Hemostasis and Platelet Disorders
Marcia Paddock, MD, PhD
John V. Mitsios, PhD
Maria T. DeSancho, MD, MSc
HEMOSTASIS AND ITS ROLE IN DISEASE
Hemostasis
Hemostasis is the process by which vascular integrity is maintained. Hemostasis is dependent on multiple components, including vascular endothelium, platelets, von Willebrand factor (vWF), and soluble plasma proteins. The process may be broken down into two main phases termed primary and secondary hemostasis. Primary hemostasis refers to the formation of the platelet plug, and secondary hemostasis refers to the role of the coagulation cascade in forming a fibrin clot, which together with platelets generates thrombus. Briefly, formation of the platelet plug is initiated by platelet adhesion to tissue factor, collagen, von Willebrand factor (vWF), or fibronectin exposed on the subendothelial matrix during vascular injury. This initial contact activates platelets, resulting in increased expression of surface proteins and release of granules that contain factors that enhance coagulation. The activated platelets express on their surface membranes glycoprotein complex IIb-IIIa, which binds fibrinogen, cross-linking the platelets together in a process called aggregation. This process is discussed in more detail in the section on platelets.
Secondary hemostasis refers to the role of the plasma coagulation factors in generating thrombin that cleaves fibrinogen to insoluble fibrin and supports the initial platelet plug. This network of platelets and fibrin is cross-linked by factor XIII to stabilize it and form a chemically stable thrombus in which red cells are enmeshed to provide bulk.
The coagulation cascade of plasma clotting factors is classified into the extrinsic, intrinsic, and common pathways (Fig. 4.1). The extrinsic pathway is initiated when blood is exposed to tissue factor (TF), a transmembrane glycoprotein expressed in vessel walls and endothelial cells. Tissue factor binds factor VII, resulting in its activation to factor VIIa, which activates factor X and generates the prothrombinase complex composed of Xa and Va. The prothrombinase complex cleaves prothrombin (factor II) to thrombin (factor IIa), a key enzyme in the coagulation cascade. Thrombin both cleaves fibrinogen to form fibrin, which is then cross-linked by factor XIII to form a stable clot, and also activates factors V, VIII, and XI, adding to thrombin activation. In contrast, fibrin deposition is limited and controlled by an endogenous anticoagulant system. Antithrombin (AT) is a plasma protein member of the serpin (serine protease inhibitor) family that inhibits the activities of all of the activated coagulation enzymes, particularly factor IIa and Xa. Protein C is a vitamin K-dependent protein that proteolytically inactivates activated factor Va and factor VIIIa, thus blocking their ability to generate more thrombin. Protein C binds to an endothelial cell protein C receptor (EPCR) and is activated by thrombin bound to thrombomodulin, another endothelial cell membrane-based protein, in a reaction that is promoted by a cofactor, protein S (Fig. 4.2). Thus, thrombin not only leads to clot formation but also contributes to clot limitation through the activation of protein C. In a parallel fashion, tissue factor pathway inhibitor is a plasma protein that forms a quaternary complex with tissue factor, factor VIIa, and factor Xa that inhibits the extrinsic coagulation pathway.
The extrinsic pathway is thought to be the primary method of activating the coagulation in vivo and is critical for normal hemostasis. This pathway is measured with the prothrombin time (PT).
The intrinsic pathway—composed of factors XII, XI, IX, and VIII—is activated by negatively charged surfaces such as inorganic polyphosphates released from platelet-dense granules upon platelet activation that start a cascade of activation of these components, which again generates the prothrombinase complex of factors Xa and Va and produces fibrin from fibrinogen, as described above. The intrinsic pathway is measured by the activated partial thromboplastin time (aPTT). Maintaining normal hemostasis depends on all these factors working in concert, and alterations in the system, either inherited or acquired, will lead to dysregulation
of normal hemostasis with subsequent bleeding or thrombosis (i.e., excessive clotting).
of normal hemostasis with subsequent bleeding or thrombosis (i.e., excessive clotting).
An alternative model of coagulation is the cell-based model proposed by Drs. Hoffman and Monroe in 2001.1 In this model, coagulation is regulated by properties of cell surfaces in three overlapping stages of initiation, amplification, and propagation, rather than the traditional cascade (Fig. 4.3). This model is appealing because it may more closely approximate what happens in vivo rather than the cascade model, which is based largely on in vitro enzyme activity.
Fibrinolysis
As hemostasis refers to the formation of a blood clot to preserve vascular integrity, the fibrinolytic system refers to the removal of blood clots no longer required, via a cascade of serine proteases that degrade fibrin and result in clot dissolution. Activation of the circulating zymogen called plasminogen into its active form, plasmin, is achieved mainly by tissue-type plasminogen activator (t-PA). This catalysis is highly dependent on the presence of fibrin, which by binding both plasminogen and t-PA increases plasmin generation by more than two orders of magnitude. Mechanistically, plasminogen binds to exposed lysine residues formed in fibrin, and these binding sites increase in number during fibrin cleavage, allowing more plasminogen binding to occur, thereby amplifying the process, allowing more plasmin to be generated. Naturally occurring plasmin inhibitors (α2 antiplasmin, plasminogen activator inhibitor [PAI]-1, and PAI-2) also exist that limit plasmin activity or its generation in the circulation. These include plasma protein forms or inhibitors released by activated platelets. The most potent of these is α2 antiplasmin. While bound to fibrin, plasmin is largely protected from α2 antiplasmin, allowing fibrin cleavage to occur. Other key regulatory steps in fibrinolysis occur at the level of the plasminogen activators, as the activities of t-PA and also urokinase-type plasminogen activator (u-PA), the second important endogenous plasminogen activator, are both regulated by PAI-1 and PAI-2.
