Hematopoietic Cytokines and Growth Factors
Hematopoietic homeostasis is tightly controlled by a group of hematopoietic cytokines ( Fig. 2.1 ). Each cytokine performs unique and/or multiple actions mediated by receptors whose cytoplasmic domains contain specialized regions initiating their various responses, including survival, proliferation, apoptosis, differentiation commitment, maturation, and functional activation. Cytokines are protein molecules and, when regulating cell growth, they are also termed growth factors. Individual cytokines can be lineage specific or can regulate cells in multiple lineages; for some cell types, such as hematopoietic stem cells (HSCs), progenitors, and precursors, the simultaneous action of multiple factors and cytokines is required for proliferative responses. These signals also affect the functions of mature cells and are involved in boosting the production of leukocytes in response to infection, red blood cells in response to anemia, and platelets in response to thrombocytopenia. Cytokines or growth factors are released systemically into the bloodstream not only by organs (e.g., the kidneys release erythropoietin [EPO] and the liver produces thrombopoietin [TPO]), but also by other bone marrow cells, such as stromal cells (fibroblasts and endothelial cells), lymphocytes, and macrophages. Hematopoietic cytokines include colony-stimulating factors (CSFs), interleukins (ILs), monokines, chemokines, and interferons (IFNs). Signaling molecules can also act more locally—for example, as in the confines of the bone marrow. These molecules may also target cells at long range or just affect target cells nearby (paracrine)—for example, within a local niche (see Chapter 1 ). In some cases, paracrine signaling requires cell-to-cell contact. Finally, a cell may release signaling molecules that it can in turn detect to affect its own behavior (autocrine signaling).

Through the 1940s and 1950s, increasing evidence from experimental animals demonstrated that a humoral regulator of red blood cell formation, termed EPO, existed and was elevated in anemic plasma and urine. Today this cytokine, EPO, along with granulocyte colony-stimulating factor (G-CSF) and granulocyte-macrophage colony-stimulating factor (GM-CSF), are in routine clinical use in patients to stimulate erythrocyte production therapeutically in bone marrow transplantation and neutrophil production in the treatment of congenital and acquired cytopenias. The effects of these cytokines depend on their interaction with the signaling ligands that bind them to their respective cell receptors. In this chapter, we review the biology of these signaling ligands, the receptors with which they interact, and aspects of how these signals are transduced by cells.
Signaling at Different Stages of Hematopoiesis
The secreted cytokines are produced in response to developmental and/or environmental cues and act via cognate cytokine receptors on target cells, stimulating specific intracellular signaling pathways to facilitate appropriate cellular responses. It is possible to identify which cytokines, growth factors, and local signaling molecules function during the different stages of hematopoiesis by documenting the different complements of cell surface receptors that detect and respond to external signaling molecules in hematopoietic stem and progenitor cells and mature blood cells (see Fig. 2.1 ). In vitro culture systems have shown that, although some cytokines support specific lineages, others affect multiple lineages. This has led to the notion that, like hematopoiesis itself, signaling molecules can be arranged in a hierarchical manner. Some, such as stem cell factor (SCF, which binds the receptor c-Kit), Fms (feline McDonough sarcoma)-like tyrosine kinase 3 (FLT3), TPO, and IL-3, act on hematopoietic stem and progenitor cells (HSPCs). Others have a more restricted action and act on specific lineages, although they may also affect earlier cells. These cytokines include EPO, which functions on erythroid cells; G-CSF, which is required for neutrophils; GM-CSF, which promotes granulocyte and monocyte production; IL-5, which is a central cytokine for eosinophils; and IL-4, INF-γ, and IL-7, which are required for lymphocytes. , Fig. 2.2 illustrates the action of different cytokines and signaling molecules on all the different cell intermediates in granulopoiesis, erythropoiesis, megakaryopoiesis, and lymphopoiesis.

