Fig. 7.1
Types of exercise and their associated glucose changes
The greatest acute hypoglycemia risk appears to be for mild-to-moderate-intensity aerobic exercise, especially when performed soon after a meal when circulating insulin levels are typically elevated [26]. Mild-to-moderate-intensity afternoon exercise (i.e., walking/jogging at a heart rate of ~140 bpm) performed in the postabsorptive state with little “on board” bolus insulin in the circulation also promotes a gradual decrease in glycemia in a majority of youth with T1D [27]. This type of activity, particularly if performed in the afternoon, increases risk for overnight and next day hypoglycemia by ~30 % [28]. In contrast, intermittent type exercise, often observed in team sports, increases counterregulatory hormones and lactate levels and is associated with less hypoglycemia, although a drop in glycemia is still often observed if the activity is prolonged [29, 30]. In contrast, a short but intense sprint promotes a sustained (>2 h) rise in glucose concentrations by 1–2 mmol/L in youth who are euglycemic before the exercise [31]. Thus, hyperglycemia is possible, and even likely, with intense aerobic or anaerobic exercise, especially if the pre-exercise glucose is already elevated [32]. In addition, if pre-exercise insulin doses are adjusted down excessively, or if excessive preemptive carbohydrate intake occurs, then hyperglycemia may also result [33]. Finally, resistance exercise (weight lifting) may increase glucose levels if done briefly [34, 35] or may be associated with little glucose change as compared to aerobic exercise [36], although it may increase postexercise late-onset hypoglycemia risk, perhaps as muscle glycogen stores are being replenished [36]. Brief bouts of resistance exercise have been shown to increase glycemia by ~2–3 mmol/L in well-controlled patients with T1D, while more prolonged efforts are associated with better blood glucose stability [34, 35, 37].
In general, reductions in basal (typically for CSII patients only) and or bolus insulin doses, with or without carbohydrate supplementation, are recommended for youth with T1D for prolonged aerobic activities like cycling and running, while intermittent sports and games and resistance-type activities typically require less modifications to insulin and carbohydrate intake [5].
Severe hypoglycemia is most often identified as the result of exercise [38] and typically occurs during or immediately after exercise (early hypoglycemia) or when the child is asleep (delayed nocturnal hypoglycemia) [39]. Acutely, exercise increases glucose disposal into skeletal muscle via both insulin-dependent and insulin-independent mechanisms [40], while delayed hypoglycemia may occur secondary to a prolonged increase in insulin sensitivity [41], reduced counterregulatory responses, and/or a lack of adjustment to delivered exogenous insulin [39].
Afternoon exercise (such as after school sports activities) between 4 p.m. and 6 p.m. is associated with hypoglycemia during the middle of the night when blood glucose monitoring is less frequent [42]. Basal rate suspension during exercise (for those youth on insulin pump therapy) is effective in attenuating the drop in glycemia during exercise, although it may still drop significantly during the first 20–30 min, and postexercise glucose levels may be in the hyperglycemic range [43]. Moreover, children using an insulin pump can reduce the risk of nocturnal hypoglycemia by suspending the basal insulin rate during exercise and then lowering the nocturnal basal rate by 20 % between 9 p.m. and 3 a.m. [39]. Because of this flexibility around basal rate reductions (or increases where necessary), the use of an insulin pump should be considered advantageous for active youth with T1D. CSII has been shown to be safe and effective for use by the pediatric population [44]. Unfortunately there is a paucity of data to inform basal/long-acting insulin adjustments in youth on multiple daily injections. A decision tree for starting point adjustments for exercise is provided in (Fig. 7.2).


Fig. 7.2
Starting point decision tree for exercise adjustments for adolescent patients with type 1 diabetes
7.5 Mechanisms of Exercise-Associated Hypoglycemia
7.5.1 Relative Hyperinsulinemia
During prolonged aerobic exercise in the nondiabetic patient, hepatic glycogen breakdown and gluconeogenesis restore glucose taken up from the circulation into the working muscle, thereby preventing hypoglycemia [45]. Appropriately decreased insulin secretion, acutely sensitive to changes in glucose concentration, and release of counterregulatory hormones facilitate this process. The rapid fall in insulin concentration in the portal circulation sensitizes the liver to glucagon, which rises as exercise continues, thereby allowing for a rapid increase in hepatic glucose production at the onset of exercise [46].
