CHAPTER 18 Emerging Infectious Diseases of Immigrant Patients
Introduction
It is understandable that a sense of complacency about infectious disease took hold of medicine and public health in the late twentieth century. Improvements in sanitation, nutrition, housing, and occupational health dramatically decreased infectious disease rates in the United States. Immunizations were effective against viral infections: smallpox was eradicated world-wide; rubella was eliminated from North America; and rates of other childhood viral diseases markedly reduced.1,2 With the development of safe and effective vaccines and drugs to treat bacterial infections, bacteria appeared defeated. All these factors caused an inappropriate confidence that infectious diseases would be completely eradicated as a public health problem. In fact, in 1967, US Surgeon General William H. Stewart announced that it was ‘time to close the book on infectious diseases, declare the war against pestilence won, and shift national resources to such chronic problems as cancer and heart disease.’3 Despite this hubris, others began to sound the alarm. The term ‘emerging diseases’ was first used by David J. Sencer in 1971.4 In 1976, the Centers for Disease Control (CDC) investigated an outbreak of disease affecting attendees at the National American Legion Convention in Philadelphia, and the following year CDC isolated the causative agent, Legionella pneumophila, for what is now called Legionnaire’s disease. In 1981, Richard M. Krause, director of the National Institutes of Allergy and Infectious Diseases, published an early clarion call with his book The Restless Tide: The Persistent Challenge of the Microbial World.5 Shortly thereafter, the epidemic of AIDS was recognized and over the next 10 years a growing sense of unease arose as new infectious disease outbreaks were identified.
Recognition and Surveillance for Emerging Infectious Disease
The Institute of Medicine triggered a landslide of interest in emerging infections when it addressed the issue in the early 1990s. In the seminal work on the topic, a 1992 Institute of Medicine report defined emerging infectious diseases as ‘infections that have newly appeared in a population or have existed but are rapidly increasing in incidence or geographic range.’6 Aside from numerous academic and lay-public publications, several landmark developments are notable in the history of emerging infections. The Centers for Disease Control and Prevention (CDC) started publication of the journal Emerging Infectious Diseases in January 1995. The journal continues to provide a venue for discussion of emerging diseases in human and animal populations.
ProMED-mail, the Program for Monitoring Emerging Diseases, (http://www.promedmail.org) is an internet-based reporting system established in 1994 with the support of the Federation of American Scientists and SatelLife. Since 1999, ProMED-mail has operated as a program of the International Society of Infectious Diseases. The electronic mail system provides subscribers with daily updates about emerging diseases from around the world. The importance and effectiveness of the system has been repeatedly documented. A notable example was an email sent by a travel medicine physician, Stephen O. Cunnion, on February 10, 2003.7 He quoted an email that he had received, stating, ‘Have you heard of an epidemic in Guangzhou? An acquaintance of mine from a teacher’s chat room lives there and reports that the hospitals there have been closed and people are dying.’ This email was an early warning of an outbreak of the previously unidentified human coronavirus causing severe acute respiratory syndrome (SARS).
The International Society of Travel Medicine (ISTM) provided seed money to establish GeoSentinel in July 1995. Initially, GeoSentinel was founded as a working group of nine US-based ISTM member travel clinics which agreed to collaborate as a sentinel emerging infections network by monitoring illness among returning international travelers. The following year the network was awarded funding from the CDC. GeoSentinel now incorporates a global network of providers at over 30 sites on all continents. A successful early recognition of disease emergence by GeoSentinel was the identification of leptospirosis among participants in the Borneo Eco-Challenge 2000 Adventure Race while many participants were still in the incubation period.8
Historical Observations: Plagues, Pestilences, People, Immigration
The opening of the New World to European settlement led to an interchange of species, including pathogens, termed the Columbian exchange.9 Measles and smallpox brought to the New World by immigrant Europeans devastated Native Americans. Meanwhile, syphilis, which is theorized to have arisen in the Americas, spread in epidemic fashion in Europe after 1492. The slave trade was responsible for the movement of HTLV (human T-cell lymphotropic virus)-I, HTLV-II, yellow fever virus and, importantly, its mosquito vector, Aedes aegypti, to the Americas. In the 1970s, Aedes albopictus, a mosquito vector competent for transmission of dengue was inadvertently imported into North America from Asia as a result of the international trade in used tires. Global air travel has connected distant corners of the planet like never before, offering pathogens unprecedented potential for rapid transmission to far-flung immunologically naïve populations.
Epidemiology and Modeling of Emerging Infectious Diseases
The first link in the chain, the source for the agent, is the place where the agent originates. This may be another infected human, or the animal reservoir in the case of zoonotic infections, or the environmental reservoir in the case of pathogens acquired from environmental sources, such as soil. Influenza, for example, circulates as a zoonotic infection with the principle reservoir being waterfowl.
Once the agent leaves the source, a mode of transmission, or means of carrying it to the host, is needed. Although in the case of influenza fomite spread plays a role, with humans carrying the virus from poultry farm to farm, the main route of transmissions from person to person tends to be droplet spread of infected respiratory material. Other modes of transmission are shown in Box 18.1.
There must be a pathway into the host, a portal of entry, which gives the agent access to tissue where it can multiply or act. Often, the agent enters the host in the same way that it left the source. This is the case with influenza or Mycobacterium tuberculosis, which leave the source through the respiratory tract and usually enter a new host through the respiratory tract.
