Melanoma is increasing in incidence and represents an aggressive type of cancer. Efforts have focused on identifying genetic factors in melanoma carcinogenesis to guide prevention, screening, early detection, and targeted therapy. This article reviews the hereditary risk factors associated with melanoma and the known molecular pathways and genetic mutations associated with this disease. This article also explores the controversies associated with genetic testing and the latest advances in identifying genetic targets in melanoma, which offer promise for future application in the multidisciplinary management of melanoma.
Key points
- •
The genetic heterogeneity of melanoma has been a challenge to identifying genetic targets for prognosis and screening.
- •
BRAF mutation analysis has become a standard approach to the management of metastatic melanoma.
- •
The role of genetic screening in the management of melanoma is controversial.
Introduction
Clinical and Epidemiologic Overview
Skin cancer is one of the fastest growing cancer diagnoses in the United States. The lifetime risk for the general population of developing melanoma is 1 in 55 and that risk has increased approximately 2% annually since 1960. Because of the association with sun exposure, efforts to reduce the incidence of melanoma have focused on educating the public on risk modification strategies to improve sun safety. However, despite these efforts, the incidence of skin cancer in general and melanoma specifically have continued to increase, especially in Western countries. Although a personal history of sun exposure and sunburns is the most important risk factor, a family history of melanoma and previous personal history of skin cancers have also been used as criteria to help guide screening. There have been efforts to identify patients who have a genetic predisposition to be at increased risk for skin cancer. There has been an increased interest in understanding the genetic components of melanoma carcinogenesis and how that influences risk in patients with melanoma to better guide screening, surveillance, and clinical management of this disease. This article reviews the hereditary factors associated with melanoma and the role of genetic testing in the multidisciplinary management of melanoma.
Risk Factors
Although genetic factors have been identified for melanoma, only 10% or less of all melanoma cases arise in families with clusters of melanoma. Many of these families do not have specific genotypic risk factors identified for melanoma, and a significant fraction of these families harbor no detectable mutations in specific susceptibility genes. For example, although CDKN2A is a gene that may indicate an increased risk of melanoma, several studies have failed to demonstrate a population-wide correlation with actually developing melanoma. The link between skin color and melanoma has been applied to study the prevalence of melanoma in ethnic groups. Although ethnic groups with a darker complexion have a lower melanoma risk, melanoma in such individuals more commonly presents in the palms, nail beds, and soles. In individuals with a lighter complexion, skin color is determined by a combination of behavior and genetics, including the MC1R pigment-controlling gene.
A positive melanoma family history has been correlated with an increased individual risk of developing melanoma, and as such, population-based studies have been conducted to focus on families with clusters of melanoma cases. Studies have reported a nearly 3 times increased risk of melanoma in individuals with a family history of melanoma, a lifetime risk of nearly 3%, and even as high as 14% when 2 or more individuals were diagnosed by age 30 years in a single family. In addition, the risk was even higher when the population had a high frequency of sun exposure. Nearly 40% of families with at least 3 melanoma cases demonstrated mutations in the major hereditary melanoma susceptibility gene, CDKN2A; however, more than 50% of such high-risk families demonstrate no known genetic mutation. Because of these findings, it is recommended that genetic counseling be sought for patients with (1) 3 or more primary melanomas, (2) melanoma and pancreatic cancer, (3) melanoma and astrocytoma, or (4) at least 3 cases of melanoma or pancreatic cancer in a first-degree relative or with 2 first-degree relatives with melanoma and astrocytoma.
When assessing an individual’s risk of developing additional melanoma primary lesions, factors such as skin type, family history, CDKN2A mutation, and sun exposure all play a role. For example, a family history has been shown to increase the risk of a subsequent primary melanoma from 5% to 30%. Similarly, if a patient has a history of nonmelanoma skin cancer, the risk of developing melanoma is also increased, which is thought to be due to a combination of genetic and environment risk factors. Although the exact genetic mechanisms remain under investigation, it is established that patients with a family history of melanoma should be actively screened for melanoma. The following sections review the genetic mechanisms under investigation in melanoma and provide an update on the status of genetic testing in the multidisciplinary management of melanoma ( Table 1 ).