The most recently described mechanism that limits the fibrinolytic system is via “thrombin activatable fibrinolysis inhibitor.” The plasma protein, thrombin activatable fibrinolysis inhibitor (TAFI), is a carboxypeptidase that specifically cleaves exposed lysine residues from fibrin, thereby removing the ability of plasminogen and t-PA to dock onto lysine-binding sites in fibrin. Because it requires activation by thrombin, TAFI becomes engaged as a direct consequence of coagulation to stabilize and protect clots from premature removal by the fibrinolytic system.
BLEEDING DISORDERS
Platelet Disorders
Stable clot formation depends on an appropriate platelet number, normal platelet function, and adequate coagulation factors. The role of platelets can be summarized as the three A’s: adhesion, activation, and aggregation. Adhesion refers to the initial binding of a single layer of platelets to sites of vascular injury and is mediated by exposure of tissue factor, collagen, von Willebrand factor (vWF), and fibronectin on the subendothelial matrix during endothelial damage. This initial contact leads to platelet activation, which causes a flipping of the polarity of inner and outer leaflets of the platelet surface membrane. The exposure of anionic phospholipids at the platelet surface serves as platforms for the assemblies of blood coagulation enzyme complexes and release of platelet granules containing factors that further enhance coagulation at the site of vascular injury (Fig. 4.4). Platelet aggregation on the initial layer of adherent platelets at the injury site then occurs when fibrinogen binds to the glycoprotein IIb-IIIa complexes expressed on multiple activated platelets and is converted to fibrin through local activation of the clotting cascade on the platelet surface, forming a platelet plug.2 Defects at any step in this complex process can lead to bleeding disorders as described in the following sections.
Thrombocytopenia
Thrombocytopenia represents a decrease in the platelet count below the normal range (150,000-450,000/mm3) and is associated with petechial and mucosal bleeding. In evaluating apparently low platelets on the automated complete blood count (CBC), pseudothrombocytopenia must be excluded. Pseudothrombocytopenia is an in vitro phenomenon in which platelets aggregate in the presence of ethylenediamine tetra-acetic acid (EDTA), the anticoagulant in lavender-top specimen tubes used for blood counts. If platelets are found clumped as in Figure 4.5, the count in the CBC is not reliable and must be repeated. Pseudothrombocytopenia can be detected in most cases by redrawing blood for the CBC in a sodium citrate (blue top) tube or by making a peripheral blood smear from a fingerstick blood specimen and counting the platelets manually.3 Once it is established that the low platelet count is real, the differential diagnosis of thrombocytopenia includes underproduction, excessive consumption or destruction, or sequestration in enlarged spleen or liver. Underproduction may be either hereditary or acquired, as seen in hematologic malignancies or with metastases of solid tumors to the bone marrow. Consumption in vivo can result from active bleeding, from platelet trapping in artificial membranes during cardiopulmonary bypass, or as a manifestation of disseminated intravascular coagulation (DIC). Platelet destruction is seen with idiopathic immune thrombocytopenia (ITP), drug-induced thrombocytopenia, infection-induced thrombocytopenia, and thrombotic microangiopathies (TMAs). Sequestration most often occurs in patients with splenomegaly. Splenomegaly is noted on the physical or radiologic examination of patients with liposomal storage diseases such as Gaucher disease, or with lymphoma, chronic lymphocytic leukemia, autoimmune disorders, thalassemias, portal hypertension, and some infections.
Immune Thrombocytopenic Purpura
One of the most common causes of severe thrombocytopenia (platelet count <50,000/mm3) is immune thrombocytopenic
purpura (ITP). ITP has a slight female preponderance (1.2-1.7 times more frequent in women than men in different studies) and may have increasing incidence with age. In children, ITP typically arises after a viral infection, has an abrupt onset and a limited course, whereas in adults ITP is more likely to have a gradual onset and to be chronic or relapsing. A patient with severe immune thrombocytopenia usually presents with mucocutaneous bleeding such as epistaxis, gingival bleeding, or a petechial rash; menorrhagia is often the presenting symptom in women of reproductive age (Figs. 4.6 and 4.7). The development of any of these symptoms should prompt an evaluation of the platelet count. Platelet counts below 30,000/mm3 may lead to petechiae and ecchymosis or bleeding with minor trauma. Platelet counts below 10,000/mm3 may result in spontaneous bleeding, although clinically significant bleeding is rare in ITP.
purpura (ITP). ITP has a slight female preponderance (1.2-1.7 times more frequent in women than men in different studies) and may have increasing incidence with age. In children, ITP typically arises after a viral infection, has an abrupt onset and a limited course, whereas in adults ITP is more likely to have a gradual onset and to be chronic or relapsing. A patient with severe immune thrombocytopenia usually presents with mucocutaneous bleeding such as epistaxis, gingival bleeding, or a petechial rash; menorrhagia is often the presenting symptom in women of reproductive age (Figs. 4.6 and 4.7). The development of any of these symptoms should prompt an evaluation of the platelet count. Platelet counts below 30,000/mm3 may lead to petechiae and ecchymosis or bleeding with minor trauma. Platelet counts below 10,000/mm3 may result in spontaneous bleeding, although clinically significant bleeding is rare in ITP.
