Abstract
In the last 30 years, an unprecedented production of new knowledge about the adrenal glands has led to subspecialization in the field: enzymatic deficiencies of steroidogenesis are mostly associated with CAH; in this chapter, we focus on congenital causes of adrenal insufficiency (AI) associated with hypoplasia of the adrenal glands. Disorders that affect aspects of adrenal function at a young age have also been recently molecularly elucidated; these diseases do not affect embryonic or early (infantile) development of the adrenal glands. We also briefly discuss genetic causes of autoimmunity that affect the adrenal gland, as these patients often present with AI at an early age. A brief outline of adrenal embryonic development precedes nosology, as the genetics of the latter can only be understood in the context of the former.
Keywords
adrenal, cortex, congenital adrenocortical hypoplasia, adrenocortical insufficiency, molecular genetics, DAX1, TPIT, SF1, glucocorticoid resistance, mineralocorticoid receptor, sodium channel defects
Introduction
Reference to the existence of the adrenal glands dates back to the times of Galen (130–201 AD); he was believed to be the first to describe the left adrenal vein. There are also some accounts that the phenotype of the biblical monozygotic twins, Esau and Jacob, in the Book of Genesis was consistent with congenital adrenal hyperplasia (CAH). But it was not until 1552 that a professor at the Collegio della Sapienza in Rome, Batholemeus Eustachius, gave the first clear description of the adrenal glands. In the last 30 years, an unprecedented production of new knowledge about the adrenal glands has led to subspecialization in the field: enzymatic deficiencies of steroidogenesis are mostly associated with CAH; in this chapter, we focus on congenital causes of adrenal insufficiency (AI) associated with hypoplasia of the adrenal glands. Disorders that affect aspects of adrenal function at a young age have also been recently molecularly elucidated; these diseases do not affect embryonic or early (infantile) development of the adrenal glands. We also briefly discuss genetic causes of autoimmunity that affect the adrenal gland, as these patients often present with AI at an early age. A brief outline of adrenal embryonic development precedes nosology, as the genetics of the latter can only be understood in the context of the former.
Genetics of embryology and function of the adrenal glands
The human adrenal cortex forms at the fourth week of embryonic development: on the 25th gestational day (embryonic day 10 in the mouse), a blastema of undifferentiated cells of mesodermal origin forms from the medial part of the urogenital ridge as a condensation of coelomic epithelial cells or from mesoderm that is intermediate between the mesonephros and the coelomic cavity. During the fifth embryonic week, the adrenogonadal primordium cells (which express steroidogenic factor 1, SF1) proliferate and invade the underlying mesenchyme; the adrenocortical primordium is separate from the gonads by 33 days postconception. A second wave of mesodermal cells then penetrates and surrounds the original cell mass by the sixth to eighth week, forming the first evidence for zonation. The latter cells are smaller than those of the first migration and form what has been called the definitive zone (DZ), whereas the former mesothelial cells develop into the fetal zone (FZ). These processes are to some extent under the control of fetal adrenocorticotropic hormone (ACTH, or corticotrophin) and cortisol is being made for the first time at about the sixth week reaching a peak between the eighth and ninth week of development; cortisol secretion at this early stage is from the rapidly growing FZ and is necessary to protect female sexual development from excess ACTH-driven adrenal androgen production. An additional cell type takes origin from the mesonephron and seems to arise from the region of Bowman’s capsule. Transitional zone (TZ) is a thin layer of cells between the small outer DZ and the much bigger FZ; although cortisol synthesis at the early phase is from the FZ, gradually aldosterone and cortisol are being made from the DZ and TZ cells, whereas the FZ produces primarily dehydroepiandrosterone (DHEA) and dehydroepiandrosterone-sulfate (DHEAS) that support estrogen production through the fetal- placental unit.
Thus, adrenal cortex derives from three embryologically distinct mesodermal cells, two from the celomic epithelium and one from the mesonephron. The adrenal capsule and outer regions of the adrenal cortex have long been hypothesized to contain progenitor cells that mediate adrenocortical maintenance. Recent studies have elucidated the cellular origin of the capsule and have identified two mutually exclusive capsular progenitor populations of the adult adrenal cortex, expressing Nr5a1 and Gli1. By the ninth week, the now encapsulated adrenal accepts migrating neural crest cells that will form the adrenal medulla. It is not until after birth and after the involution of the FZ that a clear separation exists at the corticomedullary junction between steroid hormone-producing and catecholamine-secreting cells. During fetal life, medullary cells are intermingled with cortical cells of the FZ; examples of that intermingling between neuroendocrine and cortical cells remain visible even in the adult adrenal gland, and are important regulators of corticomedullary coordination of adrenal function.