However, during exercise in T1D, glucose production by the liver is impaired because the insulin in the portal circulation does not fall after the exercise starts [45] and may even rise significantly because of increased subcutaneous blood flow [25]. Also, glucose uptake into skeletal muscle increases linearly with increased muscular effort [40] and increases with exercise duration [47]; hence hypoglycemia risk is most significant when the intensity is moderate/vigorous for a prolonged duration. Youth with T1D have been shown to have relatively normal early counterregulatory responses (glucagon, cortisol, growth hormone [GH], glucagon, adrenaline, and noradrenaline) to steady-state endurance, progressive aerobic and interval-type exercise, but relative hyperinsulinemia [29]. Overall, insulin levels are typically elevated two- to threefold above nondiabetic control subjects during aerobic exercise [48] but may be too low in early recovery following intense activities [32].
7.5.2 Counterregulatory Failure
In addition to the relative hyperinsulinemia described above, a number of other endocrine disturbances may exist in the young patient with T1D that place them at an increased risk for exercise-associated hypoglycemia, particularly delayed nocturnal hypoglycemia. Some recent evidence suggests that post prandial glucagon levels may be abnormally high in young adult T1D, but the rise in glucagon during exercise may be blunted [25]. Although glucose counterregulatory hormone responses have been reported to be normal during exercise in the pediatric patient population (see above), the counterregulatory response during exercise in the face of developing hypoglycemia may be impaired [29, 49, 50]. Indeed, young children and adolescents with T1D have blunted counterregulatory hormone responses to delayed, and especially nocturnal, hypoglycemia, even in the presence of target overall glycemic control [50]. A recent episode of either exercise or hypoglycemia may further impair the counterregulatory responses to hypoglycemia [51]. A reduced capacity to mobilize liver glycogen because of poor glycemic control may also contribute to exercise-associated hypoglycemia in the young patient with type 1 diabetes [52, 53].
7.6 Mechanisms for Exercise-Associated Hyperglycemia
Hyperglycemia may be caused by a number of factors including aggressive insulin reduction, insulin omission, and/or carbohydrate overconsumption for fear of hypoglycemia [54]. Intense aerobic (≥80 % VO2max) [32] and anaerobic [31, 54] exercise can also increase glycemia in early recovery. Importantly, this rise in glucose is associated with a rise in circulating insulin levels in nondiabetics to rapidly restore glucose homeostasis [32, 54]. Sprinting for just 10 s at maximal effort or cycling at maximal effort for 30 s increases glucose concentrations by about 1–2 mmol/L [31, 54]. This type of exercise is fueled primarily through anaerobic metabolism (i.e., lactate production from muscle glycogen breakdown) and is associated with an increase in plasma catecholamine levels which limit glucose uptake into muscle [31].
7.7 Strategies for Different Types of Exercise
7.7.1 Aerobic
As mentioned above, light-to-moderate-intensity aerobic exercise performed in the afternoon causes a significant drop in a majority of children with T1D, with ~1/3 requiring carbohydrate supplementation to treat hypoglycemia [27]. Having a pre-exercise blood glucose (BG) <120 mg/dL (6.7 mmol/L) prior to exercise increases the risk of developing hypoglycemia [27, 55]. In contrast, having a blood glucose level at the start of exercise >150 mg/dL (8.3 mmol/L) largely prevented hypoglycemia at least during a 75 min period, although a large drop in glycemia may still be observed. In fact, in one study [27], several youth, who began aerobic exercise at a glucose concentration >180 mg/dL (10 mmol/L), experienced decreases in blood glucose by >100 mg/dL (5.5 mmol/L) during the hour of exercise.
The “safe” glucose range for prolonged mostly aerobic activities (walking, running, swimming, individual and team sports), according to our perspective based on the research available to date, is between 90 and 250 mg/dL (5.0 and 13.9 mM), depending on the timing of exercise relative to the last meal and insulin dose. Anything above or below this wide range should be considered detrimental from an exercise performance and safety perspective.
