Fundamentals of Patient Management
MANAGEMENT OF THE PATIENT WITH CANCER
The optimal care of patients with malignant tumors is a multidisciplinary effort that combines the classic modalities of surgery, radiation therapy, and chemotherapy.
The role of the radiation oncologist is to assess all conditions relative to the patient and tumor, systematically review the need for diagnostic and staging procedures, and, in consultation with other oncologists, determine the best therapeutic strategy.
Radiation oncology is the clinical and scientific discipline devoted to the use of ionizing radiation in the management of patients with cancer (and other diseases), the investigation of the biologic and physical basis of radiation therapy, and the training of professionals in the field.
The aim of radiation therapy is to deliver a precisely measured dose of radiation to a defined tumor volume with minimal damage to surrounding healthy tissue.
PROCESS OF RADIATION THERAPY
The goal of therapy should be defined at the onset of therapeutic intervention:
Curative: There is a probability of long-term survival after adequate therapy; some side effects of therapy, although undesirable, may be acceptable.
Palliative: There is little hope of survival for extended periods. Symptoms producing discomfort or an impending condition that may impair comfort or self-sufficiency require treatment. Relatively high doses of irradiation (sometimes 75% to 80% of curative dose) are required to control the tumor for the survival period of the patient.
BASIS FOR PRESCRIPTION OF IRRADIATION
Evaluation of tumor extent (staging), including diagnostic studies.
Knowledge of pathologic characteristics of the disease.
Definition of the goal of therapy (cure or palliation).
Selection of appropriate treatment modalities (irradiation alone or combined with surgery, chemotherapy, or both).
Determination of optimal dose of irradiation and volume to be treated, according to anatomic location, histologic type, stage, potential regional nodal involvement (and other tumor characteristics), and normal structures in the region.
Evaluation of patient’s general condition, plus periodic assessment of tolerance to treatment, tumor response, and status of normal tissues treated.
The radiation oncologist must work closely with physics, treatment planning, and dosimetry staffs to ensure greatest accuracy, practicality, and cost benefit in the design of treatment plans.
The ultimate responsibility for treatment decisions, technical execution of therapy, and consequences of therapy always rests with the radiation oncologist.
RADIATION TREATMENT PLANNING
Different radiation doses are required for given probabilities of tumor control, depending on the tumor type, the initial number of clonogenic cells present, the extent of disease to be treated (2), and the inclusion or exclusion of additional therapeutic modalities (e.g., surgery and/or chemotherapy) in the overall treatment plan.
International Commission on Radiation Units and Measurements Reports Nos. 50 and 62 define the following treatment planning volumes (7, 8):
Gross tumor volume (GTV): All known gross disease, including abnormally enlarged regional lymph nodes. To determine GTV, appropriate computed tomography (CT) window and level settings that give the maximum dimension of what is considered potential gross disease should be used.
Clinical target volume (CTV): Encompasses GTV plus regions potentially harboring microscopic disease.
Planning target volume: Provides margin around CTV to allow for internal target motion, other anatomic motion during treatment (e.g., respiration), and variations in treatment setup. This does not account for treatment machine beam characteristics.
Treatment portals must adequately cover all treatment volumes plus a margin to account for beam physical characteristics, such as penumbra (Fig. 1-1).
Simulation is used to accurately identify target volumes and sensitive structures, and to document configuration of portals and target volume to be irradiated.
Treatment aids (e.g., shielding blocks, molds, masks, immobilization devices, compensators) are extremely important in treatment planning and delivery for optimal dose distribution. Repositioning and immobilization devices are critical because effective irradiation is that which accurately hits the clonogenic tumor cells.
Simpler treatment techniques that yield an acceptable dose distribution are sometimes preferred over more costly and complex ones, which may have a greater margin of error in day-to-day treatment.
Accuracy is periodically assessed with portal (localization) films or on-line (electronic portal) imaging verification devices, which may be 2-D (e.g., port films) or 3-D (e.g., on-board kV or MV cone-beam CT imaging) based systems. Portal localization errors may be systematic or may occur at random.
Three-Dimensional Treatment Planning
CT simulation allows more accurate definition of target volume and anatomy of critical normal structures, three-dimensional (3-D) treatment planning to optimize dose distribution, and radiographic verification of volume treated (12, 14).
Advances in computer technology have augmented accurate and timely computation, display of 3-D radiation dose distributions, and dose-volume histograms that yield relevant information for evaluation of tumor extent, definition of target volume, delineation of normal tissues, virtual simulation of therapy, generation of digitally reconstructed radiographs, design of treatment portals and aids, calculation of 3-D dose distributions and dose optimization, and critical evaluation of the treatment plan (15).