Between the ninth and 12th embryonic week, sinusoidal vascularization of the glands forms the framework for the zonation of the adult cortex. By the fourth month, the fetal adrenal attains its maximum size and becomes actually larger than the kidney; most of its volume occupied by the FZ. After the fourth month, the FZ starts receding, very gradually, at least at the beginning (despite some original evidence for massive collapse and hemorrhagic necrosis), while the thin DZ and TZ start expanding and will gradually give rise to the adult zona glomeruloza (ZG) and zona fasciculata (ZF), respectively. At birth, a dramatic reduction in adrenal mass occurs as a result of rapid degeneration of the FZ. It is not clear what initiates the process of degeneration of the fetal zone; it happens in anencephalic infants, although in the absence of proopiomelanocortin (POMC) or ACTH, regression of the FZ is even faster.
By the end of the first year postpartum, a TZ between adult and FZ is still present, FZ at this time being primarily fibrous tissue between the adult cortex and the medulla. The first evidence for an anatomically distinct zona reticularis (ZR) appears by the end of the second year of life, but unequivocal immunohistochemical evidence of steroidogenic activity is not present until the age of five years, concomitant with the onset of adrenarche. It is not clear when the adult adrenal cortex reaches maturity: estimates vary from as early as eight years of age to as late as after mid-puberty (11 years of age).
Genetic defects causing CAI: an overview and a comment on treatment
As one would expect from its complex developmental biology, several genes work together in parallel as well as vertical networks orchestrating adrenocortical organogenesis. They were discovered mostly over the last 20 years from mouse models, as well as the cloning of genes responsible for unique human disorders affecting the adrenal gland. In a Canadian cohort with experience of treating children with CAI defects over 20 years (1981–2001), CAH was the most frequent etiology (71.8%) and non-CAH etiologies accounted for 28.2%, of which 55% were nonautoimmune in etiology.
Many of the genetic disorders affecting adrenocortical development and causing CAI have not been molecularly elucidated. One of the recently elucidated ones is IMAGe (intrauterine growth retardation, metaphyseal dysplasia, adrenal hypoplasia, genitourinary anomalies), which is now known to be caused by mutations in the CDKN1C gene in chromosome 11p15. Cases of chromosomal anomalies, such as one with a 5p defect and CAI are useful potential indicators of new genetic loci involved in adrenal development and/or function. Finally, rare associations, such as the one of CAI and CHARGE syndrome (coloboma of the eye; heart anomaly; atresia, choanal; retardation of mental and somatic development; microphallus; ear abnormalities and/or deafness) that is caused by mutations in the chromodomain helicase DNA-binding protein-7 ( CHD7 ) gene may point to new functions of already identified genes.
CAI Associated with Hypothalamic-Pituitary-Adrenal (HPA) Axis Defects
As presented earlier, sufficient levels and function of ACTH and its receptor (MC2R) are essential for normal adrenocortical growth and differentiation. Genetic diseases affecting the pituitary (mutations of the HESX1 , LHX3 , LHX4 , SOX3 , and TPIT genes), homozygote or compound heterozygote genetic defects of the proopiomelanocortin ( POMC ) gene (the ACTH precursor), its processing enzyme (prohormone convertase 1 or PC1), the product of the proprotein convertase subtilisin/kexin type 1 ( PCSK1 ) gene, and the MC2R gene or the genes providing its accessory molecules ( AAAS , MRAP for definition of the abbreviation see later) all lead to CAI. Defects that lead to hypopituitarism (ACTH deficiency in combination with other defects), and POMC and PC1 gene mutations that cause, among other symptoms, pediatric obesity are discussed elsewhere in this book, so in this chapter we discuss further CAI due to TPIT (isolated ACTH deficiency), MC2R , AAAS , and MRAP (ACTH resistance) gene defects.
CAI Associated with Primary Adrenocortical Development Defects
The development of the adrenogonadal primordium from the urogenital ridge is dependent on normal function and interplay between the transcriptional factors SF1 and DAX-1 (for definition of the abbreviation see later). Mice knockouts (KO) for Sf1 have complete absence of the adrenal glands, whereas mice KO for Dax1 have developmental defects in their adrenal glands (but they are not adrenally insufficient).
Humans with heterozygous SF1 (coded by the NR5A1 gene) mutations have AI and gonadal abnormalities but this defect is a rare cause of CAI, although recently more patients with AI and heterozygous NR5A1 mutations have been described. On the other hand, X-linked DAX-1 (the NR0B1 gene) defects cause the most common form of CAI in humans, adrenal hypoplasia congenita (AHC): out of 117 patients with mostly CAI (and some adults with AI), DAX-1 gene mutations were found in 37 (58%) 46,XY phenotypic boys referred with adrenal hypoplasia and in eight boys with hypogonadotropic hypogonadism and a family history of male-only CAI; SF1 gene mutations were found in only two patients who also had 46,XY gonadal dysgenesis. Mutations in the NR0B1 (DAX1) gene are now known to associate growth hormone deficiency with CAI. More than 200 mutations in the NR0B1 (DAX1) gene have been identified to date; the phenotype varies with the type of mutation including hypogonadotropic hypogonadism, CAI, and impaired synthesis and release of gonadotropins.
