.4 | 1 | 1122 | 373 |
GLIS3 | 9p24.3-p23 | 328 | 10 | 2328 | 775 |
NKX2.5 | 5q34 | 3 | 2 | 975 | 324 |
Unknown | 15q25.3-26.1 | ? | ? | ? | ? |
While TSH resistance from inactivating mutations in the TSHR gene is generally described as being transmitted in a recessive manner, it has now become apparent that heterozygotes can have a mild phenotype, with mild hyperthyrotropinemia and a normal or small gland, which is transmitted in a dominant fashion. In a study from Italy, 12% of patients younger than 18 years of age with mild hyperthyrotropinemia, an orthotopic thyroid, and no evidence of autoimmunity had heterozygous TSHR gene mutations. It has been argued that making this diagnosis is clinically relevant, because it allows stopping treatment and follow up.
In another form of dominantly transmitted TSH resistance, which appears to be common, the TSHR gene has been excluded and a locus on chromosome 15 identified.
PAX8 gene mutations
PAX8, a transcriptional factor playing an important role in the initiation of thyrocyte differentiation and maintenance of the follicular cell, is encoded by a gene on chromosome 2q12-14. Containing a highly conserved 128 amino acid-paired domain, PAX8 recognizes specific DNA response elements and regulates the expression of TG, thyroperoxidase (TPO), and the sodium iodide symporter (NIS) by binding to their promoter regions. Furthermore, PAX8 and the thyroid transcription factor 1 (TTF1) synergistically activate the promoter of human TG. Whereas Pax8 deficient mice have hypoplastic thyroid glands with absent follicular cells, mice heterozygous for targeted disruption of the Pax8 gene do not display an obvious thyroid phenotype. The human PAX8 gene consists of 11 exons encoding a 450 amino acid protein. In humans, heterozygous PAX8 loss-of-function mutations can be associated with orthotopic, thyroid hypoplasia, or to no overt structural abnormality of the thyroid gland. The functional status of affected individuals is very variable even within the same family, ranging from severe hypothyroidism to compensated hypothyroidism to euthyroidism. This extreme variability supports the hypothesis that many factors modulate the phenotypic expression of PAX8 gene mutations. This is supported by work in mice showing that the phenotype of Pax8 deficiency is strain specific.
So far, 19 different mutations in the coding sequence of the PAX8 gene have been reported. The majority of these are located in the paired domain (L16P, F20S, P25R, R31C, R31H, Q40P, G41V, D46SfsX24, S48F, R52P, S54G, S54R, H55Q, C57Y, L62R, R108X, R133Q). With the exception of S48F, which has a normal binding affinity to DNA but an impaired capacity to recruit the general coactivator p300 and a dominant negative effect, these mutants result in severe reduction of DNA binding activity of PAX8. Dominant inheritance of the phenotype together with a heterozygous inactivating mutation is the rule, although all mutations are not fully penetrant and the mechanisms of disease expression in the presence of only one mutated allele are unknown. For one of the mutations (R133Q), which is located in the terminal portion of the DNA binding domain, the results regarding DNA binding ability are contradictory. The mutations (del989-992AAAC and T225M) outside the paired domain have a normal DNA binding ability in vitro . Whereas del989-992AAAC is truncated and transcriptionally inactive, an impaired synergistic effect of p300 on T225M-mediated transactivation has been described. Also sequence alterations in the 5′untranslated region of the PAX8 gene may cause diminished transcriptional activity. Hermanns et al. reported a girl with a monoallelic mutation in the PAX8 promoter who also had a heterozygous mutation in the NKX2-5 gene. In addition, a mutation in the PAX8 promoter at position-3, also causing impaired transcriptional activity in vitro , was found in a girl with Down’s syndrome and CH.
Except for three families with de novo PAX8 gene mutations, dominant inheritance with widely variable penetrance is the rule. The initial report of a patient with an ectopic gland and a PAX8 mutation was not based on scintigraphy, and this patient likely had orthotopic hypoplasia, as have the vast majority of the cases studied since. In a few patients normal thyroid volumes have been found and, in some, cystic thyroid rudiments have been reported on ultrasound.
It is of particular interest that one patient with a heterozygous PAX8 gene mutation was phenotypically normal at birth but his thyroid became hypoplastic postnatally probably because of deficient postnatal growth of the gland. Therefore, even an unremarkable neonatal screening result for CH and a normally developed and located thyroid gland at birth does not rule out the possibility of a PAX8 gene deficiency.
