Major Concepts
Drug Resistance
Drug resistance is becoming a major problem in the treatment of infectious diseases. Several of the important types of resistant bacteria are discussed in this chapter. The first of these are drug-resistant strains of Staphylococcus aureus. Some of these bacteria (MRSA) have become resistant to penicillin, methicillin, and cephalosporins, and perhaps to aminoglycosides and fluoroquinones as well. These bacteria may be either hospital-associated or community-associated. The former often affect individuals in a weakened state or the immunosuppressed (persons who are hospitalized; persons undergoing long-term health care, dialysis, or surgery; cancer patients; and the elderly). The latter affect children in day care facilities; athletes, especially those participating in high-contact sports; prisoners; members of the military; and men who have sex with men. Vancomycin-resistant enterococci, especially Enterococcus faecium, are responsible for nosocomial infections. Drug resistance is due to factors found on a plasmid. This plasmid has been also acquired by some strains of S. aureus. Escherichia coli have developed resistance to a number of drugs, including aminopenicillins, narrow-spectrum cepalosporins, and third-generation cephalosporins and monobactams due to their acquisition of β-lactamase and extended-spectrum β-lactamase enzymes. Increasing numbers of Pseudomonas aeruginosa isolates are resistant to extended-spectrum cephalosporins, carbapenems, aminoglycosides, and fluoroquinolones. Penicillin resistance is also becoming more common among Streptococcus pneumoniae strains.
Modes of Antibiotic Action
Antibiotics use several mechanisms of action to kill bacteria or inhibit their growth. Some of these drugs interfere with synthesis of bacterial cell walls, such as the β-lactams and the glycopeptide agents. Other drugs, such as the macrolides, chloramphenicol, clindamycin, quinupristin-dalfopristin, linezolid, tetracyclines, and aminoglycosides, inhibit protein synthesis by binding to bacterial 30S or 50S ribosomal subunits. Some antibiotics block DNA or RNA synthesis. Such drugs include fluoroquinolones, sulfamethoxalone, the folic acid analogue trimethoprim, and rifampin. Another category of drugs, including polymyxins and daptomycin, acts by disrupting the bacterial plasma membrane.
Processes Leading to Drug Resistance
Drug resistance may arise by several mechanisms. These include the production of enzymes that degrade the drugs, efflux pumps that extrude drugs from the bacteria, changes in the drug’s target site, alterations in metabolic pathways, or down-regulation of the expression of the porin gene. Some of the more common resistance elements include β-lactamases, which cleave β-lactam antibiotics; the mecA gene, which induces resistance to β-lactam drugs by not binding to them; the VanX, VanH, and VanA or VanB, which alter the bacterial cell wall so that it no longer binds vancomycin; P. aeruginosa multidrug efflux pumps; and erythromycin ribosomal methylase, which inactivates erythromycin.
Prevention
Overuse or incorrect use of antibiotics is partly responsible for the rapid rise of antibiotic resistance. To avoid giving a survival advantage to resistant bacterial strains, programs need to stress compliance with prescribed drug dosage, frequency, and duration. Decreasing prophylactic use in agriculture and in personal care products may decrease the ability of resistant clones to dominate the bacterial population. The proper use of gloves and gowns, particularly by individuals in acute care settings or in contact with draining wounds, blocks transmission of hospital-associated MRSA. Hand-washing should be stressed following visits to hospitals or long-term care facilities. Covering wounds with dry bandages prevents transmission of community-associated MRSA. Personal care items should not be shared. Athletic equipment shared by multiple individuals should be cleaned with antiseptics before use. New drugs are continuing to be tested for activity against drug-resistant bacteria.
The introduction of antibiotics and other antimicrobial compounds revolutionized medical treatment of infectious diseases, leading some observers to declare victory in the lengthy war against these illnesses. The proclamation proved to be premature, for microbes demonstrated impressive abilities to escape killing or inactivation by these drugs. In populations of bacteria and other microbes, various clones exist that are resistant to the actions of different drugs. After exposure or improper exposure to these drugs, the more susceptible organisms are killed and the more resistant organisms tend to survive and may come to dominate the population under certain circumstances due to their increased fitness (greater ability to survive and reproduce). Many bacterial resistance elements are contained either singly or in groups within mobile genetic elements (discussed later in this chapter) that may enable rapid transmission of multiple drug resistance genes. As microbial populations develop resistance to existing drugs, humans have responded by producing new drugs. The microbes subsequently find means of evading these drugs, forcing humans to search for more novel agents in the continuing evolutionary waltz of life.