Gene | Location | Function | Application in Melanoma |
---|---|---|---|
N-RAS | 1p13-p11 Somatic mutation | Encodes NRAS, cell cycle regulation | Under investigation |
H-RAS | 11p15.5 Somatic mutation | Encodes HRAS, transforming protein p21, cell cycle regulation | Under investigation |
BRAF | 7q34 Somatic mutation | Encodes BRAF, cell cycle regulation | Selection of patients for targeted therapy |
CDKN2A | 9p21.3 Germline mutation | Encodes CDKN2A, p16, p14ARF, cell cycle regulation | Selection of patients for closer screening and surveillance |
TERT | 10p15 Germline and somatic mutations | Encodes hTERT, regulation of telomerase and cellular senescence | Under investigation |
POT1 | 7q31.33 Germline mutation | Encodes POT1, regulation of telomerase and cellular senescence | Under investigation |
CDK4 | 12q14.1 Germline and somatic mutations | Encodes CDK4, cell cycle regulation | Under investigation |
BAP1 | 3p12.31-p21.2 Germline and somatic mutations | Encodes BAP1, regulates DNA repair | Under investigation |
PTEN | 10q23.31 Germline mutation | Encodes PTEN, cell cycle regulation | Under investigation |
MC1R | 16q24.3 Somatic mutation | Encodes MC1R, encodes skin and hair pigment | Under investigation |
MITF | 3p14-p13 Germline and somatic mutations | Encodes MITF, regulates melanocyte development | Under investigation |
BRCA2 | 13q12.3 Germline mutation | Encodes BRCA2, regulates DNA repair | Under investigation |
Introduction
Clinical and Epidemiologic Overview
Skin cancer is one of the fastest growing cancer diagnoses in the United States. The lifetime risk for the general population of developing melanoma is 1 in 55 and that risk has increased approximately 2% annually since 1960. Because of the association with sun exposure, efforts to reduce the incidence of melanoma have focused on educating the public on risk modification strategies to improve sun safety. However, despite these efforts, the incidence of skin cancer in general and melanoma specifically have continued to increase, especially in Western countries. Although a personal history of sun exposure and sunburns is the most important risk factor, a family history of melanoma and previous personal history of skin cancers have also been used as criteria to help guide screening. There have been efforts to identify patients who have a genetic predisposition to be at increased risk for skin cancer. There has been an increased interest in understanding the genetic components of melanoma carcinogenesis and how that influences risk in patients with melanoma to better guide screening, surveillance, and clinical management of this disease. This article reviews the hereditary factors associated with melanoma and the role of genetic testing in the multidisciplinary management of melanoma.
Risk Factors
Although genetic factors have been identified for melanoma, only 10% or less of all melanoma cases arise in families with clusters of melanoma. Many of these families do not have specific genotypic risk factors identified for melanoma, and a significant fraction of these families harbor no detectable mutations in specific susceptibility genes. For example, although CDKN2A is a gene that may indicate an increased risk of melanoma, several studies have failed to demonstrate a population-wide correlation with actually developing melanoma. The link between skin color and melanoma has been applied to study the prevalence of melanoma in ethnic groups. Although ethnic groups with a darker complexion have a lower melanoma risk, melanoma in such individuals more commonly presents in the palms, nail beds, and soles. In individuals with a lighter complexion, skin color is determined by a combination of behavior and genetics, including the MC1R pigment-controlling gene.
A positive melanoma family history has been correlated with an increased individual risk of developing melanoma, and as such, population-based studies have been conducted to focus on families with clusters of melanoma cases. Studies have reported a nearly 3 times increased risk of melanoma in individuals with a family history of melanoma, a lifetime risk of nearly 3%, and even as high as 14% when 2 or more individuals were diagnosed by age 30 years in a single family. In addition, the risk was even higher when the population had a high frequency of sun exposure. Nearly 40% of families with at least 3 melanoma cases demonstrated mutations in the major hereditary melanoma susceptibility gene, CDKN2A; however, more than 50% of such high-risk families demonstrate no known genetic mutation. Because of these findings, it is recommended that genetic counseling be sought for patients with (1) 3 or more primary melanomas, (2) melanoma and pancreatic cancer, (3) melanoma and astrocytoma, or (4) at least 3 cases of melanoma or pancreatic cancer in a first-degree relative or with 2 first-degree relatives with melanoma and astrocytoma.