It should be noted that one individual with a heterozygous PAX8 loss-of-function mutation had a positive perchlorate discharge test. Another carrier of a PAX8 gene mutation was suspected to have a defective sodium iodide symporter because he had a low thyroidal uptake of I 123 and a low I 123 saliva-to-plasma ratio. This phenomenon, which might lead to the erroneous diagnosis of dyshormonogenesis, can be explained by the fact that TPO and NIS genes transcription is dependent on PAX8. Thus, impaired PAX8 function can cause reduced TPO or NIS expression and consequently to a partial organification defect. The PAX8 gene is also expressed in the kidney. Recently, a family with a PAX8 gene mutation responsible for a severe form of dominantly inherited CH was described. The mutation seemed to be associated with abnormalities of the urogenital tract (incomplete horseshoe kidney, undescended testicles, hydrocele, and ureterocele). However, renal abnormalities seem to be uncommon in individuals with PAX8 gene mutations and have only been reported in two other affected cases.
Given the extreme variability of the thyroid phenotype in patients with mutations in the PAX8 gene, it is difficult to define in which patients these should be looked for. However, these mutations appear to be quite rare and we would suggest to screen only patients with a family history suggestive of dominant transmission or early-onset CH and an in situ gland of normal or reduced size.
TTF1/NKX2-1 gene mutations
TTF1 (also known as NKX2-1, T/EBP (thyroid-specific-enhancer-binding protein) or TITF1) is a member of the homeobox domain type of transcription factors and is encoded by a gene on chromosome 14q13. Its role in the development of the thyroid gland and of other organs was clearly shown by the observation that Ttf1 –/– mice had complete absence of follicular and parafollicular cells as well as agenesis of lung parenchyma, ventral forebrain, and anterior and posterior pituitary. Reexamination of Ttf1 + / – mice, initially reported to be normal, revealed a mild thyroid and neurological phenotype, demonstrating that “half a loaf is not enough.” Studies trying to correlate the severity of the thyroid and lung phenotypes with the effect of the mutation on organ-specific reporter genes in vitro have yielded conflicting results.
Subsequently, the role of its human homolog, TTF1, in the pathophysiology of CH, was suggested by the observation of patients with CH who had chromosomal deletions encompassing the TTF1 locus, including in a sib pair. Next, point mutations in the TTF1 gene confirmed its implication in the phenotype, which includes CH with a thyroid gland in place associated with respiratory distress syndrome and with neonatal hypotonia followed by choreoathetosis or ataxia. “Benign hereditary chorea” (BHC) had been defined clinically and is now known to be due to dominantly inherited TTF1 mutations. The severity of the three components of the “brain-thyroid-lung” syndrome is very variable. Thyroid insufficiency is generally the least severe of the three, the neurological phenotype intermediate and the lung phenotype the most severe. Indeed, neonatal death from lung hypoplasia has been described. Heterozygous TTF1 gene mutations lead to a phenotype likely through haploinsufficiency.
In 2009, a systematic review of 46 cases with variants of TTF1 showed that TTF1 mutations occur either de novo or via an autosomal dominant mode of inheritance. In addition, this review showed that only 50% of the cases had involvement of all three organs, 30% showed CH and a neurologic disorder, 13% presented with isolated BHC, and only 7% had no neurologic phenotype. Functionally, hyperthyrotropinemia was more common at diagnosis than overt hypothyroidism (61% vs. 39%). Thyroid morphology was normal in 55%, with hypoplasia or hemiagenesis in 35% and athyreosis in 10%. Sixteen percent died of severe lung disease. Recently, it has been shown that TTF1 gene mutations might be associated with severe pulmonary disease as the only clinical manifestation in the absence of thyroid and neurologic abnormalities. In CH, on the other hand, TTF1 gene mutations have only been found in patients in association with another organ involvement ( Table 8.2 ) and searching for TTF1 gene mutations in patients with isolated CH from thyroid dysgenesis has yielded negative results. Lastly, the “brain-thyroid-lung” syndrome has also been observed in one child bearing a 14q13.1-3 deletion adjacent to, but not involving TTF1 , and in another without identified molecular cause.
