General Principles of Surgery
Christopher B. Weldon
Thomas E. Hamilton
Robert C. Shamberger
Surgery remains a critical component in the multimodal therapy of childhood cancer. Accurate staging and successful resection of tumors is of vital importance as we strive to minimize the morbidity of treatment. Decisions regarding biopsy and resection are most accurately made by surgeons who are trained to address these specific issues. This chapter presents an introduction to the significant considerations for surgical patients, including their metabolic response, nutritional support, and vascular access. Critical issues regarding anesthetic management, staging, biopsy, and preoperative adjuvant therapy are also discussed. A brief summary of some of the specific surgical issues for each solid tumor concludes the chapter.
METABOLIC RESPONSE TO MALIGNANCY
Weight loss is frequently seen in children with malignancy. This form of malnutrition, termed the anorexia-cachexia syndrome, is associated with higher early cancer relapse rates, decreased tolerance to chemotherapy, and a poorer prognosis. Although reduced food intake is an important component of the syndrome, cancer patients, unlike starved individuals, have elevated protein turnover and lose the ability to conserve skeletal muscle mass. The extent of this process may be more pronounced in those patients having malignancies with high cell turnover. Other notable metabolic changes with malignancy include increased rates of gluconeogenesis and lipolysis. There is also a failure to downregulate energy expenditure in reaction to reduced nutrient intake, although actual increases in resting energy expenditure are only evident in patients with large tumor burdens. Surgical stress or infection tends to further exacerbate the net protein catabolism found in these patients.
The pathogenesis of childhood cancer cachexia is complex and incompletely understood. Elaboration of host cytokines in response to the tumor, such as tumor necrosis factor alpha, interleukin-1, interleukin-6, and interferon gamma, promote catabolism, in certain instances is further enhanced by specific tumor-generated mediators. The cytokine-independent ubiquitin-proteasome pathway has also been linked to muscle protein loss in cancer patients. Compounding this catabolic process are a reduction in enteral intake and, on occasion, malabsorption of nutrients that may be caused by the tumor itself, antineoplastic therapy, or a combination of both. Megestrol and fish oil supplemented diets have been used to ameliorate anorexia-cachexia syndrome in oncology patients, but the usefulness of these interventions remains uncertain.1,2
Vascular Access
Dependable intravascular access in pediatric oncology patients is of vital importance and impacts the child’s care daily. It is utilized for the administration of chemotherapy, intravenous fluids, parenteral nutrition, blood and blood products, antibiotics and other medications, contrast agents for radiographic and imaging studies, and the resuscitation of critically ill oncology patients. Furthermore, it can be used to harvest peripheral stem cells for therapeutic bone marrow transplants, or as an access site for hemodialysis should it be required. Finally, just as it provides a dependable route of ingress, it is also the primary site for blood sampling without undue psychological or physical stress.
Catheters are divided by type and location, but for all, the common objective remains the establishment of a dependable and reliable access to the central venous system with the tip of the catheter at the junction of the superior or inferior venae cavae and the right atrium as confirmed by radiographic studies both during and after the procedure. The type of catheter can be either temporary or permanent, and it can be either external or internal. Regardless of location or catheter type, all central venous lines (CVLs) are placed using a percutaneous technique first described by Seldinger3 or by a cut-down technique depending on operator preference and patient characteristics. Prior to placing any CVL, coagulation and platelet studies should be assessed to determine the risk of perioperative hemorrhage. The patient’s venous anatomy should be confirmed by ultrasound or angiographic studies to ensure patency if there is any question of thrombosis or a history of multiple prior CVLs. Chlorhexidine solutions (2%) are the recommended antiseptic agents for skin preparation. Parenteral antibiotic (single dose of a first-generation cephalosporin [or clindamycin for those patients who are allergic to penicillin and/or cephalosporin-like drugs] 60 minutes prior to beginning the procedure) administration is a common practice during the placement of a CVL.4 Finally, in patients with central lines and a fever, a line infection must always be considered as the source.