In situations of pre-exercise hyperglycemia (blood glucose concentration ≥14 mM/250 mg/dL), aerobic exercise can be initiated with some caution as long as blood ketone levels are not elevated, although a conservative insulin correction may be considered. Youth with T1D, as detailed above, demonstrate considerable decreases in blood glucose concentration during exercise even if starting glucose concentrations are between 14 and 22 mM and no bolus insulin “correction” is taken prior to the start of exercise [27, 55]. Only if insulin has been withheld for prolonged periods (e.g., skipped insulin administration, pump basal insulin interruption due to infusion set malfunction, intentionally prolonged pump disconnection) will glucose levels likely rise during purely aerobic exercise performed in the fasting/post meal absorptive state [56].
In general we advise that, before aerobic exercise, appropriate meal planning includes adequate sources of both complex carbohydrate and protein up to 3 h before aerobic exercise. Where exercise occurs less than 3 h after a meal, the kinetics of rapid-acting insulin analogues (aspart, lispro, glulisine) are such that a dose reduction should be considered to help minimize active insulin during exercise, which otherwise further increases the risk of exercise-induced hypoglycemia. Some authors have shown that extra pre-exercise meal protein (an average of 30 g 2 h prior to aerobic exercise in adolescents with T1D) decreases hypoglycemia during aerobic exercise, albeit not as effectively as consuming an immediate pre-exercise carbohydrate snack [57].
In addition to reductions in mealtime insulin, or to increased carbohydrate/protein feeding for the prevention of exercise-induced hypoglycemia, reductions in basal insulin can also help limit the fall in glycemia during exercise. Where this is an option (i.e., in those using an insulin pump), basal rate suspension at the start of exercise significantly reduced hypoglycemia in the study performed by the DirecNet group, although some postexercise/recovery hyperglycemia was observed [43]. In silico studies modeling the effects of aerobic exercise on glucose uptake and insulin sensitivity show a basal rate reduction before exercise (30–90 min before) to be even more effective in addressing the acute effect of higher glucose disposal induced during and after exercise [58]. Depending on baseline glucose level, food intake, and duration of activity, we suggest a 20–50 % basal rate reduction up to 90 min before the start of aerobic activity and 50–80 % reduction during the exercise with a complete suspension if the glucose drops below 90 mg/dL (5 mmol/L).
Where basal insulin delivery is not easily modifiable over short periods of time (i.e., in the case of those on long-acting basal analogues such as glargine or detemir), very little data exists to inform basal adjustment, and the effects of basal reduction to avoid acute hypoglycemia may increase rates of hyperglycemia later or even the following day. Thus, in these youth a planned strategy combining minimizing rapid-acting “insulin on board” by avoiding boluses of insulin near to the time of exercise must be combined with a carbohydrate focused strategy to balance substrate delivery with glucose uptake. The ISPAD guidelines [59] provide useful tables for activity and weight-based carbohydrate intake to preserve euglycemia. Where no adjustment to premeal insulin delivery has occurred, up to 1 g of CHO per kg of body weight, dispersed throughout the exercise period, may be required. Where pre-exercise insulin has been reduced as above, then 0.3–0.5 g/kg per hour will likely be sufficient.
7.7.2 Mixed and Anaerobic Exercise
As mentioned above, not all forms of exercise cause glucose levels to drop. In fact, many forms of team and individual sports and/or sport competition may cause glucose to rise. For example, 10–15 min of high-intensity cycling (>80 % VO2peak) to volitional exhaustion performed under basal insulin conditions causes a significant (~40 mg/dL, 2.3 mmol/L) rise in blood glucose levels in adults with type 1 diabetes [60, 61]. This prolonged hyperglycemia post aerobic exercise is gradually restored over several hours post exercise if insulin is administered (i.e., in this particular study) [62]. Similarly, with just 10 s of maximal sprinting in a cycle ergometer, glucose levels rise and stay elevated for a prolonged period (>2 h) in adolescents with T1D [54]. Performing a sprint before [63] or after [64] prolonged aerobic exercise helps attenuate the hypoglycemic effect of the aerobic exercise. Thus, a brief maximal sprint may serve as another tool to help eliminate exercise-induced hypoglycemia, even in those prone to the condition because of antecedent hypoglycemia [65].