ITP is a diagnosis of exclusion and requires isolated thrombocytopenia in the absence of an alternate explanation for low platelets. For example, splenomegaly is rare in ITP. The peripheral blood smear is normal except for low platelet numbers, with large or giant platelets seen on the smear, evidence of platelets being released early from the megakaryocytes in the bone marrow due to high demand. The reticulated platelet count (measured with an acridine orange stain) is elevated in ITP, confirming the increase in “young” platelets. Although tests for antiplatelet antibodies exist, they are not either sensitive or specific enough to warrant regular use in diagnosing ITP. If a bone marrow biopsy is performed, an increased number of morphologically normal megakaryocytes in different stages of differentiation may be observed (Fig. 4.8), in contrast to essential thrombocythemia (ET), in which megakaryocytes are often dysplastic (Fig. 4.9). Bone marrow examination, however, is not required for diagnosis. Treatments for ITP include steroids, intravenous γ-globulin, Rh immunoglobulin (Winrho), splenectomy, rituximab, and thrombopoietin agonists such as eltrombopag and romiplostim (see Fig. 4.8). Splenectomy is the only treatment that is considered curative, but it is being used less frequently because many other therapeutic alternatives are available now.
Drug-Induced Thrombocytopenia
Thrombocytopenia may also be seen as an adverse response to numerous medications. Drug-induced thrombocytopenia may present with severe thrombocytopenia (nadir platelet count <20,000/mm3). Drugs cause thrombocytopenia by a variety of mechanisms, including direct antibody interactions that increase the affinity of the antibody for platelet antigens (as for quinine), drug interaction with platelet antigens creating a neoepitope (as for heparin), platelets reacting with a drug metabolite (as for sulfamethoxazole and trimethoprim), or an on-target effect of an therapeutic antiplatelet glycoprotein antibody (as for abciximab). Drug-induced thrombocytopenia resolves after stopping the offending agent and reoccurs after reintroduction of the drug. Heparin-induced thrombocytopenia (HIT) is a special case because it represents both a thrombocytopenic and a hypercoagulable state and will be discussed under the latter topic (Table 4.1).
Infection-Induced Thrombocytopenia
Thrombocytopenia may commonly be seen during severe infections or sepsis, in which it is often a negative prognostic sign associated with DIC. Specific insect-borne infections of red blood cells (RBCs) or white blood cells (WBCs) may also cause thrombocytopenia by other mechanisms such as vascular damage and platelet consumption or DIC. Infections that can cause anemia and thrombocytopenia include viral hepatitis, rickettsial infections, ehrlichiosis, babesia (Fig. 4.10), and Plasmodium falciparum malaria (Fig. 4.11).
Inherited Platelet Disorders
Inherited platelet disorders causing thrombocytopenia are rare. Platelets may be low as part of a bone marrow failure syndrome, or platelet defects that impair platelet production or render platelets less stable in circulation. Such thrombocytopenias may be associated with a syndrome such as Wiskott-Aldrich syndrome (WAS)/X-linked thrombocytopenia (XLT), thrombocytopenia with absent radii (TAR), or MYH9-related thrombocytopenia, or they may be rare primary platelet defects such as in Glanzmann thrombasthenia, Bernard-Soulier syndrome, gray platelet syndrome, or congenital amegakaryocytic thrombocytopenia (CAMT) (Table 4.2).5, 6, 7, 8 Making the diagnosis of these rare disorders requires advanced testing, the exception being gray platelet syndrome, which can be diagnosed on light microscopy because of the absence of α-granules in these platelets (Fig. 4.12).5
Acquired Platelet Disorders
Thrombocytopenia in hematologic malignancies or myelodysplastic syndromes is usually secondary to bone marrow dysfunction or to consumption coagulopathies (DIC) related to malignancy as in acute promyelocytic leukemia [Fig. 4.13] or bone marrow metastasis of solid tumors or trauma, or in severe obstetrical complications. In lymphoproliferative disease or autoimmune diseases where T-cell function is disturbed, ITP is frequently seen either alone or associated with autoimmune hemolytic anemia (as Evans syndrome).
Thrombocytosis
Thrombocytosis represents a marked increase in platelet count to above 500,000/µL and is classified as reactive or primary. In reactive thrombocytosis, the platelets are usually of normal size and have normal reactivity, whereas in primary thrombocytosis platelets are often large or varied in size and have altered functionality. Reactive thrombocytosis occurs in patients with iron-deficiency anemia, hemolysis, connective tissue disorders, inflammatory conditions, tuberculosis, cancer, or after surgery (commonly postsplenectomy). Once reactive thrombocytosis is excluded, primary thrombocytosis or essential thrombocythemia (ET) is likely. ET is characterized by a persistent elevated platelet count and the presence of a JAK2 V617F mutation in 60% of the patients, or an MPL gene substitution or a calreticulin (CALR) mutation; in about 10% of patients with ET, no mutations have been identified. Patients with JAK2 (V617F) mutation are older, have a higher hemoglobin level and WBC count, and a
lower platelet count and serum erythropoietin than those with a CALR mutation.9 Bone marrow megakaryocytes in reactive thrombocytosis show normal maturation, whereas in primary thrombocytosis, megakaryocytes have abnormal morphology. (For further discussion of essential thrombocytosis, see Chapter 8.)
lower platelet count and serum erythropoietin than those with a CALR mutation.9 Bone marrow megakaryocytes in reactive thrombocytosis show normal maturation, whereas in primary thrombocytosis, megakaryocytes have abnormal morphology. (For further discussion of essential thrombocytosis, see Chapter 8.)