Temporary external CVLs can be placed peripherally in an extremity (Peripherally inserted central catheters, PICC) or centrally (femoral, jugular, or subclavian routes), and they can have from one to four lumens. PICC lines are generally smaller in caliber than CVLs, and because they are placed peripherally, some complications associated with accessing central veins (pneumothorax, hemothorax, etc.) are avoided. However, even these small catheters have been associated with pericardial tamponade from erosion of a catheter tip through the atrial myocardium into the pericardium.5 The main advantage of temporary CVLs is that they do not require an anesthetic to remove, and in the case of PICCs, they do not require more than local anesthesia and occasional use of intravenous sedation to place them. However, these catheters do not have a grommet or cuff to prevent bacterial migration along the catheter or to allow fibrous ingrowth to anchor the catheter to the underlying subcutaneous tissue which prevents inadvertent removal. Multiple devices and suturing techniques have been employed to secure these CVLs, but none are infallible.
Permanent external CVLs are catheters that are placed in a similar fashion and location as temporary external CVLs except that the skin exit site of the CVL is distant from its insertion point. These CVLs are tunneled under the skin in the subcutaneous tissue. As an added measure of protection and stability, they have a cuff attached to the catheter wall that is buried above the skin exit site. This cuff allows for the ingrowth of fibrous tissue (scar) around the catheter, securing it and retarding the migration of microbes along the catheter itself, thereby decreasing the risk of infection.4 These catheters are frequently made of silicone as opposed to polyurethane or polyethylene, but one must be certain of
the composition of the catheter prior to placement because some catheters (polyurethane) do not tolerate ethanol locks utilized for infection prevention in some centers.6 They can have one or multiple lumens. Unfortunately, these CVLs require an anesthetic to both place and remove, but all attempts should be made to bundle the CVL placement with other necessary procedures (tumor resection, staging bone marrow biopsies, etc.) under the same anesthetic if possible.
the composition of the catheter prior to placement because some catheters (polyurethane) do not tolerate ethanol locks utilized for infection prevention in some centers.6 They can have one or multiple lumens. Unfortunately, these CVLs require an anesthetic to both place and remove, but all attempts should be made to bundle the CVL placement with other necessary procedures (tumor resection, staging bone marrow biopsies, etc.) under the same anesthetic if possible.
Permanent internal CVLs are also known as implantable venous access devices or ports. Like permanent external CVLs, they sit distant from the introduction site in the subcutaneous tissues. They are commonly secured with sutures to the deep fascia on the anterior chest wall to ensure that they do not migrate. The ports can be single or double lumen, and they come in a variety of materials that are not immunogenic. Special ports are now available that will allow for high-pressure injections for radiographic imaging, but they are generally made of polyurethane-based materials that also cannot tolerate ethanol locks. Regardless of the material utilized in the catheter, the body of the ports possesses silicone membranes that are accessed by special needles that are noncoring. The membranes are self-sealing after the needle is removed. These CVLs have many advantages, including decreased rates of infection, increased quality of life for the patients, and minimal pain when they are accessed, requiring only topical anesthetic creams.7 However, they do require an anesthetic to place and remove.
Complications of central venous access are mechanical (related to the venous access procedure or the catheter itself), infectious, or infusion related. With percutaneous Seldinger access techniques, the greatest patient risk is pneumothorax, hemorrhage from either arterial puncture or vein laceration, pericardial tamponade secondary to atrial or ventricular penetration, or needle puncture-related neurologic (vagus, phrenic, or sympathetic trunk) or thoracic duct injury. Other mechanical complications include phlebitis, thrombosis, thromboembolism, air embolism, and cardiac arrhythmias. Of concern is the very real risk of a life-endangering event from the vascular access procedure alone, a risk that approximates 1 such event per 1,000 access procedures.8
Infectious complications are most common for pediatric central venous access. It is important to distinguish catheter-related bloodstream infections from local exit-site, tunnel, or pocket infections. The local site infections typically account for up to 50% of all central access infections and present with local signs and symptoms, including fever, erythema, tenderness, and drainage. In contrast, bloodstream infections are diagnosed when a presumptive diagnosis of bacteremia or sepsis is made and there is congruence of the cultured organism from a portion of the catheter as well as a peripheral venous blood culture.9 However, as transcatheter antibiotics are typically initially administered in an effort to salvage the infected line, the presumption of a catheter-related infection is usually made on clinical suspicion as it is impossible to culture the catheter without its removal. The pediatric age group has remained a high-risk group for such infections, but these infected CVLs often can be treated effectively with antibiotics alone, although success is dependent on the organism.10 The microbiology of such infections is predictable; coagulase-negative staphylococci are the most common.11 In all series, but particularly in immunocompromised oncology patients, the spectrum of causal organisms includes other gram-positive, many gram-negative, and, not infrequently, fungal species. Factors that may protect patients from infection historically included the aseptic technique of the operating room, the tunnel, and the catheter cuff in addition to meticulous care of the catheters by well-trained medical personnel and parents. To these have been added antibacterial substances bonded to catheters; minocycline and rifampin have decreased the frequency of CVL infections, but not all types of CVL products are manufactured with antibiotic coatings.11