Since adrenocortical development is tightly linked to that of the gonads and other structures derived from the urogenital ridge, genes encoding proteins such as Wilms’ tumor 1 ( WT1 ), a transcriptional regulator that is mutated in Denys–Drash and Frasier syndromes, and WNT4 and WNT11 , both involved in signaling via the frizzled receptor family, are important for normal adrenocortical formation. Mice that are KO for Wt1 and Wnt4 have hypoplastic adrenals but the adrenocortical function of patients with Denys–Drash and Frasier syndromes has not been studied extensively; mild functional defects in adrenarche and pubertal anomalies may be present but not CAI. A female with 46,XY karyotype associated with a 1p31-p35 duplication including the WNT4 gene was described; recently, a missense substitution of the WNT4 gene was found in an autosomal recessive syndrome designated SERKAL: female-to-male s ex r eversal and k idney, a drenal, and l ung dysgenesis. The defect reduced WNT4 mRNA levels and thus downregulated WNT4 -dependent inhibition of the degradation of beta-catenin, a process essential for adrenal and other organ development.
SF1 ( NR5A1 ) mutations have rarely been identified in patients with CAI without any evidence of gonadal defects; one of the first patients to be described was a phenotypically normal girl who presented at age 14 months with adrenal insufficiency, normal female chromosomal pattern (46,XX), normal luteinizing hormone, and follicle-stimulating hormone. The defect was a heterozygous G-to-T transversion in exon 4 of the NR5A1 (SF1) gene, leading to an arg255-to-leu mutation in the hinge region of the NR5A1 protein.
See more discussion on DAX1 and SF1 defects, under the listing of adrenal hypoplasia congenital (AHC).
CAI Associated with Metabolic Disorders
There are a few metabolic disorders that affect adrenocortical function in early life and can, thus, cause CAI. Wolman’s disease (familial xanthomatosis) may cause CAI and is occasionally associated with adrenal calcification, too. This rare metabolic disorder is caused by lysosomal acid cholesteryl ester hydrolase defects. Smith–Lemli–Opitz syndrome (SLOS), a disorder of cholesterol biosynthesis associated with developmental delay, dysmorphic features, male undervirilization, and/or hypogonadism, is often cited as one frequently associated with CAI. Rare cases of SLOS may present with adrenal crisis, but in our experience, most SLOS patients have mostly compensated adrenocortical dysfunction and not CAI, consistent with prior reports of functional abnormalities of the fetal-placental unit in this condition. X-linked adrenoleukodystrophy (X-ALD) is by far the most common metabolic disorder causing CAI and is discussed further in the subsequent section.
CAI Associated with Adrenal Calcifications and/or Hemorrhage: Genetic Factors
Adrenal hemorrhage, a well-recognized obstetrical complication of the newborn, is now a rare cause of CAI due to the improvements in care during labor and delivery. The adrenal glands often become calcified and the classic presentation is that of an infant in adrenal crisis and calcifications in the adrenal fields, usually bilaterally. Risk factors, besides difficulties in labor and delivery, include genetic disorders of the newborn, such as macrosomia and related syndromes, coagulation defects, metabolic disorders, congenital masses including hemorrhagic and teratomatous cysts, ganglioneuromas, pheochromocytomas, neuroblastomas, and adrenocortical cancer.
A newly described rare disease is hereditary cystatin C amyloid angiopathy; it is a genetic amyloid disease that occurs frequently in Iceland. The condition is caused by a mutation in cystatin C that causes amyloid deposition, predominantly in brain arteries and arterioles, but also in tissues outside the brain including the adrenal cortex. The abnormal deposits lead to occlusion or rupture resulting in hemorrhage in the brain, adrenal and rarely in other tissues.
CAI Associated with Genetic Defects Leading to Autoimmunity
Autoimmune polyglandular failure, involving the adrenal glands, usually presents after the first two years of life along with other autoimmune diseases in the form of two genetic syndromes: multiple endocrine abnormalities types 1 and 2 (MEA-1 and MEA-2). In the Canadian cohort of primary AI, the most frequently encountered autoimmune CAI was the autoimmune polyendocrinopathy-candidiasis-ectodermal dysplasia (APECED) syndrome. Patients with APECED presented with AI four years earlier than those with nonautoimmune disease. AI in APECED occurs in association with chronic mucocutaneous candidiasis and/or acquired hypoparathyroidism, chronic active hepatitis, malabsorption, juvenile onset pernicious anemia, alopecia and primary hypogonadism, whereas insulin-dependent diabetes and/or autoimmune thyroid disease are infrequent. AI in the context of MEA-2 is associated with insulin-dependent diabetes and/or autoimmune thyroid disease, has a later but variable age of onset, and occurs predominantly in females. The association of HLA-B8, -DR3, and -DR4 with MEA-2, and more recently the cytotoxic T-lymphocyte antigen (CTLA-4) gene, and the protein tyrosine phosphatase nonreceptor type 22 ( PTPN22 ) genes on chromosomes 6, 2, and 1, respectively, but not with MEA-1, further confirms the different clinical and genetic nature of these two syndromes, which accounts for almost all pediatric and approximately 50% of adult cases of autoimmune AI. In addition, 100% concordance of disease in identical twins has not been shown.