How to best manage postexercise hyperglycemia caused by intense sprint-based exercise or by other intense activities is currently unclear, although some conservative insulin correction may be warranted. As a practical point, these authors generally recommend using approximately 50 % of the usual correction dose for postexercise hyperglycemia and only if hyperglycemia persists for >60 min postexercise. In many young athletes with T1D, early postexercise hyperglycemia rapidly wanes. Excessive early postexercise correction boluses of insulin may peak simultaneously with the natural resolution of a transient hyperglycemic “spike,” leading to hypoglycemia. We recognize, though, that this general (and still individualized) approach is based on clinical experience rather than firm clinical data.
7.8 Postexercise Late-Onset Hypoglycemia
With just 30 min of moderate-to-vigorous activity, adolescents and young adults with T1D are at increased risk for hypoglycemia the following evening (by ~40 %) and the next day (by about 30 %) [28]. A greater volume of exercise further increases risk [28]. A higher hypoglycemic risk may occur with late-day exercise as compared to morning exercise [66]. Moreover, afternoon or early evening exercise is associated with a biphasic change in insulin sensitivity, such that heightened insulin sensitivity occurs during the exercise and again 7–11 h later during sleep [41]. Notably, if exercise is performed earlier in the day, rather than in the afternoon, it is associated with sustained insulin sensitivity in recovery for about 11 h [67].
Glucose utilization (GU) during exercise is subject to complex regulation by insulin, plasma glucose, alternate substrates, other humoral factors, and muscle factors. At lower exercise intensities, plasma glucose is normally regulated to remain constant during postabsorptive exercise and declines during postprandial exercise (and often more so in persons with diabetes). During such exercise, insulin secretion is inhibited by beta-cell alpha-adrenergic receptor activation. In contrast, in intense exercise (>80 % VO2max), glucose production (GP) rises seven- to eightfold and GU rises three- to fourfold; therefore, glycemia increases, and plasma insulin decreases minimally, if at all [32]. In intense exercise, unlike at lesser intensities, carbohydrate (i.e., muscle glycogen and blood glucose) is the main muscle fuel. It must be mobilized from muscle and liver glycogen in both the fed and fasted states. At exhaustion, GU initially decreases more than the decrease in GP, which leads to greater hyperglycemia, requiring a substantial rise in insulin for 40–60 min to restore pre-exercise glucose levels. Absence of this response in type 1 diabetes leads to sustained hyperglycemia, and mimicking it by intravenous infusion restores the normal response [62]. Compelling evidence supports the conclusion that the marked catecholamine responses to intense exercise are responsible for both the GP increment (that occurs even during glucose infusion and postprandially) and the restrained increase of GU. These responses are normal in persons with type 1 diabetes, who often report exercise-induced hyperglycemia, and in whom the clinical challenge is to reproduce the recovery period hyperinsulinemia.
7.9 Modern Glucose Monitoring Management
Insulin pump therapy is associated with lower rates of severe hypoglycemia [68]—a common fear for both parents and children with T1D [8]. Continuous glucose monitoring (CGM) can assist children and adolescents in self-monitoring of glucose levels, while detecting time-based changes and trends in glucose levels [69]. During exercise, stress hormones increase and influence glucose metabolism, and changes are often rapid and unpredictable. By continuously monitoring glucose levels, these devices show potential to improve glucose control during exercise [70]. Automatic suspension of insulin delivery in insulin pumps, facilitated by CGM, appears to offer some prevention of postexercise nocturnal hypoglycemia, although threshold settings may need to be adjusted if a recent bout of hypoglycemia has occurred [71]. Improved glucose data in real time with a predictive element may help inform appropriate measures to avoid immediate post-activity, as well as delayed, hypoglycemia.
Conclusion
With the prevalence of pediatric diabetes increasing globally, it is important for both children and parents to be aware of the benefits that exercise can confer and equally that T1D not be seen as an inherent major barrier to participation in exercise programs and incidental play that is both fun and health promoting. Children, adolescents, and their care providers should be focused on the attainment of at least 60 min of moderate-to-vigorous PA daily to achieve health benefits while at the same time focus on strategies to minimize glucose dysregulation.