Risk factors for the pediatric patient that influence central catheter infections have been carefully analyzed.9 Early infection occurred in 12% of children (53 of 437), and factors that adversely influenced this rate included moderate (absolute neutrophil count <1,000) or severe (absolute neutrophil count <500) neutropenia and failure to use systemic prophylactic antibiotics. The type of catheter and site of placement did not influence the risk of infection. In this series, as in many others, transcatheter antibiotics as an initial therapy in presumed central line infection were effective in treating the infection and preserving the catheter in 70% of the patients.9 Such transcatheter antibiotic agents worked less well for the child with fungemia. Furthermore, because of the demonstrated relationship between thrombosis and infection, thrombolytic agents, anticoagulants, as well as antibiotics have all been used to treat the infected catheter.12
PRINCIPLES OF PERIOPERATIVE AND ANESTHETIC MANAGEMENT
Although the perioperative and anesthetic management of children with malignancy is generally similar to that of other ill pediatric patients, the following aspects warrant more detailed consideration: coagulation and transfusion, tumors that result in anterior mediastinal masses, and pheochromocytomas. In patients already treated with chemotherapy, a careful assessment of cardiac, pulmonary, renal, and electrolyte status is particularly warranted. Patients who have received cardiotoxic agents, such as doxorubicin (Adriamycin), require echocardiography to quantitatively document cardiac function before surgery.
Coagulation and Transfusion
Thrombocytopenia is the most commonly encountered clinical coagulation abnormality in children with malignancy requiring surgery. Platelet counts of 150,000 per mm3 are the lower limit of the normal range in children, whereas normal neonates may have platelet counts as low as 80,000 per mm3. Patients with platelet counts more than 10,000 per mm3 have a low risk of spontaneous bleeding if they are without evidence of other medical complications. If surgery is contemplated, however, a platelet count of 50,000 per mm3 is usually preferable. This guideline is empiric and has not been formally studied; hence, lower levels may be acceptable to the operating surgeon in specific clinical contexts. In children, the transfusion of 0.1 units of platelet concentrate for each kilogram of body weight may be anticipated to raise the platelet count to 40,000 per mm3.
No specific hemoglobin concentration or hematocrit level mandates blood transfusion in an oncologic surgical patient. Children with hemoglobin levels of 6 g per dL are often free of adverse physiologic sequelae. The specific clinical scenario governs the need for transfusion, as rapid blood loss, infection, pulmonary dysfunction, cardiomyopathy, and central nervous system compromise may all mandate early transfusion. Immunodeficient patients should receive irradiated blood products to avoid graft-versus-host disease, and if the patients are serologically cytomegalovirus-negative, they should receive cytomegalovirus-negative or leukocyte-poor blood products. Packed red blood cells are the usual blood product used for both acute blood loss and chronic anemia. Although whole blood is a suitable choice with massive hemorrhage, it is rarely available.
Elevations in prothrombin time (PT) and partial thromboplastin time (PTT) are acquired defects in most surgical patients. Vitamin K deficiency manifests with a marked prolongation of the PT level primarily, and normal PTT (unless significantly deficient) and normal platelet and fibrinogen levels. In older children, this may be due to malabsorption, dietary deficiency, or drug antagonism. The subcutaneous administration of vitamin K results in improvement within several hours and correction within a day. Fresh frozen plasma is required for immediate correction. If the platelet count and fibrinogen are also low, disseminated intravascular coagulation must be considered, particularly in patients with sepsis or
leukemia. Peripheral blood smears, platelet count, thrombin time, fibrin degradation products, fibrin monomers, and d-dimer levels are all useful in confirming the diagnosis. Treatment of disseminated intravascular coagulation consists of correction of the underlying disease and supportive care. If hemorrhagic manifestations are present without major thrombosis, fresh frozen plasma may be indicated. Liver disease with decreased synthetic capacity may also result in an elevated PT and PTT; however, in this instance correction is refractory to vitamin K.
leukemia. Peripheral blood smears, platelet count, thrombin time, fibrin degradation products, fibrin monomers, and d-dimer levels are all useful in confirming the diagnosis. Treatment of disseminated intravascular coagulation consists of correction of the underlying disease and supportive care. If hemorrhagic manifestations are present without major thrombosis, fresh frozen plasma may be indicated. Liver disease with decreased synthetic capacity may also result in an elevated PT and PTT; however, in this instance correction is refractory to vitamin K.