Over time, and with increasingly refined analysis of genetically modified animal models, it has become apparent that cytokines such as TPO, G-CSF, and GM-CSF can regulate HSPCs as well as lineage-specific cells. TPO is a good example of a cytokine that has been known to act on multiple cell types and promote HSC growth, but it also has a critical role in megakaryocyte proliferation and maturation. Also, the extrinsic administration of G-CSF, a key cytokine promoting granulopoiesis, facilitates the mobilization of HSPCs from the bone marrow to peripheral blood, , where they can be collected by apheresis and used in HSC transplantations for the treatment of hematologic disorders. In addition to the study of genetically modified mice, improvements in the in vitro HSC culture system have produced powerful tools to elucidate the functions of cytokines that regulate HSC function. During the past decade, stromal cell lines have been established from in vivo fetal or adult hematopoietic organs, including aorta-gonad mesonephros (AGM), fetal liver, yolk sac, and bone marrow. Extensive efforts have led to the successful isolation of secreted proteins from these HSC-supportive stromal cell lines. Examples of these secreted proteins include insulin-like growth factor 2 (IGF-2) and a group of angiopoietin-like proteins (Angptls), which were identified by transcriptional profiling of the mouse fetal liver; CD3 + Ter119 − mouse fetal liver cells, as well as IGF binding protein 2 (IGFBP2), a protein secreted by HEK 293T cells, and angiopoietin-like 5, support HSC expansion. A serum-free culture containing SCF, TPO, fibroblast growth factor 1 (FGF), angiopoietin-like 5, and IGF binding protein 2 (IGFBP2) has been shown to support an approximately 20-fold net expansion of repopulating human cord blood HSCs. These recent advances in the identification of novel HSC supportive cytokines have further revealed the complex nature of the mechanisms by which extrinsic factors regulate the signal transduction and cell fate decisions of HSCs.
The signaling interplay between hematopoietic cells and surrounding cells in their shared microenvironment is also complex. Endotoxin stimulates monocytes to release IL-1 and tumor necrosis factor (TNF), which in turn induces marrow stromal cells (fibroblasts, endothelial cells), as well as T lymphocytes and macrophages, to produce a variety of cytokines. Blood oxygen tension is sensed by mesangial cells in the kidney and, to a lesser extent, by hepatocytes. At high-oxygen tension, a family of oxygen-sensitive prolyl hydroxylase enzymes hydroxylates the transcription factor hypoxia-inducing factor 1α (HIF-1α) on prolyl residues (see Fig. 2.2 ). This leads to ubiquitination of HIF-1α by a ubiquitin ligase complex that contains the tumor suppressor known as von Hippel-Lindau protein. Ubiquitinated HIF-1α is then degraded. Lower levels of HIF-1α are known to lead to lower transcription of EPO in renal mesangial cells, indicating that there is autocrine/paracrine regulation of EPO levels in the kidneys. In contrast, in response to the hypoxia caused—for example, by anemia or high altitude—prolyl hydroxylases are inhibited and HIF-1α levels are high, which upregulates EPO production. EPO stimulates erythropoiesis largely at the committed colony-forming unit-erythroid (CFU-E) stage; also, a proportion of burst-forming unit-erythroid (BFU-E) progenitors and more mature cells (up to the reticulocyte stage) are sensitive to EPO. The action of EPO increases the overall red blood cell mass, leading to increased hemoglobin.
Some of these cytokines likely have biological effects outside the process of hematopoiesis. For example, EPO is thought to protect central nervous system neurons against hypoxic stress. The pleiotropic effects of cytokines are likely caused by the level of cell-specific cytokine receptor expression, the availability of cytokines, synergistic interactions between cytokines, and the differential attenuation of cytokine signals in a cell type–specific manner by negative regulators such as the suppressor of cytokine signaling proteins. Recent studies have shown cytokines are active in the bone marrow niche for myeloid progenitors and indicate a link to leukemic myelopoiesis. The study by Hérault et al. revealed the presence of cyclic guanosine monophosphate (cGMP) cluster formation in the bone marrow microenvironment during regenerative and leukemic myelopoiesis. Using the same immunofluorescence staining previously established for flow cytometry, individually scattered GMPs in situ in the bone marrow (BM) microenvironment were observed under steady state, seemingly without a specific distribution pattern. These scattered GMPs undergo clonal expansion and form large compact patches surrounded by Lin + cells with defined boundaries in both regenerative and leukemic myelopoiesis models. G-CSF and IL-1 promoted cGMP formation, whereas megakaryocytes prompted HSCs to return to quiescence and inhibited prolonged cGMP formation. These opposing signals from transforming growth factor β and CXCL4 are among the signals from the microenvironment that regulate cGMP formation. Thus cytokines represent essential mediators of cell-cell communication that play particularly important roles within the hematopoietic system through precise regulation of signaling at each step of hematopoietic lineages.