MEA-1 or APECED is a genetic disorder inherited in an autosomal recessive manner and caused by mutations in the a uto i mmune re gulator ( AIRE ) gene. Although technically not a congenital form of AI, we discuss it briefly in the subsequent section because if its frequency among young pediatric patients with AI (for details see Chapter 27 ). We do not discuss here MEA-2, a syndrome that affects mostly older children and adults and has no known single gene as a cause (see Chapter 27 ).
Treatment
All disorders leading to AI, whether primary (peripheral or due to adrenal defects) or secondary (central, due to hypothalamic or pituitary defects) require treatment with adequate glucocorticoid coverage, most commonly with hydrocortisone at the usually recommended dose of 10–12 mg/m 2 /day. The dose schedule varies, but most patients do well with a twice-a-day administration of two-thirds of the total dose in the morning and one-third in the early evening hours. The usual stress coverage dosing recommendations also apply. It is important to note that the ACTH levels of patients with primary AI vary widely and should not be routinely monitored, as this often leads to increased glucocorticoid administration with detrimental long-term effects. Titration of coverage should be done by careful clinical examination and consideration of the patient’s overall well-being and not aim at suppressing ACTH levels.
Mineralocorticoid replacement is usually not necessary in disorders associated with secondary (central) AI. It is also rarely needed in patients with ACTH resistance syndromes. Most patients with primary AI due to an adrenal developmental defect require mineralocorticoid replacement: the usual dose is 0.1 mg fludrocortisone per day; salt may also be given in these patients. For detailed guidelines, please refer to the newly published consensus statement on the diagnosis, treatment, and follow-up of patients with primary adrenal insufficiency.
Specific genetic conditions associated with CAI
Congenital Isolated ACTH Deficiency
TPIT (or TBX19 ) is a transcription factor, member of the T-box gene family that is required for expression of the POMC gene in the differentiating pituitary corticotrophs. All members of the T-box gene family encode an N-terminal DNA-binding domain (the T-box) and are important for development of several, mostly mesodermal, tissues in the human and mouse embryo. Holt–Oram ulnar-mammary and DiGeorge syndromes are all caused by mutations in a member of this family of transcription factors.
TPIT gene mutations are associated with autosomal recessive CAI. Most patients with CAI and TPIT mutations presented with neonatal hypoglycemia, seizures, and occasionally death; all patients had very low (but not necessarily undetectable) ACTH and cortisol levels. Patients with isolated ACTH deficiency and CAI have a high likelihood of having TPIT mutations, but patients that have either late onset AI or other pituitary and/or other developmental defects should generally not be tested for defects of this gene. Studies of congenital isolated ACTH cases found that about 65% of patients with CAI (neonatal onset) had TPIT mutations. A recent study identified mutations that led to loss of function by different mechanisms, such as nonsense-mediated mRNA decay, abnormal mRNA splicing, loss of TPIT DNA binding, or protein-protein interaction defects.
ACTHR Defects (Familial Glucocorticoid Deficiency 1, FGD-1)
Hereditary resistance to ACTH action (RACTH) or familial glucocorticoid deficiency (FGD) type 1 (FGD-1) is an autosomal recessive disorder that is caused by defects of the ACTH receptor (ACTHR) (the MC2R gene). The MC2R gene, with only one coding exon, is on chromosome 18 (18p11). The presentation of the disease is quite variable with little genotype-phenotype correlation, although certain mutations may have a more specific phenotype, such as the homozygous insertion of a cytosine nucleotide between codons 153 and 154 (c.459_460insC) that was associated with thyroid dysfunction, growth hormone secretion abnormalities, and hyperferritinemia. Patients can present in infancy with severe AI, hypoglycemia, and seizures, or later in childhood with a milder form of AI. The occasional patient may require mineralocorticoid replacement, but most require only glucocorticoid replacement; ACTH levels can vary from slightly above the normal range to several-fold higher. Finally, patients with MC2R defects tend to be taller than their expected (by genetic target) height, a phenotype that is largely unexplained.