Thyroid phenotype | Other features | Gene | Transmission |
---|---|---|---|
From apparent athyreosis to normally appearing gland | None | TSHR | AR |
Normally appearing gland or orthotopic hypoplasia | None | ? on 15q25.3-26.1 | AD |
From apparent athyreosis to normal gland, usually mild ↑ TSH |
| TTF1 | De novo or AD |
From apparent athyreosis to normally appearing gland |
| PAX8 | AD or de novo |
True athyreosis |
| TTF2 | AR |
Apparent athyreosis |
| GLIS3 | AR |
TTF2 (FOXE 1 or FKHL15) gene mutations
Thyroid transcription factor 2 (FOXE1, TTF2, or FKHL15) is a member of the forkhead/winged helix domain protein family and is encoded by a gene on chromosome 9q22. It regulates transcription of TG and TPO. FOXE1 binds to specific regulatory DNA sequences in the promoter regions through its highly conserved forkhead domain. The human gene is located in chromosome 9q22. It consists of a single exon, which encodes a 373 amino acid protein. Foxe1 –/– mice embryos have cleft palates and athyreosis or an ectopic sublingual gland, whereas heterozygous Foxe1 knockout mice are phenotypically normal.
So far, only five FOXE1 gene mutations have been identified in humans. In the first report of a human FOXE1 gene mutation, two Welsh boys with athyreosis, cleft palates, spiky hair, bilateral choanal atresia, and hypoplastic bifid epiglottis (so-called Bamforth–Lazarus syndrome ) were identified to have a homozygous FOXE1 gene mutation (A65V), which is located in the highly conserved forkhead DNA-binding domain. In vitro studies showed that this mutant FOXE1 protein displayed impaired DNA binding and no transcriptional function. Interestingly, one of the parents had unilateral choanal atresia. In the second family, two affected individuals of Tunisian origin with thyroid athyreosis, cleft palates, and spiky hair but neither choanal atresia nor bifid epiglottis were described. Both boys were also homozygous for a mutation in the forkhead domain of FOXE1 (S57N), but in vitro studies of the mutant protein showed only partial loss of DNA binding and retained some transcriptional activity, which might explain the normal epiglottis and choanae. The third patient with a known FOXE1 gene mutation (R102C) was of Turkish origin. She presented with CH and also had a cleft palate, bilateral choanal atresia, and spiky hair. Her mutation was located in the forkhead DNA-binding domain of FOXE1 and encodes a defective FOXE1, which has negligible DNA binding and transcriptional activity. Neck ultrasound and CT examination showed hyperechoic, soft, nonenhancing tissue at the site of the normal thyroid lobes. However, 123 I uptake was negligible, and plasma TG was very low. It is therefore likely that the structures seen on ultrasound and CT were the ultimobranchial bodies and that, in spite of the authors’ claim to the contrary, the thyroid phenotype was true athyreosis, as in the two previously reported pedigrees.
Another mode of inheritance of FOXE1 gene mutations was reported by Castanet et al. in a patient with CH due to severe thyroid hypoplasia, a cleft palate, and spiky hair who had a homozygous missense mutation (F137S) in the FOXE1 gene. His mother was an unaffected heterozygous carrier of the mutation, but the father was homozygous for the wild-type sequence. Using microsatellite markers and multiplex ligation-dependent probe amplification, the authors demonstrated paternity and complete maternal uniparental disomy. Therefore, FOXE1 gene mutations should also be considered in nonconsanguineous families. Very recently, Carré et al. identified a new FOXE1 gene mutation (R73S) in a patient with athyreosis, a cleft palate, and partial choanal atresia. Interestingly, this mutation led to increased thyroidal gene expression in vitro by enhancing the TG – and TPO -promoters. In another study, Carré et al. suggested a different mechanism whereby FOXE1 may play a role in thyroid dysgenesis. They found that the lengths of the polyalanine tract within the FOXE1 gene differed between patients and controls. However, the length of the polyalanine tract was the same in the affected and the unaffected twin of four discordant monozygotic pairs. These seemingly contradictory observations underscore the complexity of the genetic and epigenetic mechanisms involved in thyroid dysgenesis.
Given the extreme rarity of mutations in the FOXE1 gene, it seems reasonable to restrict the search for mutations in this gene to patients with at least three elements of the Bamforth–Lazarus syndrome. Indeed, sequencing of the FOXE1 gene in patients with “only” CH and cleft lips/palates has yielded negative results.
Nevertheless, as Foxe1 is the only gene known to be associated with thyroid ectopy in animal models, a broader association between FOXE1 gene polymorphisms and thyroid dysgenesis is suspected. As described earlier, the length of the polymorphic FOXE1 gene alanine stretch is associated with thyroid dysgenesis. In human leukocytes and thyroid tissues, the methylation status of a tissue-dependent differentially methylated region (DMR) in the FOXE1 gene promoter shows an inverse correlation with FOXE1 expression. Whether rare genetic variants in the FOXE1 DMR are associated with CH due to thyroid ectopy is currently under investigation.