Evaluation of Anesthetic Risk Produced by an Anterior Mediastinal Mass
Respiratory collapse on induction of general anesthesia is a well-recognized complication of an anterior mediastinal mass (Fig. 12.1). Identification of patients at significant risk remains a major challenge. Orthopnea, respiratory stridor, and wheezing are all ominous signs of significant major airway obstruction (Fig. 12.2). Several patients with no respiratory symptoms, however, have been reported to develop major respiratory complications. The presence of congestion of the face and upper chest or venous distention suggests superior vena cava syndrome, which can be a prodrome of vascular collapse, but is rarely seen in the absence of significant airway compression.
Computed tomography (CT) is useful in defining the cross-sectional area of the trachea, and a reproducible technique for defining the tracheal area has been published.13 The cross-sectional area is measured from the CT slices showing the smallest tracheal area. The extent of narrowing is calculated by dividing the measured area by the established standard value and is expressed as the percentage of expected.14
The use of pulmonary function tests in evaluating children with an anterior mediastinal mass is also important. The predominant distortion of the flow loop for intrathoracic obstruction is a marked reduction in the maximum expiratory flow rate. For extrathoracic obstruction, it is a reduction of the maximum inspiratory flow rate. A fixed lesion usually produces an equal reduction of the inspiratory and expiratory peak flows. The high-flow portion of the forced expiratory loop near total lung capacity, best represented by the peak expiratory flow rate (PEFR), is the first to be distorted by an intrathoracic compression.
The PEFR can be easily obtained in patients by using a handheld device in the preoperative area or the emergency ward. A prospective evaluation of pulmonary function and tracheal area in 31 children with a mediastinal mass was performed before 34 surgical procedures.15 The tracheal area was determined by CT. In this study, criteria for administration of local anesthesia were either a tracheal area of less than 50% of predicted or a PEFR of less than 50% of predicted. All children administered anesthetics following these guidelines had an uneventful intraoperative course (Fig. 12.3). The study did not prove that children with a PEFR of less than 50% of predicted would experience respiratory collapse with general anesthesia, because all children who met this criterion were excluded from receiving general anesthesia. It did confirm, however, that general anesthesia could be used safely in children who met the minimum criteria of PEFR and tracheal area of greater than 50% of predicted. As treatment began and the masses shrank, the pulmonary function tests and total lung capacity improved. The patients who had a tracheal area of greater than 50% and yet a PEFR of less than 50% were found to have either larger masses or compression of the bronchus, which is difficult to quantitate. In a recent review of 55 children at another institution,
respiratory complications were found to occur only in children with a tracheal area of less than 30% or less than 70% with associated bronchial compression.16 Measurement of the PEFR may be the most accurate means of risk assessment and can be obtained in patients who are unable to lie flat.
respiratory complications were found to occur only in children with a tracheal area of less than 30% or less than 70% with associated bronchial compression.16 Measurement of the PEFR may be the most accurate means of risk assessment and can be obtained in patients who are unable to lie flat.
Among patients with Hodgkin and non-Hodgkin lymphoma (NHL), respiratory symptoms were more common in the children with NHL than in those with Hodgkin disease, and their pulmonary function was also more impaired.17 The NHL cohort had a disproportionate number of the larger masses, explaining their increased physiologic abnormalities. A correlation between the size of the mass and the impairment in pulmonary function was also noted. Other findings that correlated with acute airway compromise in 8 of 29 pediatric patients in an additional study included anterior location of the mass, histologic diagnosis of lymphoma, signs and symptoms of superior vena cava syndrome, radiographic evidence of vessel compression or displacement, pericardial effusion, and pleural effusion.18 In a third study of 117 pediatric patients with an anterior mediastinal mass, 11 experienced an anesthesia-related complication.19 Features associated with these complications included orthopnea, upper body edema suggesting superior vena caval syndrome, great vessel compression or compression of the main-stem bronchus, pleural effusion, tracheal compression, and the diagnosis of lymphoblastic lymphoma.