Inactivating MC2R gene mutations in FGD-1 are responsible for 25–40% of FGD cases. Thus, for genetic counseling purposes, one needs to know that the chance of MC2R gene mutation(s) in a patient with isolated ACTHR defects (with no other health or developmental problems) appears to be significantly less than 50%, with only few of the remaining patients bearing mutations in a known gene (e.g., MRAP ).
Recently, disruption of Mc2r in mice led to neonatal lethality in most but not all animals, in agreement with the variability of the phenotype in humans. Mice that survived had small adrenal glands with atrophic ZF confirming the role of ACTH and ACTHR in the development of the mature (adult) adrenal cortex.
Other Genetic Defects for Familial Glucocorticoid Deficiency Type-2 (FGD-2)
Recently, it was discovered that at least some of the non-ACTHR-mutant FGD cases, FGD-2, have mutations in the gene encoding an accessory protein required for ACTH signaling, the MC2 receptor accessory protein (MRAP). Lack of functional MRAP gene defects, like MC2R gene defects, causes RACTH and severe glucocorticoid deficiency that can be fatal if the condition is not recognized and treated. MRAP exists as a homodimer and is essential for the cell surface expression of the MC2R. In the absence of MRAP, MC2R is trapped in the endoplasmic reticulum, but with MRAP, MC2R is glycosylated and can be present in the plasma membrane for proper ACTH signaling.
MRAP gene mutations are an overall rare cause of RACTH; other genes are continuously sought, including genes that confer an AI phenotype in mice: a gene ( acd ) responsible for the urogenital and caudal dysgenesis and adrenocortical dysplasia in mice was investigated in patients with FGD-2 and no mutations were found.
New gene defects in FGD have recently been recognized in the mini-chromosome maintenance-deficient 4 homologue ( MCM4 ) and nicotinamide nucleotide transhydrogenase ( NNT ) genes. These findings highlight new pathogenetic mechanisms involved in replicative and oxidative stress and set the basis for future studies to expand the spectrum of pathways for FGD.
Allgrove or Triple-A Syndrome
Allgrove or triple A syndrome (AAAS) is an autosomal recessive condition characterized by RACTH A I, reduced or absent tearing ( a lacrima), and a chalasia. Patients can also exhibit other signs of autonomic dysfunction such as ocular abnormalities, an abnormal reaction to intradermal histamine, abnormal sweating, orthostatic hypotension, and heart rate disturbances, leading some to suggest the name “4A syndrome.” Both static and progressive neurological abnormalities – including microcephaly, mental retardation/learning disabilities, bulbospinal amyotrophy, dysarthria/nasal speech, optic atrophy, ataxia, muscle weakness, dementia, hyperreflexia, Parkinsonian features, and sensory impairment – have been reported. Palmar and plantar keratosis is a frequent dermatologic manifestation.
Thus, AAAS is a multisystem disorder with endocrine, gastrointestinal, ocular, and neurologic manifestations. The “classic” presentation reported in the literature is that of a child born healthy who does not make tears when crying, develops ACTH-resistant adrenal insufficiency in the first decade of life, and goes on to have achalasia some time in the first or second decade of life. Our experience, however, is that AAAS is clinically a heterogeneous disorder with highly variable severity of presentation. For example, it is well recognized that while most children are born healthy and normal, a small percentage appears to have developmental abnormalities such as microcephaly and mental retardation/developmental delay. AI due to RACTH may present in a dramatic fashion (e.g., hypoglycemic seizures, adrenal crisis) or may be mild and not require pharmacologic replacement of glucocorticoids until teenage years or later. For example, we encountered a case who did not present with AI until age 13, despite being a compound heterozygote for two AAAS mutations. The pituitary may appear hypoplastic on magnetic resonance imaging, despite clear evidence of RACTH being the cause of adrenal insufficiency. While adrenal failure is generally limited to glucocorticoid deficiency, mineralocorticoid deficiency has also been reported. However, in at least one case, an intact ZG was reported on autopsy consistent with the low incidence of mineralocorticoid deficiency. Achalasia may also have a variable – often insidious – presentation. Dysphagia may be present for years before a diagnosis of achalasia is made and may present as gastroesophageal reflux. In our experience, surgical intervention is usually successful. However, anesthesiologists should be warned about the possible proclivity of peripheral neuropathy in these patients and should take appropriate intraoperative precautions. The autopsy case presented by Allgrove showed muscular hypertrophy, loss of ganglion cells, and a paucity of small nerves in the distal esophagus of an affected patient.
Ocular abnormalities may be the most invariable and present the earliest. The loss of basal and reflex tearing (which is under parasympathetic control) likely stems from disease of the autonomic nervous system and may result in corneal punctate epitheliopathy, melting, and/or scarring. The main lacrimal gland appears small to absent on neuroimaging, either because of primary hypoplasia or secondary denervation atrophy. Autonomic dysfunction may also result in pupillary abnormalities and/or accommodative spasms. Optic nerve pallor, generally described in the literature as “optic nerve atrophy” with either delayed timing or reduced amplitude on visual-evoked potential testing, has been reported is several cases. It is unclear from most reports whether these optic nerve changes are secondary to episodes of hypoglycemia from adrenal insufficiency or represent a primary, progressive neurodegenerative process.