When assessing an individual’s risk of developing additional melanoma primary lesions, factors such as skin type, family history, CDKN2A mutation, and sun exposure all play a role. For example, a family history has been shown to increase the risk of a subsequent primary melanoma from 5% to 30%. Similarly, if a patient has a history of nonmelanoma skin cancer, the risk of developing melanoma is also increased, which is thought to be due to a combination of genetic and environment risk factors. Although the exact genetic mechanisms remain under investigation, it is established that patients with a family history of melanoma should be actively screened for melanoma. The following sections review the genetic mechanisms under investigation in melanoma and provide an update on the status of genetic testing in the multidisciplinary management of melanoma ( Table 1 ).
Gene | Location | Function | Application in Melanoma |
---|---|---|---|
N-RAS | 1p13-p11 Somatic mutation | Encodes NRAS, cell cycle regulation | Under investigation |
H-RAS | 11p15.5 Somatic mutation | Encodes HRAS, transforming protein p21, cell cycle regulation | Under investigation |
BRAF | 7q34 Somatic mutation | Encodes BRAF, cell cycle regulation | Selection of patients for targeted therapy |
CDKN2A | 9p21.3 Germline mutation | Encodes CDKN2A, p16, p14ARF, cell cycle regulation | Selection of patients for closer screening and surveillance |
TERT | 10p15 Germline and somatic mutations | Encodes hTERT, regulation of telomerase and cellular senescence | Under investigation |
POT1 | 7q31.33 Germline mutation | Encodes POT1, regulation of telomerase and cellular senescence | Under investigation |
CDK4 | 12q14.1 Germline and somatic mutations | Encodes CDK4, cell cycle regulation | Under investigation |
BAP1 | 3p12.31-p21.2 Germline and somatic mutations | Encodes BAP1, regulates DNA repair | Under investigation |
PTEN | 10q23.31 Germline mutation | Encodes PTEN, cell cycle regulation | Under investigation |
MC1R | 16q24.3 Somatic mutation | Encodes MC1R, encodes skin and hair pigment | Under investigation |
MITF | 3p14-p13 Germline and somatic mutations | Encodes MITF, regulates melanocyte development | Under investigation |
BRCA2 | 13q12.3 Germline mutation | Encodes BRCA2, regulates DNA repair | Under investigation |
Somatic genetic alterations associated with melanoma carcinogenesis
Tyrosine Kinases
An important class of proteins to consider in understanding the carcinogenesis of melanoma includes the receptor tyrosine kinases (TKs), which have been implicated in the carcinogenesis of many tumor types. TKs, which are postulated to play a role in the development of melanoma, include the epidermal growth factor receptor (EGFR), Met receptor tyrosine kinase (c-MET), and Kit receptor TK (c-KIT). Although EGFR activation can result in further activation of the mitogen-activated protein kinase and phosphoinositide 3-kinase (PI3K) signaling cascades, initial studies in melanoma have not demonstrated overexpression of EGFR. However, there are data from melanoma cell lines that targeting the EGFR-RAS signaling pathway may have potential as a therapeutic target in melanoma. c-MET has been shown to be overexpressed in metastatic melanoma and promote invasiveness in in vitro and in vivo animal models, and there are investigations underway to further assess its role as a targeted therapy. c-KIT has been implicated in activating numerous signaling pathways, such as PI3K and RAS/ERK. Similar to c-MET, there are in vitro and in vivo animal model data in melanoma to support the role of c-KIT in cancer progression, invasiveness, and survival. In addition, the TK inhibitor imatinib may be an effective agent in treating melanoma, as case reports in rectal and anal mucosal melanoma demonstrated KIT overexpression and promising therapeutic responses to treatment, but further studies are still required. Although these preliminary results are promising, the exact role of TKs in melanoma remains under investigation, and thus, the application to clinical management is not yet established.