Yet even when all of the above factors are present, some diseases do not become epidemics. Mathematical modeling of infectious disease outbreaks has led to the characterization of the basic reproduction number, R0, which describes the average number of secondary cases of disease generated by each typical case in a susceptible population. For epidemic spread of a disease, the R0 for the pathogen must be greater than one (R0 > 1). In its most simplistic form, the SIR model divides the population into proportions corresponding to susceptible (S), infected (I), and removed (either recovered and immune, or dead) (R).10 By definition:
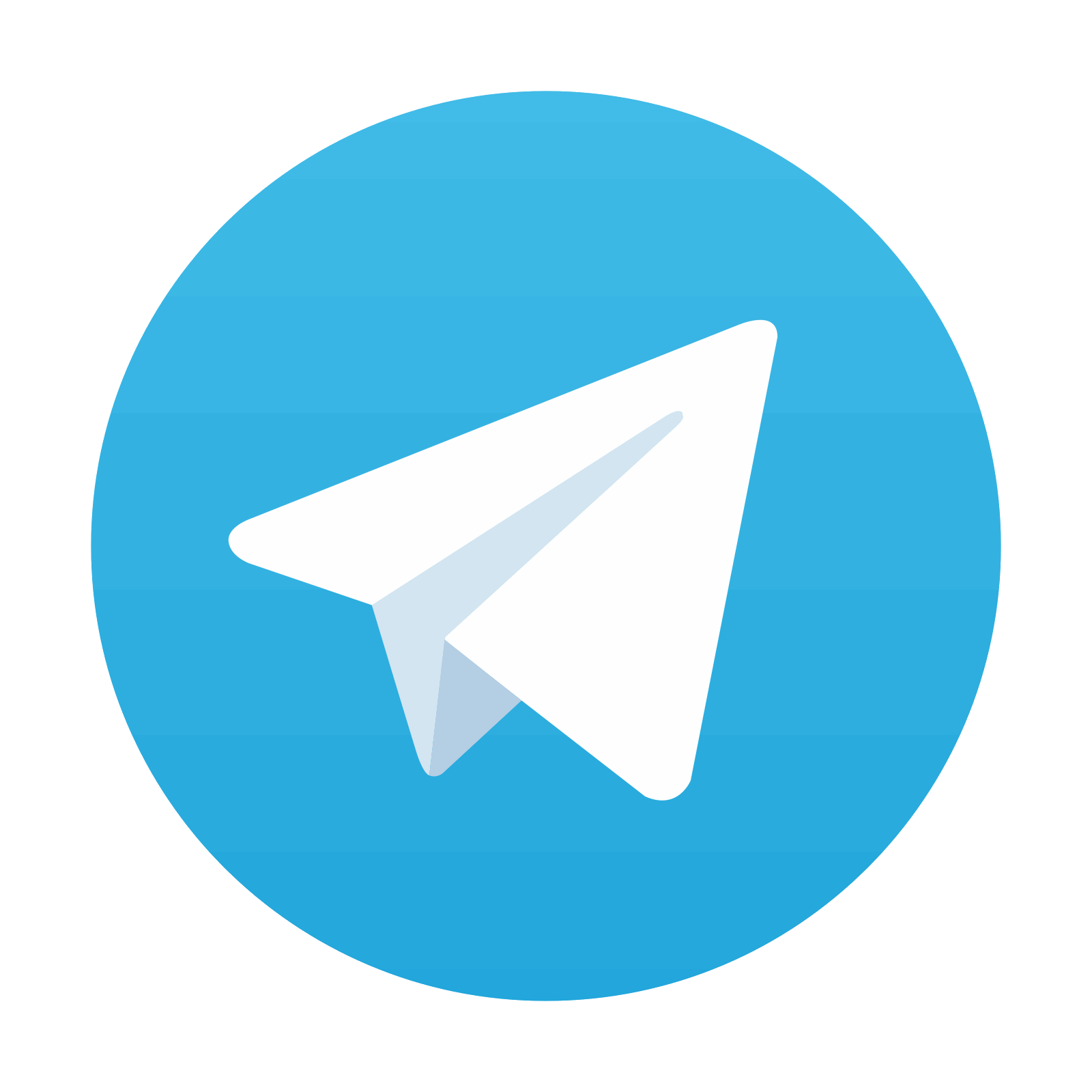
Stay updated, free articles. Join our Telegram channel

Full access? Get Clinical Tree
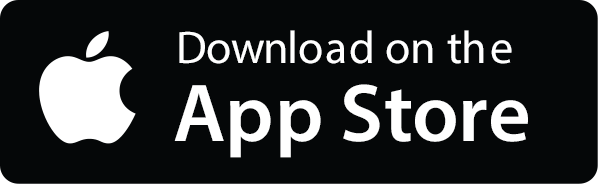
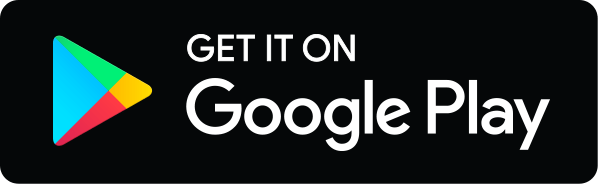