The most concerning and least treatable manifestations of AAAS are related to central and peripheral neurodegeneration. While symptoms may present early in life, they are not invariably present. Polyneuropathy (sensory, motor, and autonomic components), Parkinsonian features, dementia, bulbospinal amyotrophy, and selective ulnar nerve involvement in patients with peripheral motor neuropathy and amyotrophy have all been reported in separate case reports. Counseling presymptomatic, mutation-proven patients regarding neurologic prognosis is difficult, as it is currently unclear if all patients with AAAS go on to develop significant neurologic deterioration.
Mutations in the AAAS gene on 12q13, which codes for a 546 amino acid protein called ALADIN (for a lacrima- a chalasia- a d renal i nsufficiency n eurologic disorder), have been described in several individuals. A splicing mutation, the IVS14+1G>A change, is the most common AAAS gene mutation. The frequent presence of this mutation appears to be the result of a founder effect. New mutations have also been found, however, in ethnically diverse or mixed populations. There is little, if any, genotype-phenotype correlation.
The results of a recent international literature search revealed a delayed onset of symptoms in patients with missense mutations in one allele as compared to those with truncation mutations in both alleles; for example, alacrima, adrenal insufficiency, and motor impairment developed significantly later in patients with missense mutations than in those with only truncation mutations. The frequency of adrenal insufficiency was also significantly higher in patients with only truncation mutations; in contrast, the frequencies of occurrence of other major symptoms (achalasia, alacrima, and motor impairment) did not differ significantly. ALADIN, the AAAS protein, belongs to the WD-repeat family of regulatory proteins that have functions ranging from transmembrane signaling and transcription to cell division and intracellular trafficking. While the precise function of ALADIN is unknown, it appears to be a protein in the nuclear core complex of cells; a variety of missense, nonsense, and splicing mutations in ALADIN cause the protein to mislocalize to the cytoplasm. Because microscopic analysis of cells from an AAAS patient showed no morphologic abnormalities in the nuclei, nuclear envelope, or nuclear pore complexes, it has been suggested that most missense AAAS mutations result in a functional, rather than a structural, abnormality in the nuclear pore complex. Interestingly, Aaas deficient mice largely failed to replicate the human phenotype.
Is AAAS a genetically homogeneous disorder? Some of the studied patients, although they clinically had unequivocally AAAS, did not have mutations in both alleles of the AAAS gene. In addition, AAAS gene mutations are completely absent in a number of patients with the triple A syndrome. These findings raise the possibility of mutations in regulatory or deeper intronic sequences of the AAAS gene, and/or of genetic heterogeneity with mutations expected to be found in molecules that are functional partners of the ALADIN protein.
Adrenal Hypoplasia Congenita (AHC)
AHC is a disease that has at least two major histologic types: the “cytomegalic” form and the “miniature” form. The cytomegalic form typically presents in infancy with adrenal insufficiency; these children who are typically male ultimately fail to undergo puberty because of hypogonadotropic hypogonadism. The inheritance is X-linked recessive, although genetic heterogeneity may exist. The gene for the X-linked cytomegalic AHC was cloned in 1994: it was called DAX-1 , for d osage-sensitive sex-reversal [and] a drenal hypoplasia congenital [on the] X chromosome. DAX-1 is an orphan nuclear receptor that is expressed in the adrenal gland, gonads, ventromedial hypothalamus (VMH), and the pituitary gonadotropes. Most DAX1 gene mutations introduce frameshifts and/or cause premature termination of the protein. Relatively few missense mutations have been described and most are located within the carboxy-terminal half of the protein. Functional assays show that AHC-associated DAX-1 mutations abrogate the ability of this gene to act as a transcriptional repressor of SF-1. There are “mild” missense DAX-1 gene mutations but, in general, genotype-phenotype correlation is not as strong as one might expect. Hypogonadropic hypogonadism, for example, appears to be particularly variable among patients both in its severity and age of onset. The Dax-1 KO mice show an important role of this gene in testis development and spermatogenesis, abnormalities that are also present in humans.
The miniature form of AHC appears to be autosomal recessive (it has been reported in both males and females) and is much less common than the cytomegalic form. It is often associated with other developmental defects including abnormalities of the pituitary gland and the central nervous system. When these defects are present, the clinical course is ominous and diagnosis is often made in infancy. The disease may be genetically heterogeneous.