RAS/RAF/MEK/ERK and Phosphoinositide 3-Kinase Pathways
Of the receptor TK-associated pathways in cancer, the most promising pathways for clinical application in melanoma have been the RAS/RAF/MEK/ERK and the PI3K pathways. The most common TK mutation found in melanoma is N-RAS, which is seen in approximately one-third of primary melanomas and about one-fourth of metastatic lesions, yet these mutations are rarely present in benign skin lesions such as nevi. Although H-RAS mutations have been identified in Spitz nevi, these lesions do not transform into melanoma, and the application to atypical Spitz nevi, including in cases where they may transform into melanoma, remains controversial. The precise role of the different RAS mutations in melanoma carcinogenesis remains unclear and is a focus of research. In contrast, the RAF family plays an important role in melanoma, and advances in research have already translated into a major impact on the management of melanoma with targeted therapy, as described in the following.
The RAF family consists of ARAF, BRAF, and CRAF; however, thus far BRAF mutations seem to be the most clinically relevant in melanoma. In fact, BRAF mutations have been identified in a variety of tumor cell lines, the highest occurrence in melanoma. Sixty-seven percent of sequenced melanoma tumor samples demonstrated a mutation in BRAF. An amino acid substitution (V600E) was, by far, the most common mutation both in cell lines and tumor samples. Alterations in BRAF may be an early somatic event (see later), as germline mutations in BRAF are not commonly found in familial melanoma. However, studies of benign cutaneous lesions and dysplastic nevi have identified the presence of this mutation, even though such lesions do not often progress to melanoma. Although BRAF is thought to be a mechanism of inhibiting the progression of premalignant lesions to melanoma by inducing senescence, investigations are underway to clarify this mechanism. In fact, the BRAF pathway has not been shown to promote melanoma carcinogenesis without failures in other pathways, such as p53 deficiency or changes in N-RAS expression. Although BRAF V600E mutated melanoma is more likely to arise in less sun-exposed areas of the body, N-RAS mutated melanoma is more likely present in more sun-exposed areas, with significant differences between the two in genetic signatures of these 2 melanoma types. The ERK pathway is another important factor under investigation, especially because in NRAS cells ERK activation is low in contrast to BRAF mutated melanoma. The PI3K pathway has been implicated as an important factor in determining survival in melanoma because of its activation of AKT, which is inversely related to patient survival. AKT3 activation has been identified in nearly two-thirds of sporadic melanoma samples; its role in cancer progression has been demonstrated in many in vitro and in vivo animal studies, whereas studies are underway to clarify its relevance to melanoma.
Although the specific mechanisms of the role of BRAF in melanoma carcinogenesis and biology remain under investigation, BRAF regulation of melanoma cell survival has emerged as a target for therapy. The BRAF kinase inhibitor vemurafenib demonstrated improved overall and progression-free survival in stage IIIC and IV melanoma. Accordingly, the US Food and Drug Administration (FDA) granted approval in 2011 for the use of vemurafenib in patients with BRAF V600E mutated unresectable metastatic melanoma. A second agent targeting BRAF has demonstrated promise. Dabrafenib, a selective BRAF kinase inhibitor, demonstrated improved progression-free survival in an international multi-institutional crossover study not designed to demonstrate an overall survival benefit. The FDA in 2014 granted approval for the use of dabrafenib in the treatment of BRAF V600E mutated unresectable metastatic melanoma.
Germline (hereditary) genetic alterations associated with melanoma predisposition
CDKN2A (P16)
Of all the candidates for a genetic test to assess the risk of developing melanoma in the general population, CDKN2A has demonstrated the most promise (see Table 1 ). CDKN2A/p16 is a cyclin-dependent kinase inhibitor 2A located on chromosome 9p21. It operates through the cyclin D1/cyclin-dependent kinase 4 complex to regulate the retinoblastoma gene pathway functioning as a tumor suppressor, controlling cell cycle progression, and blocking progression at G1/S for DNA damage repair. It encodes p16INK4a and p14ARF, proteins that inhibit cellular senescence. When the standard reading frame protein is not encoded, the exon 1 alternate reading frame (ARF) functions through the p53 pathway, which normally causes cell cycle arrest supporting blockade at G1/S and allows for DNA repair to thus prevent carcinogenesis. Taken together, the disruption of this pathway results in failure in tumor suppression and is actually accountable for 40% of all familial melanoma cases.