Cytokine Receptors
Hematopoietic growth factors display the common 4-α-helical bundle structure, which has the ability to promote the proliferation and differentiation of hematopoietic progenitors derived from HSCs in hematopoietic lineages. Hematopoietic growth factors are classified into two groups: intermediate-acting lineage-nonspecific factors and late-acting lineage-specific factors.
Cytokines bind to their receptors with high picomolar affinity leading to either receptor homodimerization (e.g., EPO receptor [EPOR]), heterodimerization/oligomerization of receptor subunits (e.g., IL-2 receptor), or induction of a conformational change in preformed receptor dimers (EPOR) resulting in the activation of downstream signaling pathways. The cytokine receptor superfamily is composed of more than 20 members and is characterized by structural motifs in the exoplasmic domain and by the absence of catalytic activity in the cytosolic segment. Many cytokine receptors are lineage restricted. An example of such a receptor is the EPOR, which was the first cytokine receptor to be cloned, and is not expressed on HSCs or immediate descendants. The expression of the EPOR can be detected on committed BFU-E progenitors and on the surface of their progenies as well as in megakaryocytic progenitors. BFU-Es require a variety of growth factors for survival and proliferation, such IL-3, GM-CSF, and Kit ligand, and EPO can stimulate the proliferation and size of the bursts. The later committed progenitor CFU-E absolutely requires EPO to survive, proliferate, and differentiate into mature red blood cells. Epo – or Epor -knockout embryos die at embryo day 12.5 owing to severe anemia. , Interestingly, in such embryos, a normal number of erythroid progenitors (BFU-E and CFU-E) are exhibited at the level of the fetal liver. Alhough Epo and Epor are dispensable for erythroid lineage commitment, it has been well shown that both genes are crucial in vivo for the proliferation, survival, and terminal differentiation of CFU-E progenitors. Interestingly, EPOR expression is lost after the basophilic stage, suggesting that once the EPOR receptor transmits its signal, the intrinsic erythroid differentiation program unfolds irrespective of the receptor’s presence. Infection of adult mice with a retrovirus coding for the constitutively active EPOR resulted in proliferation and contributed to the transformation of multipotent, committed nonerythroid and erythroid progenitor cells. In addition to polycythemia and an increase in megakaryocytes, these mice exhibited leukemias and lymphomas of different lineages, suggesting that EPOR signals in all blood lineages.
Cytokine receptors are divided into several families ( Fig. 2.3A ) and can be composed of dimers of a single receptor chain. Examples include EPOR, thrombopoietin receptor (TPOR), or myeloproliferative leukemia (c-MPL) protein, and G-CSFR. TPOR activates many of the same pathways as EPOR (JAK2, signal transducer and activator of transcript 5, mitogen-activated protein kinase, phosphatidylinositol 3 kinase). TPOR is expressed on HSCs and early descendants and, as described earlier, serves as both a lineage-specific growth factor and a regulator of HSCs, thus affecting all blood lineages. As the receptor for TPO that promotes megakaryocyte progenitor expansion and differentiation, TPO has been shown to support the maintenance of HSC capacity in cultures of purified HSCs. Knockout mice in which the genes coding for Tpo or Tpor were deleted showed a profound reduction not only in megakaryocytes and platelets, but also in committed myeloid progenitors of all types, primitive progenitors, and HSCs.