Laboratory Testing of Platelet Function
Platelet functional assays such as the PFA-100 and platelet aggregometry measure qualitative platelet defects and serve as screening tests. In the past, platelet function was assessed by the bleeding time, the time in seconds required for bleeding to stop from a small skin wound made in a standard manner. Bleeding time has since been largely discarded because of variability and the facts that its performance is labor intensive and operator dependent. The PFA-100 test was designed to replace the bleeding time and measures how long a sample of whole blood takes to clot when passed through a special cartridge in which platelets are activated by either collagen + epinephrine or collagen + adenosine diphosphate (ADP) (Fig. 4.14).10 If the platelets are normally activated by these agonists, the clot forms quickly within the normal time range of the test; if platelet function is deficient, the time to clotting is prolonged or indefinite. This test uses a freshly drawn tube of blood, can be done in the laboratory rather than at the bedside, does not require the presence of the patient, and the results are less variable than with the classic bleeding time.
The most common cause of platelet dysfunction, by far, is medication taken by the patient, often over-the-counter drugs. Aspirin is a frequently used drug that impairs platelet aggregation and release and does so in very low doses and with a prolonged effect (up to a week). Beyond drug effects on platelets, platelet function may also be intrinsically abnormal (poor) in uremia, von Willebrand disease (vWD), liver disease, autoimmune disease such as lupus, and the hereditary thrombocytopathies, whereas it may be hyperactive in arteriosclerosis and diabetes.
Aspirin and nonsteroidal anti-inflammatory drugs (NSAIDs) cause a prolongation specifically of the collagen-epinephrine aggregation time in the PFA-100. Other antiplatelet medications such as clopidogrel or ticagrelor, other over-the-counter medications such as guaifenesin, some herbal supplements such as fish oil, or certain antidepressants all can depress platelet function and impair hemostasis. Therefore, a careful medication history is necessary in evaluating hemostasis. In uremia, vWD, and liver disease, both types of PFA-100 results may be abnormal. The PFA-100 has a high negative predictive value; for example, if the PFA-100 gives a normal result, then with a few exceptions (storage pool disease, primary secretion defects, and mild type 1 vWD), primary hemostasis is intact and may eliminate the need for further screening of platelet function. However, if more specific testing is required, complete platelet aggregometry can be performed on either platelet-rich plasma or whole blood. Complete aggregometry includes using a panel of soluble platelet agonists, including ADP, epinephrine, collagen, a thromboxane A2 mimetic, arachidonic acid, thrombin receptor-activating peptide, and ristocetin. By correlating which platelet agonists are able to induce aggregation and which do not, specific defects in platelet function can be determined (Fig. 4.15).11
Coagulation Factor Deficiencies
Inherited Factor Deficiencies
vWD is the most common congenital bleeding disorder, with an estimated prevalence of up to 1% of the population, although the bleeding phenotype is variable. The disease is autosomal dominant in inheritance and represents the most common bleeding disorder in women. Bleeding is typically into skin and mucosae—petechiae and ecchymoses—rather than joint or deep tissue bleeding, and menorrhagia is common in affected women. In vWD, the large multimeric plasma glycoprotein, vWF, necessary for normal platelet adhesion to the subendothelium and also for stabilizing circulating coagulation factor VIII, is decreased or absent. When vWD is inadequate, platelet plug formation is depressed and bleeding occurs in a mucosal and petechial fashion. The severity of vWD ranges from very mild (type I) to severe (type III); in the latter, the vWF level is so low that factor VIII levels are as low as in classic hemophilia, and the clinical phenotype is that of hemophilia A complicated by defective platelet adhesion.