Despite the prevalence of CDKN2A mutations in cases of familial melanoma, the penetrance of the melanoma phenotype in carriers of CDKN2A mutations has varied in the literature. Although in Australia the penetrance has ranged from 30% to 91%, this contrasts to the United States (50%–76%) and Europe (13%–58%). However, there was no difference in the cumulative risk of melanoma in CDKN2A mutation–positive families in Australia versus those in the United Kingdom. Because of this variable penetrance, other genetic factors have been investigated as modifiers of the risk of developing melanoma in CDKN2A mutation carriers. For example, the melanocortin 1 receptor (MC1R), a somatic mutation, has been found to augment CDKN2A penetrance, and other genetic factors such as interleukin 9 (IL-9) and GSTT1, both somatic modifiers, have also been found to affect the risk of developing melanoma. In addition to these identified genetic factors, it has been shown that CDKN2A mutation carriers who develop melanoma do so at a younger age than patients with CDKN2A wild-type melanoma, at a median age of 39 versus 54.3 years, respectively.
Greater Breslow thickness was also found to correlate with the presence of a CDKN2A mutation; a 90% chance of the mutation was demonstrated in cases greater than 0.4 mm thick when investigating cases of melanoma in high cluster families (defined as at least 3 cases per family). Patients with the CDKN2A mutation also had increased risks of pancreatic, breast, gastrointestinal, and lung cancers and Wilms tumor, suggesting additional genetic modifiers of the cancer phenotype in CDKN2A mutation carriers remain to be identified. Although more specific CDKN2A variants have been identified, such as the CDKN2A exon 1ß mutations (p14ARF), which demonstrated increased melanoma risk compared with individuals without the p16INK4a mutation, there is no commercially available genetic test for this mutation at this time. Accordingly, studies have evaluated potential candidates for genetic testing in CDKN2A carriers to assess for the risk of other malignancies and hereditary syndromes. However, specific candidates have not yet been firmly established as appropriate for genetic testing.
Investigations of families with CDKN2A germline mutations indicate an increased incidence of pancreatic cancer and neural system tumors within certain subpopulations. Specifically, in families with CDKN2A pathologic mutations that take the form of a so-called ankyrin repeat, there was a higher incidence of family clusters with both melanoma and pancreatic cancer cases rather than melanoma alone. Families carrying a deletion CDKN2A exon 2 mutation (p16 Leiden) were also found to be at increased risk of melanoma and pancreatic cancer, in contrast to melanoma cluster families without that specific CDKN2A mutation that did not demonstrate an increased pancreatic cancer incidence. However, other studies have not demonstrated an increased risk of pancreatic cancer in CDKN2A mutation families, and some CDKN2A mutation families demonstrated pancreatic cancer risk only. Therefore, it is thought that other genetic factors may be contributing to the link between CDKN2A mutation and the phenotype of melanoma and pancreatic cancer. Some CDKN2A mutation carriers also demonstrate the phenotype of the melanoma-astrocytoma syndrome. The hallmark of this syndrome is the presence of melanoma in addition to tumors of the nervous system. This syndrome was only identified in the 1990s when individuals in high cluster melanoma case families were also found to have nervous system tumors, including neural cell tumors. When these individuals were studied further, there was a loss of function in p14ARF, an ARF protein product of CDKN2A, strongly suggesting a link to CDKN2A.
CDKN2A has been widely studied as a candidate gene for melanoma. Its mechanism through a cyclin-dependent kinase as a tumor suppressor has been established for oncogenesis in multiple studies. In addition, there have been some promising results that have demonstrated a link between CDKN2A mutations and a risk for developing cancer, including melanoma. However, these results have not been validated sufficiently to translate into a specific genetic test that assesses the risk of developing these phenotypes in the general population.
Telomeres
Telomeres are another important area of inquiry in the genetic understanding of melanoma carcinogenesis. Mutations in the telomerase reverse transcriptase (TERT) gene, on chromosome 5p, have been demonstrated in individuals with familial melanoma and other cancers such as bronchial, renal, ovarian, and bladder cancers, as well as in cases of sporadic melanoma. The prevalence of TERT mutations in families with multiple melanoma cases is under investigation. Another telomere-associated gene has been implicated in melanoma; POT1 maintains telomere length by binding single-stranded repeats. Studies in multiple melanoma case families demonstrated correlations with POT1 missense mutations, including Ser270Asn, Tyr89Cys, Arg137His, and GIn623His. Supporting this mechanism is the finding of longer telomeres in POT1 mutated melanoma cases than in nonmutated cases. Although these mutations were identified in 4% of non-CDKN2A melanoma families, the results have not been sufficiently validated to allow development of a genetic test that could be used to assess the risk of developing melanoma or to affect treatment.