Inevitably, children will be encountered with significant respiratory compromise who require biopsy, but will not tolerate a general anesthetic. The presence of extrathoracic tissue for biopsy, particularly cervical lymphadenopathy, should be sought in all cases, but in some children it will be entirely lacking. It was demonstrated in the late 1990s that children with lymphoblastic lymphoma have a significantly higher incidence of an associated pleural effusion than do children with Hodgkin disease.20 Using cytologic and marker studies, this study and others also demonstrated that aspiration of the effusion was diagnostic of lymphoblastic lymphoma. Cytogenetic evaluation of cells obtained from pleural fluid can be helpful and diagnostic as well. The (t8;14) (q24;q11) translocation is particularly associated with T-cell lymphoblastic leukemia and the related T-cell lymphoblastic lymphomas, and its identification will facilitate their diagnosis.21 Immunophenotyping of the cells obtained from pleural fluid can also show a predominance of T-cell versus B-cell markers, supporting the diagnosis of lymphoblastic lymphoma.
Children with significant respiratory compromise and no pleural effusion for aspiration and no extrathoracic disease require an intrathoracic biopsy under local anesthesia, an image-guided percutaneous needle biopsy, or preliminary treatment with radiotherapy. Shielding of an area of the mass for future biopsy may allow successful diagnosis after the bulk of the mass and airway obstruction have resolved. Needle biopsies are often successful in establishing a diagnosis of NHL, but are less helpful in cases of Hodgkin disease. At our institution, biopsies under local anesthesia using an extrapleural anterior thoracotomy (Chamberlain procedure) have been performed successfully in four children as young as 10 years of age. This should be done with the children in a semi-upright position breathing spontaneously. The partially upright position will maximize the compromised respiratory function.16 The negative intrathoracic pressure exerted by the chest wall during spontaneous ventilation minimizes collapse of the trachea. By following these guidelines for the use of general anesthesia, and
the biopsy techniques described, a safe biopsy can be obtained in essentially all children and adolescents with an anterior mediastinal mass (Fig. 12.4). An algorithm for the care of children with a “critical airway” was proposed based on a recent review of 40 children.22 It was found that multiple children had the diagnosis established simultaneously when two procedures were performed, most often a bone marrow biopsy and biopsy of the mediastinal mass. Performing the bone marrow biopsy first would have avoided the risk for the more invasive mediastinal biopsy in these cases. While the use of extracorporeal membrane oxygenation (ECMO) or cardiopulmonary bypass has been described for near-total airway collapse, it can be avoided in almost all cases.23,24,25,26
the biopsy techniques described, a safe biopsy can be obtained in essentially all children and adolescents with an anterior mediastinal mass (Fig. 12.4). An algorithm for the care of children with a “critical airway” was proposed based on a recent review of 40 children.22 It was found that multiple children had the diagnosis established simultaneously when two procedures were performed, most often a bone marrow biopsy and biopsy of the mediastinal mass. Performing the bone marrow biopsy first would have avoided the risk for the more invasive mediastinal biopsy in these cases. While the use of extracorporeal membrane oxygenation (ECMO) or cardiopulmonary bypass has been described for near-total airway collapse, it can be avoided in almost all cases.23,24,25,26
MANAGEMENT OF PHEOCHROMOCYTOMAS/PARAGANGLIOMAS
Pheochromocytomas and paragangliomas (extra-adrenal pheochromocytomas) in children generally present with hypertension and associated symptoms. Once the diagnosis is confirmed by a 24-hour urine collection for catecholamines or by measurement of plasma free metanephrines (including chromogranin A levels), radiographic imaging is obtained to localize the tumor, which will include both axial (CT or magnetic resonance imaging [MRI]) and nuclear medicine (F18-positron emission tomography) imaging techniques to characterize the primary lesion in addition to localizing other such tumors.27 The definitive therapy is resection, but not before the patient has been carefully prepared for surgery. The principles of preoperative management are blood pressure control and the repletion of intravascular volume. α-Adrenergic blockade should begin at least 1 week before the surgery and is usually accomplished through the use of phenoxybenzamine. This is an oral long-acting α-adrenergic antagonist that is usually well tolerated by children. The typical starting dose for phenoxybenzamine is 0.20 mg per kg per day divided into a twice-daily dosing. More recently, doxazosin, a compound related to prazosin, was reported to be as effective as phenoxybenzamine, but with fewer complications.28 The intravenous administration of phentolamine, with its short half-life, may on occasion also be useful in controlling bouts of hypertension, especially intraoperatively. Effective α-blockade is established once orthostatic hypotension has been established, with improvement in the patient’s hypertension. This finding is secondary to the fact that patients with pheochromocytoma are intravascular volume-constricted because of the α-agonist effect on their vessels. As the α-agonists are blocked, adequate intravascular volume expansion is required to correct the fluid and red cell mass deficits and prevent reflex tachycardia. Weight-appropriate intravascular volume expansion utilizing 0.9% normal saline should be started concurrent with the α-blockade in the majority of patients. Beta-adrenergic blockade should be reserved for the treatment of persistent sinus tachycardia and arrhythmias associated with prior α-blockade despite intravascular repletion, and it should never precede α-blockade and saline administration.