Increased incidence of drug resistance is currently a rapidly growing phenomenon in many types of organisms, including viruses, bacteria, protozoa, and insects. Separate chapters deal with this phenomenon in several organisms, including HIV, tuberculosis, and malaria. In bacteria, genes encoding products that allow bacteria to escape drug actions (resistance genes) often exist on plasmids, circular pieces of extrachromosomal DNA. These genes may also be found in transposons and integrons. These three genetic elements are readily passed between bacteria of the same as well as different species, allowing intra- and interspecies spread of resistance elements.
Bacteria may pass resistance genes to other organisms during the processes of conjugation, transformation, or transduction. During conjugation in gram-positive bacteria, DNA is exchanged between a mating pair of microbes. In gram-negative bacteria, plasmids are transferred to nearby bacteria in an act that often involves elongated pili that join the microbes together. Transformation involves the acquisition of DNA from other bacteria that have previously lysed and released their DNA into the external environment. During transduction, bacteriophages (viruses that infect bacteria) transfer genetic material between bacteria. These mechanisms may lead to multidrug resistance, the acquisition of resistance to three or more drugs, an increasing cause for concern, especially in health care settings.
Human actions may aid in the development of drug resistance. Such actions include noncompliance with instructions regarding the completion of a course of medication and the overuse of antimicrobial drugs in both humans and agriculture.
Antibiotics revolutionized the treatment of infectious diseases of bacterial origin. Older antimicrobial compounds included toxic compounds, such as mercury and arsenic derivatives; gramicidin, discovered by the work of René Dubos and Rollin Hotchkiss; and sulfanilamide. The first antibiotic was penicillin, produced by the fungus Penicillium notatum and discovered almost accidentally by Sir Alexander Fleming in 1928. Two Australian scientists, Howard Florey and Ernst Chain, found means of producing penicillin in the large quantities necessary for general use. Widespread use of penicillin began in the 1940s, whereupon morbidity and mortality rates from bacterial diseases fell drastically. Military forces during World War II benefited greatly as the number of deaths due to wounds infected with organisms such as Staphylococcus aureus and Streptococcus pyogenes diminished. Penicillin is itself attacked (hydrolyzed) by the enzyme β-lactamase. This enzyme is carried on a plasmid that resided within most strains of S. aureus by the end of that war. Currently, 95% of the clinical isolates of these bacteria are penicillin-resistant.
Bacteria soon began to develop resistance to other antibiotics—in 1961, methicillin-resistant strains of S. aureus were reported; during the mid-1970s, strains of P. aeruginosa developed resistance to extended-spectrum cephalosporins, carbapenems, aminoglycosides, and fluoroquinolones; in 1983, strains of E. coli became resistant to penicillin; and the 1990s saw the advent of vancomycin-resistant enterococci. Resistance genes are often contained within mobile genetic elements, allowing rapid transmission between bacteria of the same or different species. Some of these genetic elements have accumulated resistance genes to several drugs, resulting in multidrug resistance. The increased use of antibiotics in human health and in agriculture combined with noncompliance in completion of the course of medication has fueled and accelerated this natural trend in bacterial evolution.
The Diseases, Causative Agents, and Treatment Options
Methicillin- and Vancomycin-Resistant Staphylococcus aureus
Staphylococcus species are common bacteria occurring naturally in the environment and in hospital settings. These gram-positive spherical bacteria reside on the skin or in the nasal or pharyngeal passages of 25% to 40% of the general population. They may enter the body’s interior through cuts, sores, catheters, and breathing tubes. S. aureus is pathogenic, resulting in a wide spectrum of diseases ranging from minor infections of skin or soft tissues to severe sepsis with a high rate of mortality.