Cyclin-Dependent Kinases
Another important candidate for melanoma genetic testing includes cyclin-dependent kinases, which regulate cell cycle progression and function in concert with CDK4 and 6. Although cyclin-dependent retinoblastoma protein phosphorylation results in changes in gene expression along the same pathway as CDKN2A, CDK4 mutations are not prevalent in sequenced melanoma samples. Although families bearing CDK4 mutations demonstrated melanoma risk similar to that of CDKN2A families, CDK6 mutations have not been found in patients with melanoma. It has also been demonstrated that patients with xeroderma pigmentosum, which results from defects in DNA repair, have a 1000 times increased likelihood of developing melanoma compared with the general population. Another cyclin-dependent kinase–regulated tumor suppressor gene, BRCA-associated protein 1 (BAP1), was demonstrated as a factor in 84% of metastatic uveal melanoma somatic samples and has also been postulated to play a role in cutaneous melanoma. Not only has BAP1 been implicated in sporadic and familial cases of melanoma but also there is evidence that BAP1 mutations are associated with paraganglioma, mesothelioma, and renal and lung cancer. Although cyclin-dependent kinase–associated candidate genes remain under study, there is no established validated assay for genetic testing.
Phosphatase and Tensin Homologue, 9p21, and Additional Candidate Loci for Melanoma Predisposition
Phosphatase and tensin homologue (PTEN) is another important candidate for genetic testing in melanoma. PTEN is a phosphatase gene in which multiple mutations have been identified and have been implicated in the pathology of multiple organ systems. PTEN mutations are associated with the PTEN hamartoma tumor syndromes, including Cowden and Bannayan-Riley-Ruvalcaba syndromes. Diagnostic criteria for Cowden syndrome include mucocutaneous lesions and gangliocytoma. Although the vast majority of patients with the PTEN mutation failed to demonstrate the phenotypic diagnostic criteria, they still had an 85% chance of developing cancer by age 70 years and a 6% chance of developing melanoma. PTEN is located on chromosome 9p21. Loss of heterozygosity at 9p21 has been independently linked to melanoma carcinogenesis, and additional genes in this region are being sought. In addition, the 1p22 locus has been correlated in melanoma cases that were CDKN2A and CDK4 mutation negative. However, specific gene targets remain under investigation. Recent studies have identified the 10q25.1 locus as a genetic association with melanoma, with single nucleotide polymorphisms significantly increasing the risk of melanoma, but specific genes have not been identified yet. Other loci under investigation include 3p29, 17p11 to 12, and 18q22, but their link to melanoma have not been thoroughly validated. Another candidate is the 20q11 locus, which encodes a signaling protein that controls hair color and is a MC1R antagonist, which has been correlated with an increased risk of melanoma. However, no interaction between variants at this locus, MC1R variants, and melanoma risk have been proved to date (see later discussion).
Melanocortin 1 Receptor
Mutations in MC1R have been correlated with red hair, fair skin, poor tanning, and increased skin cancer risk, and thus MC1R has been investigated as a candidate for genetic testing in melanoma. Although some studies demonstrated a link between melanoma risk and MC1R mutation in patients with red hair, other studies demonstrated the link in MC1R mutants with dark hair, dark complexion, and good tanning ability. In addition, an independent link between the R163Q DNA sequence variant of MC1R and melanoma was identified, as well as an increased risk of basal cell carcinoma in MC1R mutants whose other characteristics are traditionally considered low risk. Similar findings have also been reported in cutaneous squamous cell carcinoma. Patients with both CDKN2A and MC1R mutations had a significantly increased risk of melanoma; however, this increased risk only remained significant in individuals with dark hair. Furthermore, patients with CDKN2A mutation had greater increased risk of melanoma when they expressed one or more MC1R variants, and this risk was seen among younger age groups. For example, both Arg160Trp and Asp84Glu mutations of MC1R have been associated with increased melanoma risk in CDKN2A mutants. There is some evidence that MC1R mutations may correlate with improved survival and may thus provide prognostic information.