Targeted mutagenesis of Sf1 in mice (the FtzF1 gene) prevents gonadal and adrenal development and causes male-to-female sex reversal. Several naturally occurring mutations have been previously reported and reviewed. At first, a heterozygous loss-of-function human SF1 gene mutation (G35E) in the first zinc finger of the DNA binding domain was described in a patient with adrenal insufficiency and 46,XY sex reversal. A second patient, a 46,XX prepubertal female with adrenal insufficiency was otherwise phenotypically normal; she had a heterozygous mutation of the SF1 gene in the hinge region corresponding to the AF-1 domain. Another mutation caused sex reversal but had no effect on adrenal function, whereas the newest SF1 mutation, in an infant with homozygosity for the R92Q genetic SF1 change, both adrenal insufficiency and sex reversal were present. In functional assays, this mutant SF1 protein exhibited partial loss of DNA binding and transcriptional activity when compared with the more severe G35E P-box mutant The parents of the patient and a heterozygote sibling were all unaffected, suggesting that overall SF1 gene mutations can present with a recessive or a dominant inheritance, depending on the defect.
X-Linked Adrenoleukodystrophy (X-ALD)
X-ALD is the most common inherited peroxisomal disorder, affecting 1/15,000–20,000 males in the Caucasian population. The disease is characterized by progressive demyelination of the white matter and by AI, although the latter is rarely congenital. In X-ALD, peroxisomal 5-oxidation of unbranched saturated very-long-chain fatty acids is defective or absent, depending on the molecular defect. The phenotype can vary widely from severe ALD and CAI (less than half of the patients) to only adrenomyeloneuropathy (one-quarter of the patients) or only AI (in a tenth of the patients). The remaining male carriers of pathogenic defects have no detectable disease until later in life or none at all, indicating that there are other, perhaps some environmental, factors that determine disease expression. The gene for X-ALD, ATP-binding cassette transporter 1 ( ABCD1 ) encodes a peroxisomal membrane transporter that belongs to the “ATP-binding cassette” superfamily of membrane proteins. To date, a total of 591 mutations have been identified in the ABCD1 gene in 1,260 kindred worldwide. The phenotypic variability is extremely broad, ranging from cerebral inflammatory demyelination of childhood onset, and early death, to adults remaining presymptomatic through more than five decades. There is no general genotype-phenotype correlation in X-ALD.
APECED (MEA-1)
APECED is a rare autosomal recessive disorder that is also extensively discussed in Chapter 27 . APECED patients usually have at least two out of three main symptoms: Addison’s disease, hypoparathyroidism, and chronic mucocutaneous candidiasis. Patients may also develop other organ-specific autoimmune disorders leading to gonadal failure, pancreatic (β-cell) deficiency, gastric (parietal cells) dysfunction, hepatitis, and thyroiditis. Other, less common, clinical manifestations include ectodermal dystrophy, affecting the dental enamel and nails, alopecia, vitiligo, and corneal disease (keratopathy). MEA-1 usually occurs in early childhood, but new, tissue-specific symptoms may appear throughout life. Immunologically, the main finding in APECED patients is the presence of autoantibodies against the affected organs, including those against steroidogenic enzymes (P450scc, P450c17, and P450c21) in patients with Addison’s disease, glutamic acid decarboxylase in patients with diabetes, and the enzymes aromatic L -amino acid decarboxylase and P4501A2 in patients with liver disease. Mucocutaneous candidiasis, hypoparathyroidism, and AI usually present in this order in pediatric patients with APECED. As with the other manifestations of the syndrome, there is a wide variability of age of onset, from six months to 41 years, with a peak around 13 years of age. AI is usually one of the reasons these patients end up being diagnosed with MEA-I; it develops in 60–100% of patients with APECED and may be preceded by months or years of detectable adrenal cortex autoantibodies. APECED is more common in certain genetically isolated populations. In Finland, the incidence has been estimated to be 1:25,000 and in Iranian Jews, 1:9,000. APECED is also relatively common among Sardinians (1:14,400) and in northern Italy. Based on linkage analysis in Finnish APECED families, the locus for the APECED gene was mapped to chromosome 21q22.3, and recently, the gene responsible for this disease was cloned. It encodes a predicted 545 amino acid protein, which was named AIRE ( a uto i mmune re gulator). It contains two plant homeodomain (PHD)-type zinc finger motifs and a newly described putative DNA-binding domain SAND, a proline rich region, and three LXXLL motifs, all suggestive of a transcription regulator. AIRE is expressed in thymus, lymph node, and fetal liver, tissues that have important roles in the maturation of immune system and development of immune tolerance. These findings, together with the immunologic deficiency in APECED patients, suggest that AIRE may have an important role in the control of immune recognition and may function as a transcription factor or as transcriptional coactivator. To date, several mutations in the AIRE gene have been described in APECED patients: a common Finnish mutation, R257X, was shown to be responsible for 82% of Finnish APECED cases, whereas a deletion of 13 nucleotides (1094–1106del) has been detected in several patients of different ethnic origin, and another nonsense mutation, R139X, is the major mutation among Sardinian APECED patients.