Dose-volume histograms are useful in assessing several treatment plan dose distributions and provide a complete summary of the entire 3-D dose matrix, showing the amount of target volume or critical structure receiving more than the specified dose. They do not provide spatial dose information and cannot replace other methods of dose display.
3-D treatment planning systems play an important role in treatment verification. Digitally reconstructed radiographs based on sequential CT slice data generate a simulation film that can be used in portal localization and for comparison with the treatment portal film for verifying treatment geometry.
Increased sophistication in treatment planning requires parallel precision in patient positioning and immobilization, as well as in portal verification techniques (17). Several real-time, on-line verification systems allow monitoring of the area to be treated during radiation exposure.
Computer-aided integration of data generated by 3-D radiation treatment planning with parameters used on the treatment machine, including gantry and couch position, may decrease localization errors and enhance the precision and efficiency of irradiation.
Intensity-Modulated Radiation Therapy
Intensity-modulated radiation therapy (IMRT), a relatively newer approach to 3-D treatment planning and conformal therapy, optimizes delivery of irradiation to irregularly shaped volumes through complex forward or inverse treatment planning and results in modulated fluence of multiple photon beam profiles.
Inverse planning starts with an ideal dose distribution and results in, through trial and error or multiple iterations (simulated annealing), the desired beam characteristics (fluence profiles). It produces the best approximation to the ideal dose defined in a 3-D array of dose voxels organized in a stack of 2-D arrays.
Approaches to IMRT include the following:
The step-and-shoot method, which employs a linear accelerator and multileaf collimator that breaks each treatment field into a set of smaller subfields. Each subfield is delivered one at a time in a predefined sequence. After a subfield is treated, the beam is shut off, the MLC leaves are repositioned for the next subfield and the beam is turned on again.
Dynamic computer-controlled IMRT is delivered when the configuration of the portals with the MLC changes at the same time that the gantry or accelerator changes positions around the patient.
In helical tomotherapy, a photon fan beam continually rotates around the patient as the couch transports the patient longitudinally through a ring gantry (9). The ring gantry enables verification processes for helical tomotherapy; the geometry of a CT scanner allows tomographic processes to be reliably performed. Dose reconstruction is a key process of tomography; the treatment detector sinogram computes the actual dose deposited in the patient. The lengths of the MLC in helical tomotherapy are temporally modulated or binary because they are rapidly driven either in or out by air system actuators rather than by beams slowly pushed by motors driving lead screws, as in the conventional MLC.
The robotic arm of the IMRT system Cyberknife (Accuray, Sunnyvale, CA) consists of a miniaturized 6-MV photon linear accelerator mounted on a highly mobile arm and a set of ceiling-mounted x-ray cameras to provide near real-time information on patient position and target exposure during treatment.
The majority of IMRT systems use 6-MV x-rays, but energies of 8 to 10 MV may be more desirable in some anatomic sites to decrease skin and superficial subcutaneous tissue dose.
PROBABILITY OF TUMOR CONTROL
Various levels of irradiation yield different probabilities of tumor control, depending on the histology and number of clonogenic cells present. Numerous dose response curves for a variety of tumors have been published with higher doses of irradiation producing better tumor control.
For every increment of irradiation dose, a certain fraction of cells will be killed; the total number of surviving cells is proportional to the initial number present and the fraction killed with each dose (5).
For subclinical disease (deposit of tumor cells too small to be detected clinically or even microscopically), doses of 45 to 50 Gy will result in disease control in more than 90% of patients (6).
Microscopic tumor, such as at the surgical margin, is not subclinical disease; cell aggregates 106 per cm3 or greater are required for the pathologist to detect them. These volumes must receive higher doses of irradiation (e.g., 60 to 65 Gy in 6 to 7 weeks) (6).
For clinically palpable tumors, doses of 65 (for T1 tumors) to 75 to 80 Gy or even higher (for T4 tumors) are required (1.8 to 2.0 Gy per day, five fractions weekly) (6).
A boost is an additional dose administered through small portals to residual disease; it is given to obtain a similar probability of control as for subclinical aggregates.
Portals can be progressively reduced in size (i.e., the “shrinking-field” technique) to administer higher doses to the central portion of the tumor, where more clonogenic cells (presumably hypoxic) are present, in contrast to the smaller doses required to eradicate disease in the periphery, where a lower number of better oxygenated tumor cells are assumed to be present.