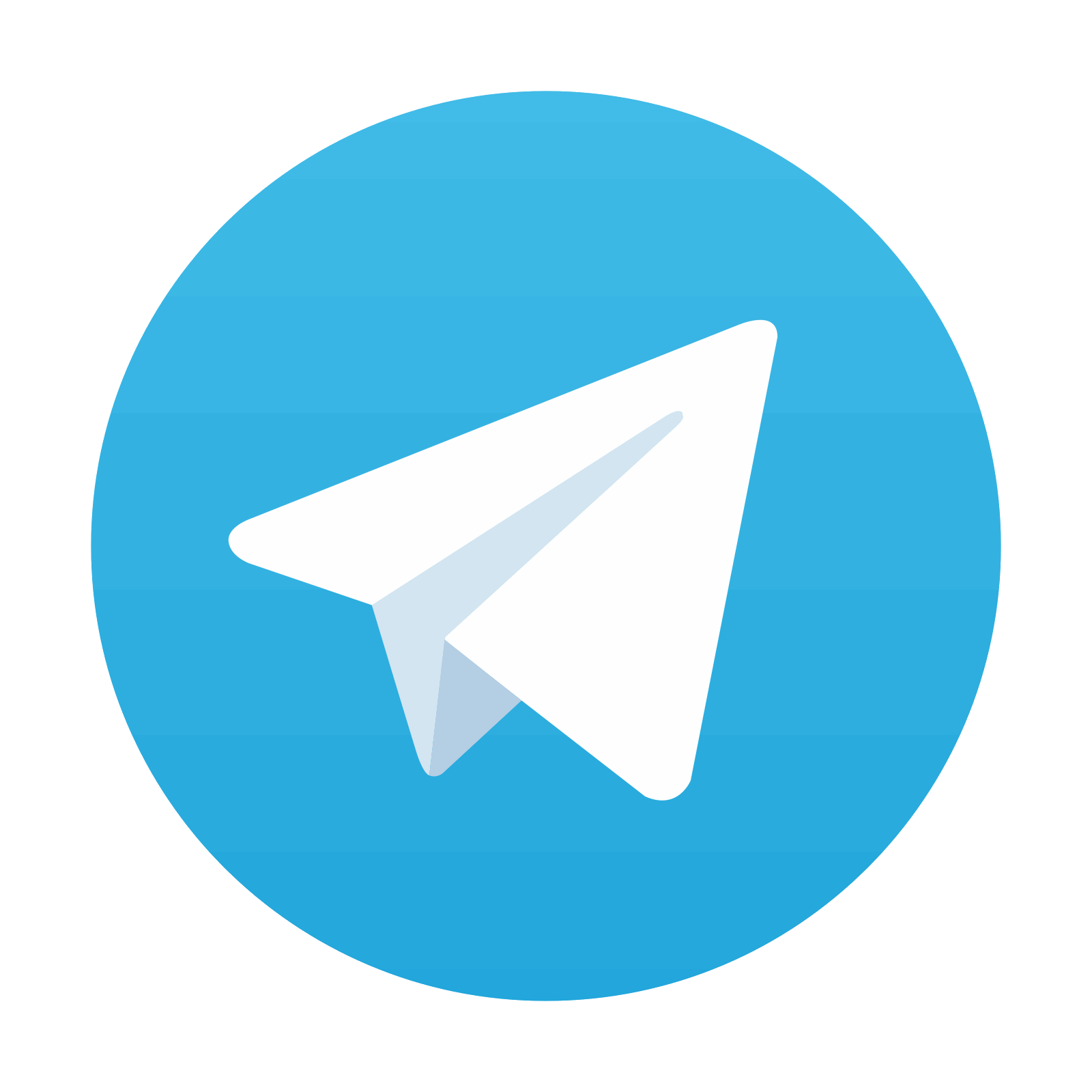
Stay updated, free articles. Join our Telegram channel

Full access? Get Clinical Tree
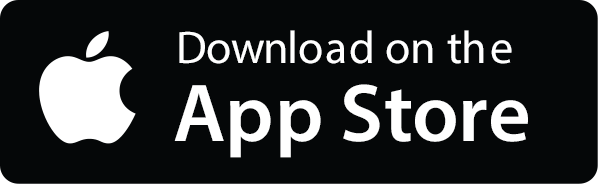
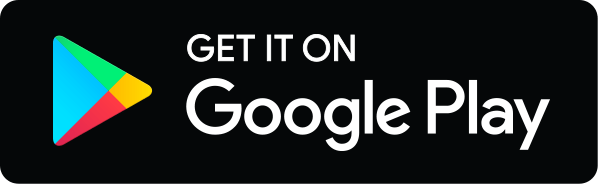