In assessing vWD, several tests are available:
Antigen level (vWF:Ag)—a quantitative measure of the vWF protein without information about its function
Activity (vWF:RCo)—a functional assay that measures platelet aggregation after addition of the activator ristocetin to the patient’s plasma
vWF:RCo/vWF:Ag ratio—the ratio between antigen and activity levels, which can help distinguish type I from type II vWD
Factor VIII levels—vWF is required to maintain FVIII in circulation
Ristocetin-induced platelet aggregation (RIPA and low-dose RIPA)—patients with type IIB vWD demonstrate enhanced platelet aggregation in response to stimulation with low-dose ristocetin
Multimer assay—vWF functions optimally as a large multimer, and electrophoresis for multimer size helps to distinguish subtypes of vWD
vWD is classified into three main types, as shown in Table 4.3. Type I is a quantitative deficiency of vWF and can be mild, moderate, or severe, depending on the level of the protein deficiency. In von Willebrand assays, this will result in concordantly low von Willebrand antigen and activity, maintaining a preserved vWF:RCo/vWF:Ag ratio. vWD type IC has been described and results from increased VWF clearance from the circulation. Type II vWD represents a qualitative deficiency of vWF activity and is characterized by a decreased ratio of vWF:RCo/vWF:Ag (often <0.7). There are multiple subtypes of type II vWD, including IIA, IIB, IIM, and IIN—each characterized by a different defect. vWD IIA is the most common subtype and results from a deficiency
of the high-molecular-weight multimers of vWF, which are required for efficient binding to platelets, that causes the observed bleeding phenotype (Fig. 4.16). vWD type IIB is a gain-of-function mutation that causes increased binding to platelet glycoprotein Ib; however, as this binding occurs inappropriately without hemostatic challenge, it leads to a consumption of platelets and the larger WVF multimers.12 As such, treatment of bleeding with deamino-8-D-arginine vasopressin (desmopressin acetate or DDAVP), which releases vWF from endothelial storage sites and thus improves the clinical status in type I vWD, is relatively contraindicated in type IIB owing to the risk of worsening bleeding by increasing the consumption of platelets. VWD IIM is a defect in the ability of vWF to bind platelets. Although both levels of VWF protein and HMW multimers are present, vWF activity is decreased, as measured by platelet aggregation in response to activation with ristocetin. vWD type IIN (Normandy) subtype is distinct in that it can be misdiagnosed as hemophilia A. Type IIN is caused by mutations that decrease the capacity of vWF to bind and stabilize FVIII. These patients will have a prolonged aPTT and low FVIII activity.
of the high-molecular-weight multimers of vWF, which are required for efficient binding to platelets, that causes the observed bleeding phenotype (Fig. 4.16). vWD type IIB is a gain-of-function mutation that causes increased binding to platelet glycoprotein Ib; however, as this binding occurs inappropriately without hemostatic challenge, it leads to a consumption of platelets and the larger WVF multimers.12 As such, treatment of bleeding with deamino-8-D-arginine vasopressin (desmopressin acetate or DDAVP), which releases vWF from endothelial storage sites and thus improves the clinical status in type I vWD, is relatively contraindicated in type IIB owing to the risk of worsening bleeding by increasing the consumption of platelets. VWD IIM is a defect in the ability of vWF to bind platelets. Although both levels of VWF protein and HMW multimers are present, vWF activity is decreased, as measured by platelet aggregation in response to activation with ristocetin. vWD type IIN (Normandy) subtype is distinct in that it can be misdiagnosed as hemophilia A. Type IIN is caused by mutations that decrease the capacity of vWF to bind and stabilize FVIII. These patients will have a prolonged aPTT and low FVIII activity.
Type III vWD, the most severe form, represents an absence of von Willebrand factor and shows no vWF:Ag. Type III vWD is extremely rare and is associated with a severe bleeding phenotype.
In platelet-type or pseudo-vWD, platelet glycoprotein 1b is abnormal and remains in an active form that binds vWF even in the absence of appropriate platelet activation. Pseudo-vWD can be very difficult to distinguish from vWD type IIB in which vWF has increased affinity for platelet gpIb. However, patients with platelet-type vWD will aggregate platelets in the presence of cryoprecipitate in a cryo-challenge test, whereas those with vWD type IIB will not.
Treatment of type I vWD includes avoidance of aspirin and NSAIDs and administration of desmopressin, which releases endogenous vWF from endothelial stores and so raises circulating vWF levels. Specific concentrates of vWF or of factor VIII:vWF are available for severe cases as in type III vWD. More recently recombinant VWF was approved for the on-demand treatment and control of bleeding episodes in adults diagnosed with VWD. Plasma cryoprecipitate, which is rich in vWF, factor XIII, factor XIII, fibrinogen, and fibronectin, can also be used in moderately severe cases, but if used in large volumes may raise fibrinogen and FVIII to potentially hypercoagulable levels.
Hemophilia A and B
Hemophilia is an X-linked recessive disorder of hemostasis that causes a lifelong bleeding tendency. Hemophilia A, or factor VIII deficiency, is present in 1 in 5 of 10,000 male births, whereas hemophilia B, a deficiency of factor IX, is seen in approximately 1 in 30,000 male births. Approximately 30% cases of hemophilia result from spontaneous mutations and thus lack a positive family history. The FVIII gene is extremely large, and many different mutations occur that result in hemophilia A. Occasionally, owing to skewed lyonization, female carriers of hemophilia A can have a bleeding tendency. True hemophilia A in females is extremely rare, comprising the offspring of a hemophilic father and hemophilia carrier mother.
Coagulation factors are normally present in the blood at concentrations far in excess of those needed for normal hemostasis. Therefore, hemophilia is classified as clinically mild (>5% of the normal factor level), moderate (<5% but >1%), or severe (<1%). Patients with hemophilia can present with ecchymosis (spontaneous bruising without preceding trauma) and, most commonly, bleeding into joints (hemarthroses) or soft tissues (Fig. 4.17), which over time and many rebleeding episodes can lead to significant inflammation joint deformity and limited range of motion (Figs. 4.18, 4.19, 4.20 and 4.21). Intracranial bleeding is also a dreaded complication. In severe hemophilia, spontaneous bleeding usually manifests in the 1st year of life (classically at circumcision in the newborn), whereas in moderate or mild hemophilia presentation can be variable. As hemophilia is caused by a defect in the intrinsic pathway, patients have a prolonged aPTT, the finding of which should prompt measurement of factor VIII and/or factor IX levels. Usually, only severe hemophiliacs (factor level <1%) require prophylactic factor replacement. However, patients with moderate hemophilia may still have severe bleeding in response to relatively minor trauma and require factor replacement after injuries or before scheduled surgical procedures13 (Table 4.4). Patients with severe hemophilias may also develop antibodies against factor VIII (or factor IX) after extensive treatment with replacement coagulation factors, leading to further exacerbation of bleeding episodes and difficulty with managing their coagulation defect. Special products such as FEIBA (factor VIII inhibitor bypass activity) and recombinant factor VIIa (rVIIa) have been developed to combat such complications, used together with immunosuppressive therapy to suppress the offending autoantibody.