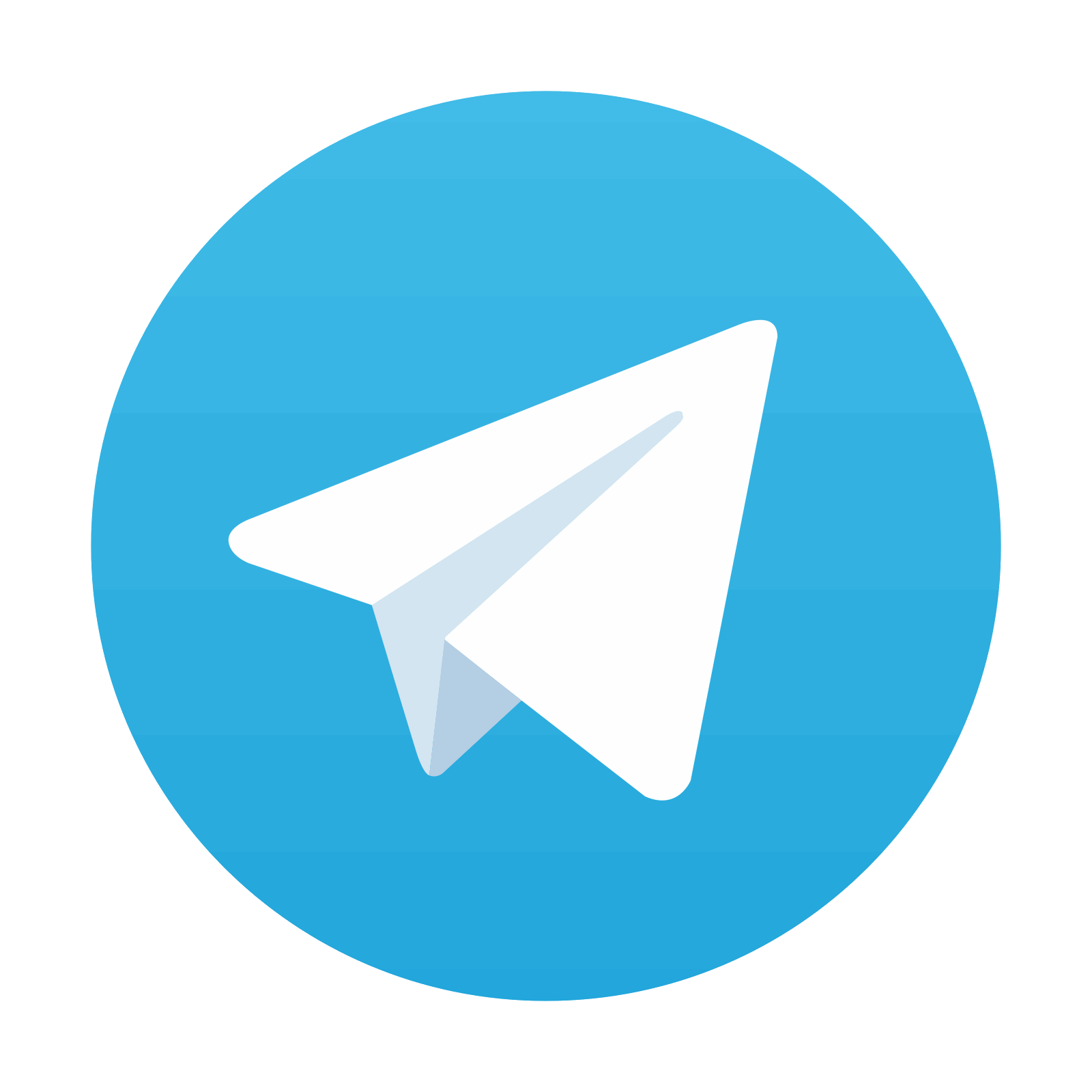
Stay updated, free articles. Join our Telegram channel

Full access? Get Clinical Tree
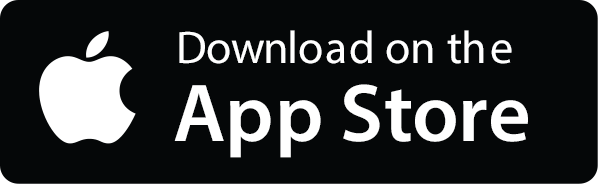
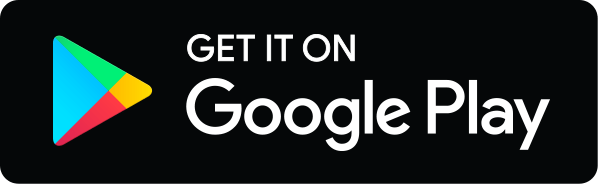