Surgical resection of a pheochromocytoma produces a rapid drop in circulating catecholamines and possible hypotension, which may require further fluid administration in the perioperative period. Conversely, the induction of anesthesia or the manipulation of the tumor(s) during resection can result in hypertensive episodes that are best treated with intravenous sodium nitroprusside.
Careful perioperative planning must commence with the anesthesia service once the patient is diagnosed and pharmacologic therapy has begun.
Careful perioperative planning must commence with the anesthesia service once the patient is diagnosed and pharmacologic therapy has begun.
CANCER SURGERY
Staging
It is critical to establish the correct stage of a pediatric solid tumor for optimal treatment and outcome. Current protocols base therapy on the tumor stage, which determines such factors in treatment as the timing of surgery, use and extent of radiotherapy, and the intensity of chemotherapy. In an era when cure can be achieved in the majority of patients, efforts to limit therapy and minimize the long-term sequelae of treatment assume a prominent role, particularly in children with low-stage tumors. It has been demonstrated in several tumors that treatment of children based on inadequate or incorrect staging results in an increased incidence of relapse.29,30 Only in the management of Hodgkin disease has surgical staging decreased in importance as systemic therapy has been used uniformly.
The unique staging system for each of the solid tumors will be presented in the appropriate chapters. These staging systems are not static tools; rather, they have evolved over time as the significance of specific criteria for staging and treatment has been established. Hilar lymph node involvement in Wilms tumor was considered as a criterion for stage II in the first two National Wilms Tumor Study Group (NWTSG) protocols. On the basis of the demonstrated increased incidence of local recurrence in children with hilar lymph node involvement in the National Wilms Tumor Study-2, hilar lymph node involvement became a criterion in subsequent studies for stage III. A new staging system for neuroblastoma, the International Neuroblastoma Staging System (INSS), was created in the 1990s by a committee of international experts on neuroblastoma based on the results of prior studies.31 The treatment for neuroblastoma has of late progressed even further so that not only the stage but also the biologic markers of the tumor now determine the intensity of treatment in many protocols, and surgical risk factors are defined radiographically.32,33
The surgeon is critical to the proper assignment of stage. A surgical staging system is used for most pediatric solid tumors. In these systems, the extent of residual disease after resection as well as lymph node involvement determine local tumor stage. In children with Wilms tumor, lymph nodes must be sampled not only from the perihilar region, but also from along the aorta or vena cava to adequately stage the child. It is of note that it has been well established in Wilms tumor that assessment of lymph node involvement by gross inspection has a very poor correlation with the histology of the node. Othersen et al.34 demonstrated a false-negative rate of 31.3% and a false-positive rate of 18.1% in a prospective series. An increased incidence of local recurrence occurred in a recent review in children from NWTS-4 in whom lymph node sampling was not performed. The recurrence rate was actually higher than that of children with lymph node involvement who had been appropriately treated.30 The reason for the increased local recurrence was presumed under treatment of the children; the increased risk of recurrence occurred primarily in children with stage I disease who received limited chemotherapy and no radiotherapy.