Hemophilia C: Factor XI Deficiency
Inherited factor XI deficiency is an autosomal bleeding disorder characterized by reduced levels of factor XI in plasma. Factor XI deficiency is seen primarily in Ashkenazi Jewish populations, where up to 1 in 10 individuals may carry a mutation. In contrast to hemophilia A and B, the level of factor XI in this deficiency does not accurately predict the bleeding phenotype because different mutations may have different phenotypes ranging from asymptomatic to severe. Most bleeding manifestations in patients with severe factor XI deficiency are injury related. When a site of injury with high fibrinolysis is involved, the risk of bleeding is increased in comparison with sites without fibrinolysis. This is because factor XI indirectly increases TAFI and thus leads to reduced fibrinolysis and better-stabilized clot formation, and hence deficiency of factor XI is associated with increased fibrinolysis. The main predictor of bleeding in patients with factor XI deficiency is the clinical history of bleeding18 (see Table 4.4). Factor XI has a long half-life, and so administration of fresh frozen plasma as replacement usually suffices for treatment or for surgical prophylaxis.
Rare Factor Deficiencies
Deficiencies of other coagulation factors—including II (fibrinogen), V, VII, X, and XIII—are very rare, occurring in 0.5 to 2 of 1,000,000 individuals (Table 4.5). Factor XII deficiency is not associated with a bleeding phenotype, although the aPTT may be significantly prolonged above normal. Indeed, clinically factor XII deficiency has been linked with thrombosis. For deficiencies of factors VII, X, and XIII, replacement factor concentrates are available, but may not be readily available during an acute bleed. In the absence of specific factor concentrate, prothrombin complex concentrate (PCC), cryoprecipitate, or fresh frozen plasma can be used to replace the relevant missing factor. PCC comes in three-factor (II, IX, and X) and four-factor (II, VII, IX, and X) varieties. The 4PCC contains heparin and should be avoided in patients with heparin-induced thrombocytopenia. Cryoprecipitate, as mentioned above, contains fibrinogen, vWF, factor VIII, factor XIII, and fibronectin. Fresh frozen plasma contains all the clotting factors and is readily available in most clinical settings, although it contains much lower concentrations of factor (by definition, 1 U/ml) than in concentrates and a large volume is needed to control bleeding. Lifelong prophylaxis is useful in prevention of bleeding in patients with congenital afibrinogenemia or factor XIII deficiency.
Acquired Factor Deficiencies
There are many acquired coagulation factor deficiencies, ranging from common (e.g., due to medication such as a vitamin K antagonist) to very rare (e.g., acquired hemophilia). Acquired factor deficiencies are often diagnosed when abnormal coagulation studies are noted as part of the workup of another disorder (Table 4.6) or as an effect of a medication. Warfarin is the classic medication that causes abnormal coagulation studies. Warfarin acts as an indirect anticoagulant by limiting access to vitamin K, the essential cofactor in synthesis of coagulation factors II, VII, IX, and X. Because of warfarin’s slow onset of action and wide variability in dose needed from subject to subject, there is increasing clinical use of direct oral anticoagulants (DOACs), which directly block thrombin or factor X, act rapidly, and do not require monitoring of blood levels. As direct factor inhibitors, the DOACs may alter coagulation studies including PT, aPTT, and thrombin time (TT); however, the effects on these tests are less predictable, nonlinear, and vary between individuals (Table 4.7). The DOACs can also cause a false-positive lupus anticoagulant (LA) test. Therefore, patients taking DOACs should not be tested for the LA unless they have discontinued the anticoagulant.19
Acquired hemophilia A is an autoimmune disease caused by spontaneously arising autoantibodies to endogenous factor VIII.20 In contrast to inherited hemophilia, acquired hemophilia is more likely to present with mucocutaneous bleeding or extensive ecchymosis, rather than deep tissue bleeds (Fig. 4.22). Acquired hemophilia is most common in older men, in whom the development of these autoantibodies is idiopathic, but it can also affect women and younger individuals, in whom it may be associated with pregnancy, autoimmune disease, disturbed immunity, or lymphoma. It is diagnosed by a markedly prolonged aPTT that does not correct on mixing patient plasma with normal plasma, plus identification of a factor VIII inhibitor by the Bethesda assay. The diagnosis is often made in the setting of an acute bleed, which should be managed aggressively with both local control of bleeding with bypassing agents such as FEIBA, recombinant factor VIIa (rVIIa), or porcine factor VIII and immunosuppressive therapy to eradicate the autoantibody with agents such as rituximab or cyclophosphamide in idiopathic cases, and treatment of the underlying condition if that can be identified. An interesting feature of acquired hemophilia A is that once treatment has successfully eliminated the autoantibody, it rarely recurs. Recurrence is more frequent if the autoantibody titer at presentation is very high.