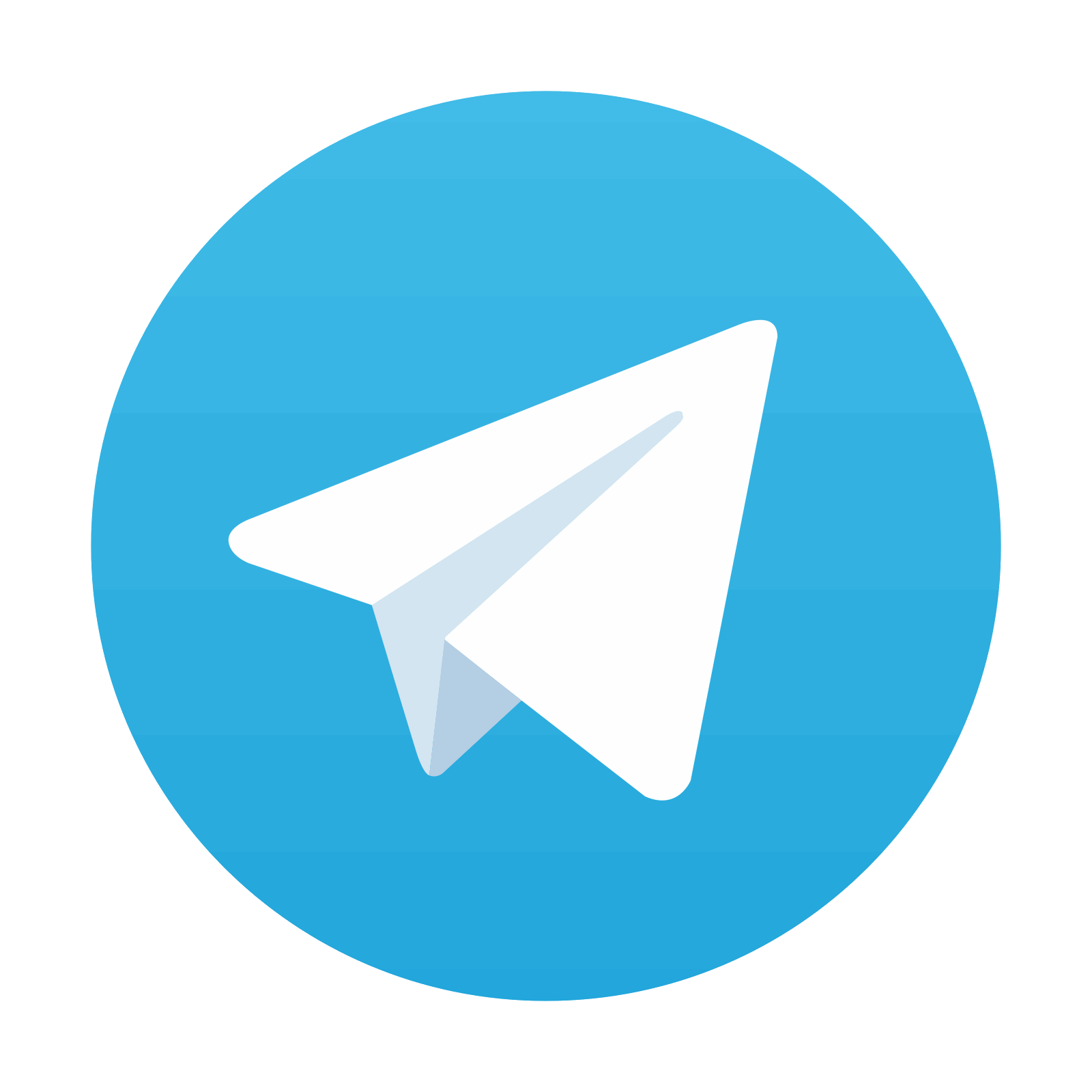
Stay updated, free articles. Join our Telegram channel

Full access? Get Clinical Tree
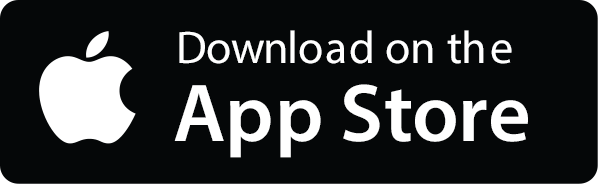
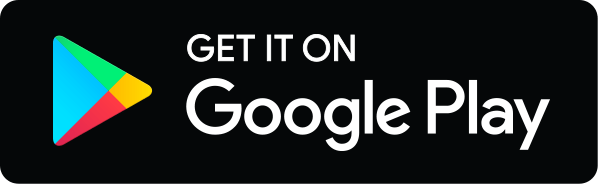