NORMAL TISSUE EFFECTS
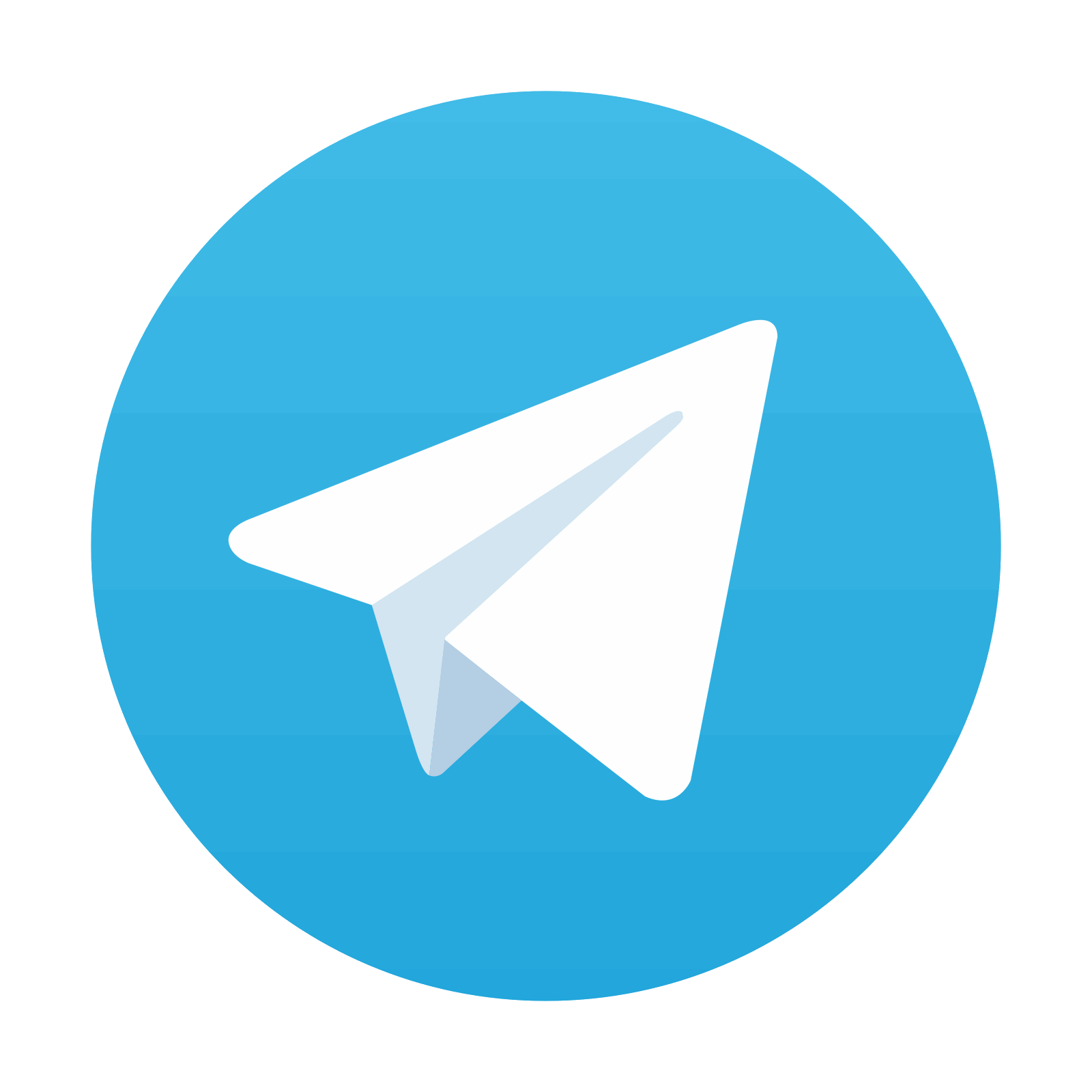
Stay updated, free articles. Join our Telegram channel

Full access? Get Clinical Tree
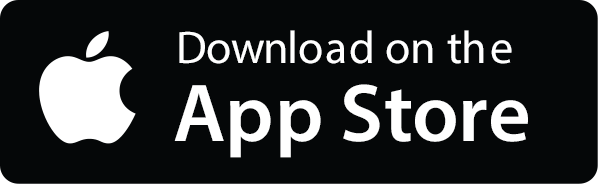
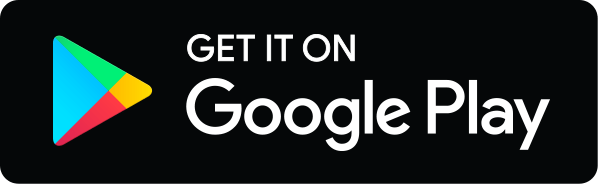