Microphthalmia-Associated Transcription Factor
The microphthalmia-associated transcription factor (MITF) regulates melanocyte function; the E318K mutation has been implicated in melanoma, and thus MITF is another candidate for melanoma genetic testing. Not only has this mutation been found to be in higher prevalence in melanoma cases but it has also been identified in CDKN2A and CDK4-negative melanoma cases. That correlation was found in patients who had a light complexion, nevi, freckles, more than 1 primary melanoma, and amelanotic melanoma. Although population-based studies in the United Kingdom and Australia demonstrated an increased risk of melanoma correlated with the E318K MTIF mutation, a similar correlation was not demonstrated in a population-based Polish study. It remains under investigation and has not yet been established for genetic testing in clinical practice.
BRCA
Although BRCA2 mutations have been established as an important genetic factor in carcinogenesis in other cancers, their role in melanoma remains uncertain and under investigation. Despite initial reports of an increased risk of melanoma in BRCA2 carriers as high as 2.6 times that of the general population, further studies have not demonstrated the same significantly increased risk of melanoma. A Dutch study reported a lower risk of developing melanoma in BRCA2 carriers. Studies of melanoma cases in the Ashkenazi Jewish population have failed to demonstrate any increased rate of either BRCA2 or BRCA1 positivity.
The Current Role of Genetic Testing in the Clinical Management of Melanoma
Although genetic testing is available for CDKN2A, the most established genetic risk factor for melanoma, its role in clinical practice remains controversial. Advocates for genetic testing cite the importance of accurate diagnosis, improving patient compliance with screening for early detection, and the value of a negative test result for individuals in a mutation-bearing family. However, as discussed earlier, many patients who have a genetic predisposition still test negative for CDKN2A mutations. Furthermore, individuals in a family with CDKN2A mutation who test negative for CDKN2A are still at an increased risk of developing melanoma compared with the general population. In contrast, somatic genetic testing of melanoma tumors to identify which patients may benefit from targeted therapy has made significant progress. The dramatic advances made with the success of BRAF-targeted therapy has changed significantly the landscape of the multidisciplinary management of metastatic melanoma with routine testing for the BRAFV600E mutation. The clinical applications of genetic testing for assessing the risk of developing melanoma remain unclear, and instead, the clinical assessment of risk remains based primarily on personal and family history.
Clinical Assessment of Melanoma Risk
Personal and family history are important risk factors for melanoma. Furthermore, environmental factors such as sun exposure also increase the risk of developing melanoma. Therefore, education about sun safety and early signs of melanoma is critical, including regular skin examinations by the appropriately skilled providers. Patients with a personal history of melanoma and/or a strong family history of melanoma whereby multiple first- or second-degree relatives have been diagnosed with melanoma should undergo semiannual examinations starting at age 10 years until nevi are considered stable, then annually thereafter. These patients should also be taught skin self-examination techniques (parents for the pediatric population), to be performed on a monthly basis. Observation of lesions may be aided by techniques such as full-body photography and dermoscopy. A cost-utility analysis has demonstrated the benefits of screening in the high-risk population. The same criteria for biopsy of suspicious lesions should be applied to this population, and prophylactic removal of nevi without suspicious features should not be advocated. Furthermore, it is important to consider that these patients are more likely to develop melanoma spontaneously rather than from a premalignant lesion. For individuals at normal risk, monthly self skin examinations and yearly whole-body skin examinations from a qualified physician are recommended for early detection of melanoma.