Another mechanism for acquired factor deficiencies is consumption of coagulation factors as in DIC (Table 4.8).21 The many potential causes of DIC include sepsis, trauma, pregnancy, liver failure, and malignancy. Although DIC results in excessive bleeding that can be difficult to control, it is caused by overactivation of thrombotic pathways and is more correctly a hypercoagulable disorder. DIC is a result of inappropriate activation of thrombotic pathways, by extensive exposure to tissue factor in the case of malignancy or trauma, inflammation in the case of sepsis, or inability to synthesize appropriate natural anticoagulants in the case of liver failure. The common features are bleeding or oozing in very ill patients, which should prompt investigation of coagulation studies where fibrinogen will be low, PT and/or aPTT will be elevated, D-dimers will be elevated, hemoglobin and platelets may be low, and review of a peripheral blood smear will show more than one schistocyte per high-power field (HPF). Treatment is supportive with cryoprecipitate to replace fibrinogen that is being consumed, transfusion of platelets, and treatment of the underlying condition that caused DIC, if possible.
HYPERCOAGULABLE STATES
Venous Thrombosis
Venous thrombosis (VT) is a common problem that affects 900,000 persons annually in the United States. In 1856, Virchow described a triad of risk factors for VT that remain key today—interrupted blood flow (stasis), irritation of the vessel (endothelial injury), and enhanced blood coagulation (hypercoagulability) (Fig. 4.23). Deep venous thrombosis (DVT) is often suspected clinically on the basis of unilateral lower extremity edema or redness (Fig. 4.24) and confirmed on ultrasound (Fig. 4.25).
Many common risk factors for VT, including DVT and pulmonary embolism (PE), are listed in Table 4.9. These risk factors can be divided into inherited and acquired. Some patients with VT may have an underlying thrombophilia, placing them at increased risk for thrombosis. Screening
for thrombophilia is indicated in specific clinical scenarios as recommended by published guidelines (Table 4.10).22,23
for thrombophilia is indicated in specific clinical scenarios as recommended by published guidelines (Table 4.10).22,23
Hypercoagulable State and Cancer
A diagnosis of unprovoked VT in an adult should, however, prompt age-appropriate cancer screening. Malignancy, especially gastrointestinal (GI) malignancy such as pancreatic cancer, can promote VT through a variety of mechanisms that are not fully understood currently, which, however, may involve the exposure of tissue factor on tumor and/or neoangiogenic blood vessels. Trousseau syndrome consisting of migratory VT in the setting of abdominal cancer is a classic example of this process. VT associated with cancer is currently best treated with low-molecular-weight heparins (LMWHs), although clinical trials evaluating DOACs versus LMWH are underway. VT in the context of cancer is difficult to treat, especially during chemotherapy, and may progress in spite of appropriate anticoagulation. (Figs. 4.26 and 4.27 show DVT and PE; Well’s criteria for PE are outlined in Table 4.11.24) Some of the ways that malignancy may affect the various hemostatic pathways are outlined in Table 4.12.25
Protein C or S Deficiencies
As can be seen from Figure 4.2, natural anticoagulants have critical roles in regulating and limiting the coagulation cascade and preventing inappropriate thrombosis. Unlike normal coagulation factors, which are normally present in great excess, natural anticoagulants are less abundant so that moderate decreases (e.g., 50% decrease) result in high thrombotic risk. Decreased levels of protein C or S can be inherited or acquired, in the latter case through vitamin K antagonism or depletion (e.g., after surgery). When warfarin administration is initiated, the decrease in protein C occurs before the decrease in vitamin K-dependent coagulation factors because of the very short half-life of protein C, causing a window of hypercoagulability before clinically effective anticoagulation is established. This is the reason that patients need to be “bridged” with a rapidly active anticoagulant such as unfractionated heparin (UFH) or LMWH at the time they are started on a vitamin K antagonist such as warfarin. The rapid decrease in proteins C and S levels is also the mechanism of warfarin-induced skin necrosis, a severe complication of warfarin that can occur in some patients with hereditary protein C and S deficiencies in the absence of proper bridging.
Inherited protein C or S deficiency usually presents as VT and should especially be considered in differential diagnosis of patients with unprovoked VT at a young age, or those with a strong family history of thrombosis, or presenting with an unusual anatomic site of VT such as cerebral VT (Fig. 4.28). While a heterozygote carrier may have variable hypercoagulability, from mild to severe, homozygous deletion of protein C typically presents in utero or shortly after birth with severe purpura fulminans (Fig. 4.29). Homozygous protein C deficiency is fatal unless treated with continuous protein C replacement plus anticoagulation, both of which will be required lifelong.