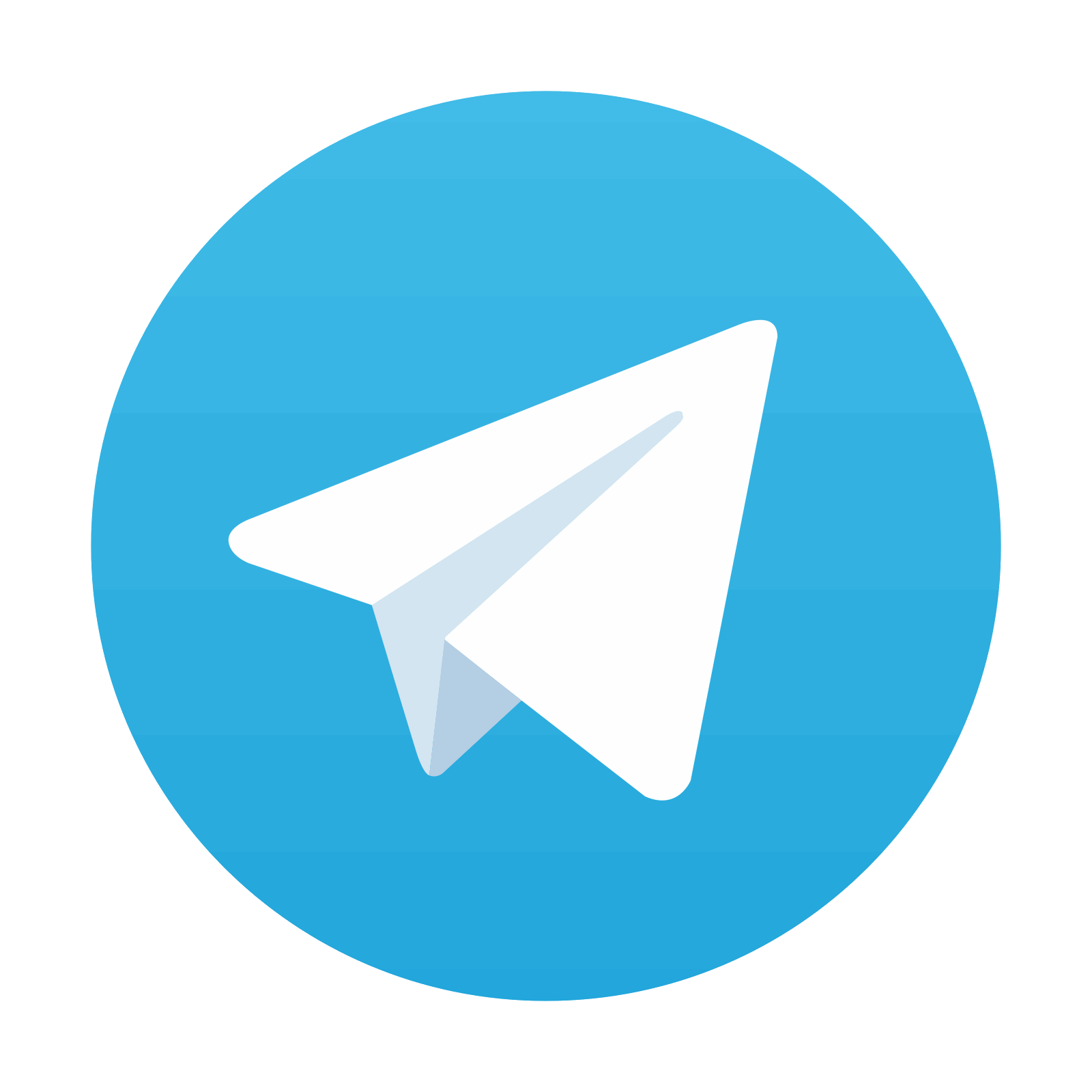
Stay updated, free articles. Join our Telegram channel

Full access? Get Clinical Tree
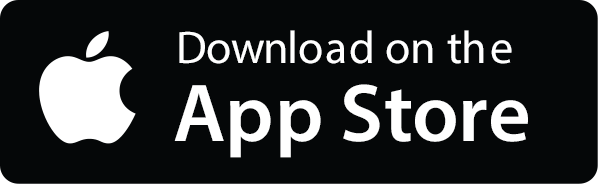
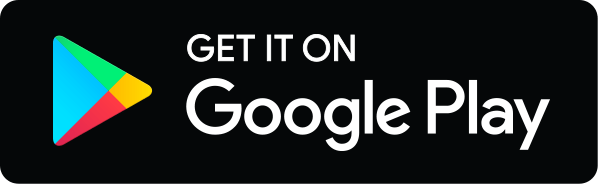