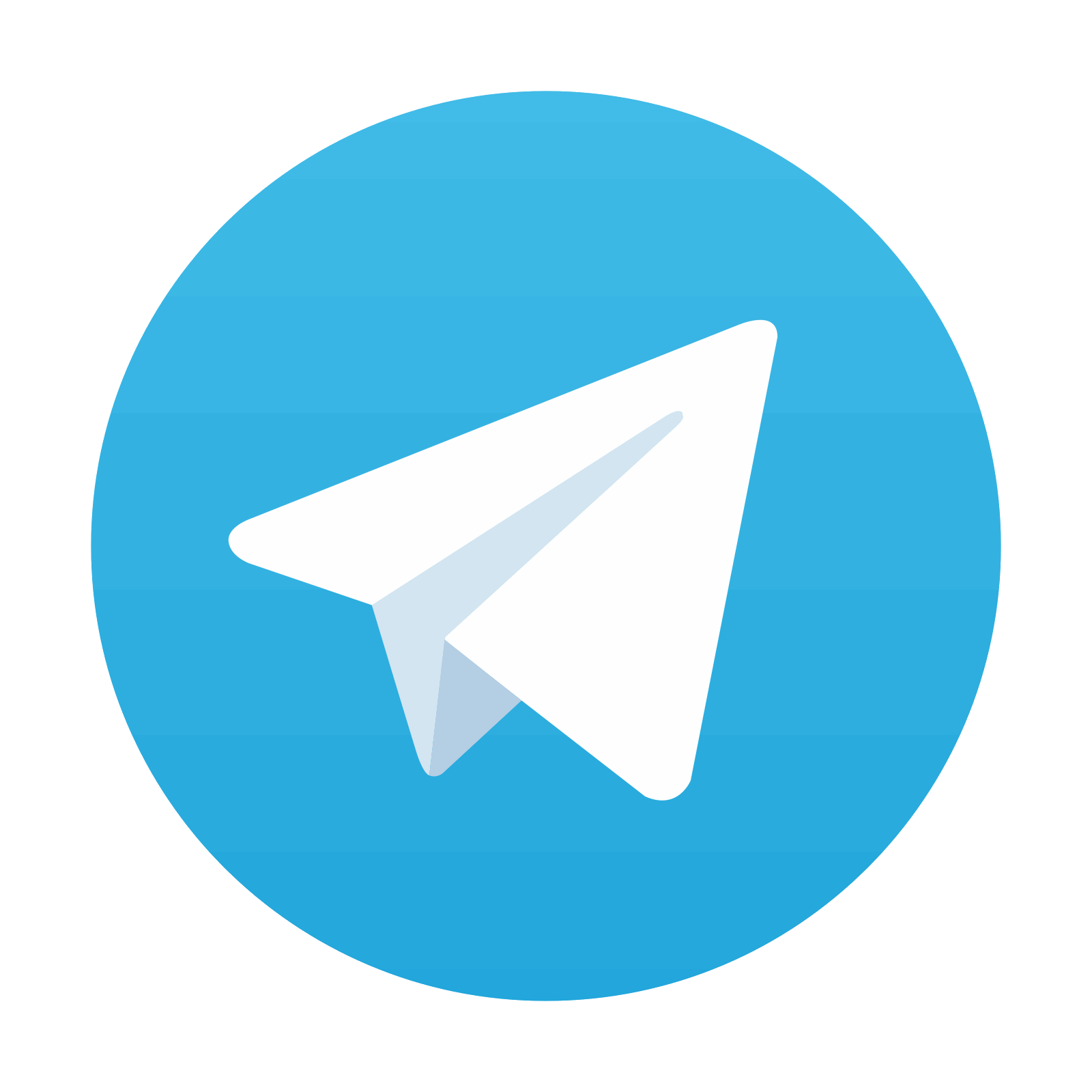
Stay updated, free articles. Join our Telegram channel

Full access? Get Clinical Tree
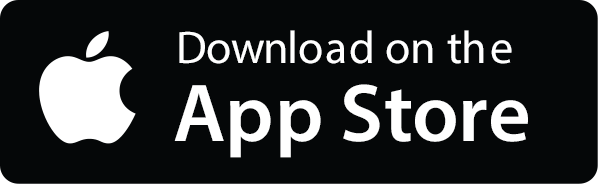
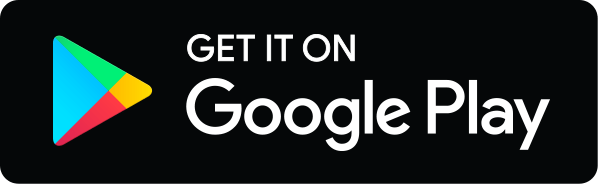