Antithrombin Deficiency
Antithrombin (AT) is a serine protease synthesized in the liver that inactivates thrombin and factor Xa and, to a lesser extent, factors XIIa, XIa, and IXa, and prekallikrein. Its inhibitory effect is amplified 5,000 to 40,000 fold in the presence of heparin, to which it binds. The half-life of AT is approximately 2.4 days. The gene coding for antithrombin is located at chromosome 1q23-25. Inherited AT deficiency, an autosomal-dominant trait with variable clinical penetrance, is a major risk factor for venous thromboembolism (VTE) and was the first identified inherited thrombophilia.26 Approximately 50% to 90% of untreated heterozygote carriers develop VTE during their lifetime. The estimated prevalence of heterozygote AT deficiency in the general population is between 1 in 500 and 1 in 5,000. Two major types of inherited AT deficiency have been identified. In type I AT deficiency, the AT antigen and activity levels are similarly reduced, whereas in type II, the AT activity is reduced but antigen levels are normal. These two types of AT deficiency in the heterozygous state are associated with approximately 50% of normal AT activity and are estimated to increase by about 20-fold the risk of developing a first episode of VTE. Another variant of AT deficiency contains a defective heparin-binding site; it is about five times as common as type I and type II deficiencies combined, but has approximately one-fifth their risk of VTE.27
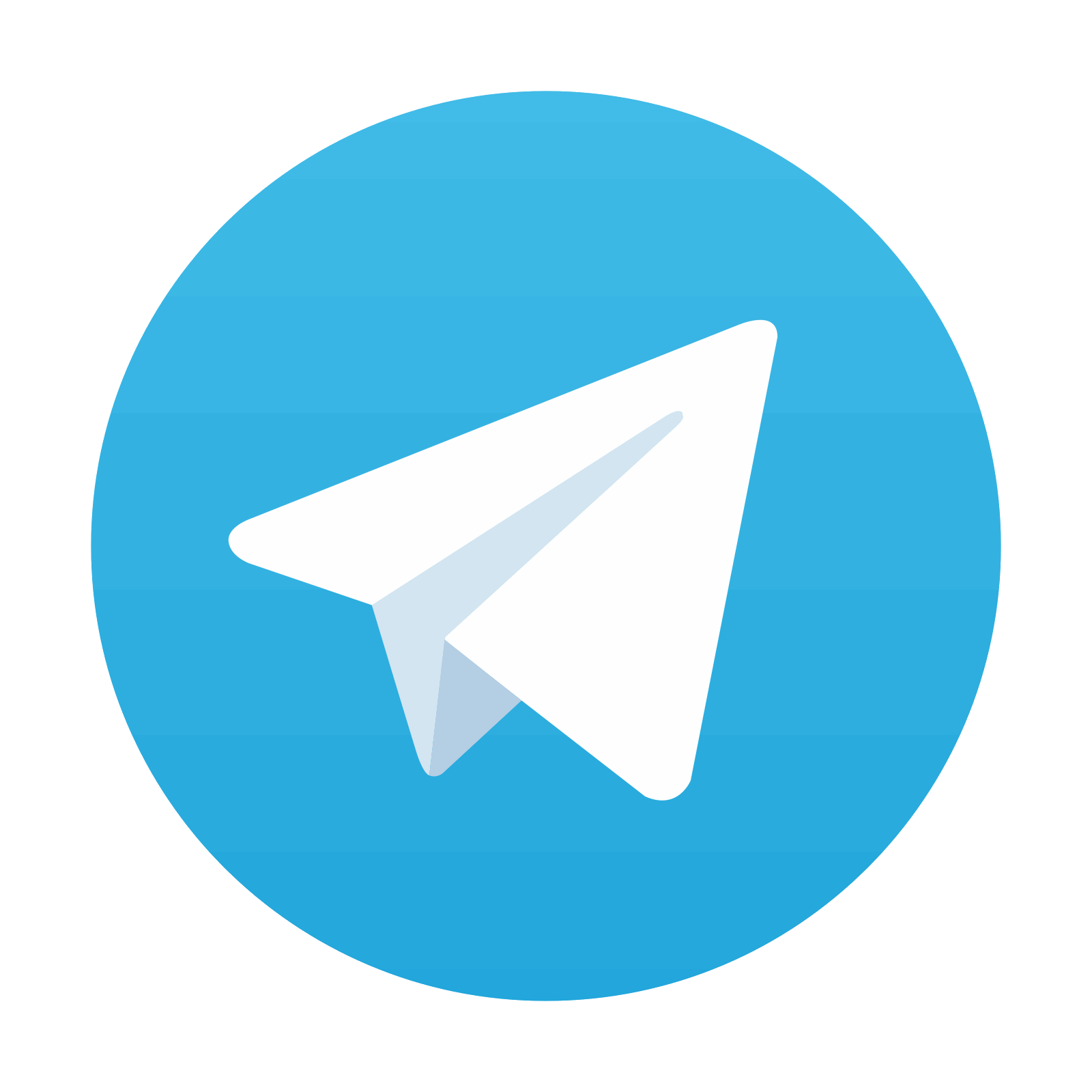
Stay updated, free articles. Join our Telegram channel

Full access? Get Clinical Tree
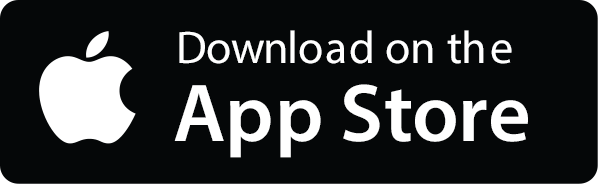
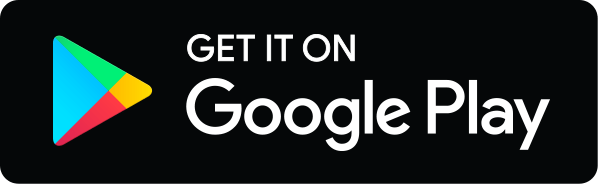