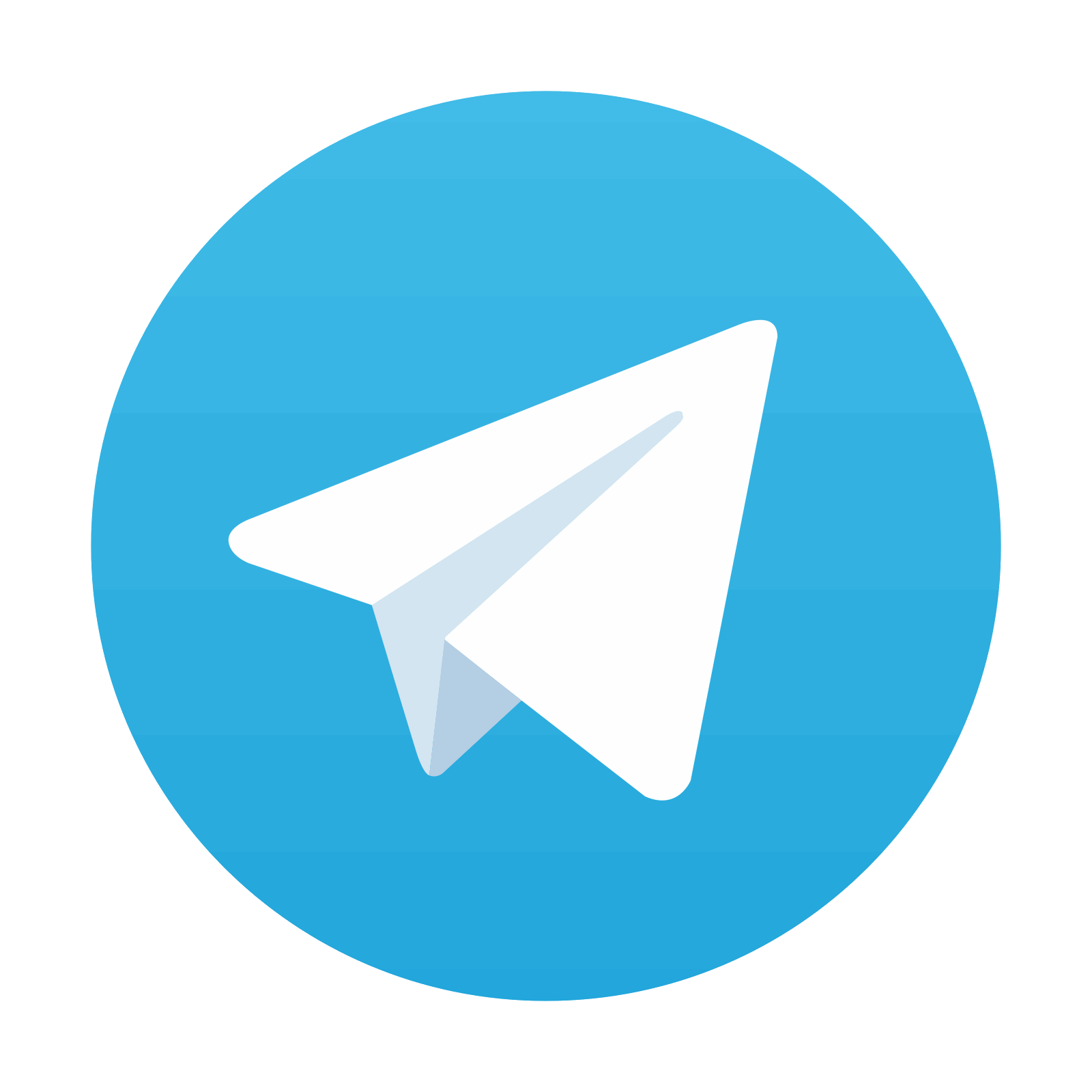
Stay updated, free articles. Join our Telegram channel

Full access? Get Clinical Tree
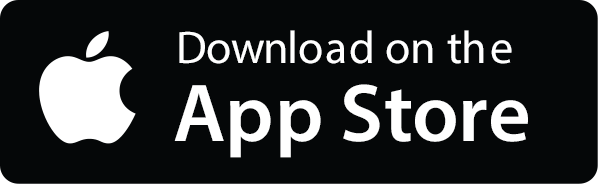
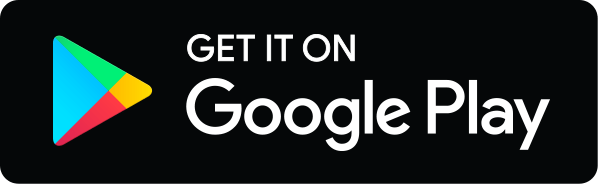