Penicillin was the first antibiotic to be highly effective against S. aureus infection. As noted earlier, however, penicillin resistance emerged soon after it became widely used. Other antibiotics effective against the β-lactamase-containing bacteria were introduced in the early 1960s and included methicillin and oxacillin. Within a year of their introduction, however, resistant strains of bacteria were reported in the United Kingdom and later in Europe and Australia, occurring in hospitals throughout the world by the 1980s. These strains came to be known as methicillin-resistant Staphylococcus aureus (MRSA) and were soon found to be resistant not just to methicillin but to all β-lactams, including many penicillins and cephalosporins. Many strains are also resistant to aminoglycosides and fluoroquinones.
MRSA was first reported in the United Kingdom in 1961. Between the 1970s and late 1990s, MRSA slowly began to spread and cause infections, mostly in medical centers or in patients using these facilities. By 2004, some 69% of the staphylococcal isolates in U.S. intensive care units were of the resistant phenotype. Five MRSA clones are responsible for approximately 70% of the current infections.
Infection with MRSA is especially problematic for individuals in a weakened state or immunosuppressed, such as those who are hospitalized; patients undergoing long-term health care, dialysis, or surgery; cancer patients; and the elderly. This type of MRSA is referred to as hospital-associated (HA-MRSA). Risk factors for developing HA-MRSA include exposure to a hospital or long-term health care facility, use of antibiotics within the past year, chronic disease, IV drug use, or close contact with a person having one of these risk factors. HA-MRSA infections usually involve ventilator-associated pneumonia or bacterial invasion of indwelling venous devices or wounds. Such infections are related to increased length of hospital stay and cost and higher mortality.
Recently, a growing number of MRSA infections have been found in normal, healthy people and are designated community-associated MRSA (CA-MRSA). They are particularly prevalent in Denmark and Switzerland. In one Chicago hospital, the incidence of CA-MRSA rose from 10 cases per 100,000 admissions (1988–1990) to 259 cases per 100,000 (1993–1995). CA-MRSA may be acquired by children in day care facilities and by athletes sharing equipment, including towels and razors. Athletes in high-contact sports, such as wrestling and football, are at particularly great risk. Prisoners, members of the military, and men who have sex with men are also affected. Prison outbreaks may be due to decreased hygiene, limited access to health care, infrequent clothes laundering, and limited access to soap. CA-MRSA predominantly causes infections of pulmonary tissue, bone, and skin and soft tissues and is associated with Panton-Valentine leukocidin, encoded by the pvl genes and transmitted by bacteriophages. This toxin destroys host neutrophils and causes tissue necrosis. Asymptomatic isolates have a far lower chance of carrying pvl.
HA-MRSA and CA-MRSA strains appear to differ. The two have different types of the mobile genetic island, staphylococcal cassette chromosome SCCmec, which contains the mecA gene conferring methicillin resistance. Due to the frequency of multidrug resistance, HA-MRSA is often treated with vancomycin as a drug of “last resort” despite potential adverse effects, such as nephrotoxicity and “red-man syndrome,” a histamine-like reaction. CA-MRSA isolates are often susceptible to non-β-lactam drugs, such as clindamycin, doxycycline, and trimethoprim-sulfamethoxazole. Pathology associated with CA-MRSA infections appears to be somewhat similar to those of community-associated methicillin-susceptible S. aureus (CA-MSSA). The pvl gene is found in almost all CA-MRSA isolates and 93% of CA-MSSA strains from patients with boils and in 85% of CA-MSSA strains from S. aureus pneumonia. These facts have led to the suggestion that CA-MRSA and CA-MSSA share a genetic background, as further evidenced by molecular typing studies.
The range of symptoms of S. aureus infection vary widely and include normal infections of the skin, such as red, swollen, or painful areas; abscesses; pus formation; boils; furuncles; and warmth in the affected area. Symptoms of more serious infections include rash, shortness of breath, fever, chills, chest pain, fatigue, myalgia, malaise, and headache. Invasive MRSA infections may result in bacteremia, necrotizing pneumonia, necrotizing fasciitis, cellulitis, endocarditis, septic shock syndrome (hypotension, respiratory distress syndrome or failure, and abnormal functioning of the central nervous system, liver, kidneys, or muscles), osteomyelitis, septic thrombophlebitis with pulmonary embolisms, septic arthritis, and death. Both HA-MRSA and CA-MRSA infections may be invasive, although the rate is higher with HA-MRSA. Very serious infections may result in MRSA pneumonia or blood poisoning, with high death rates. Influenza virus infection may predispose to lung colonization by CA-MRSA.
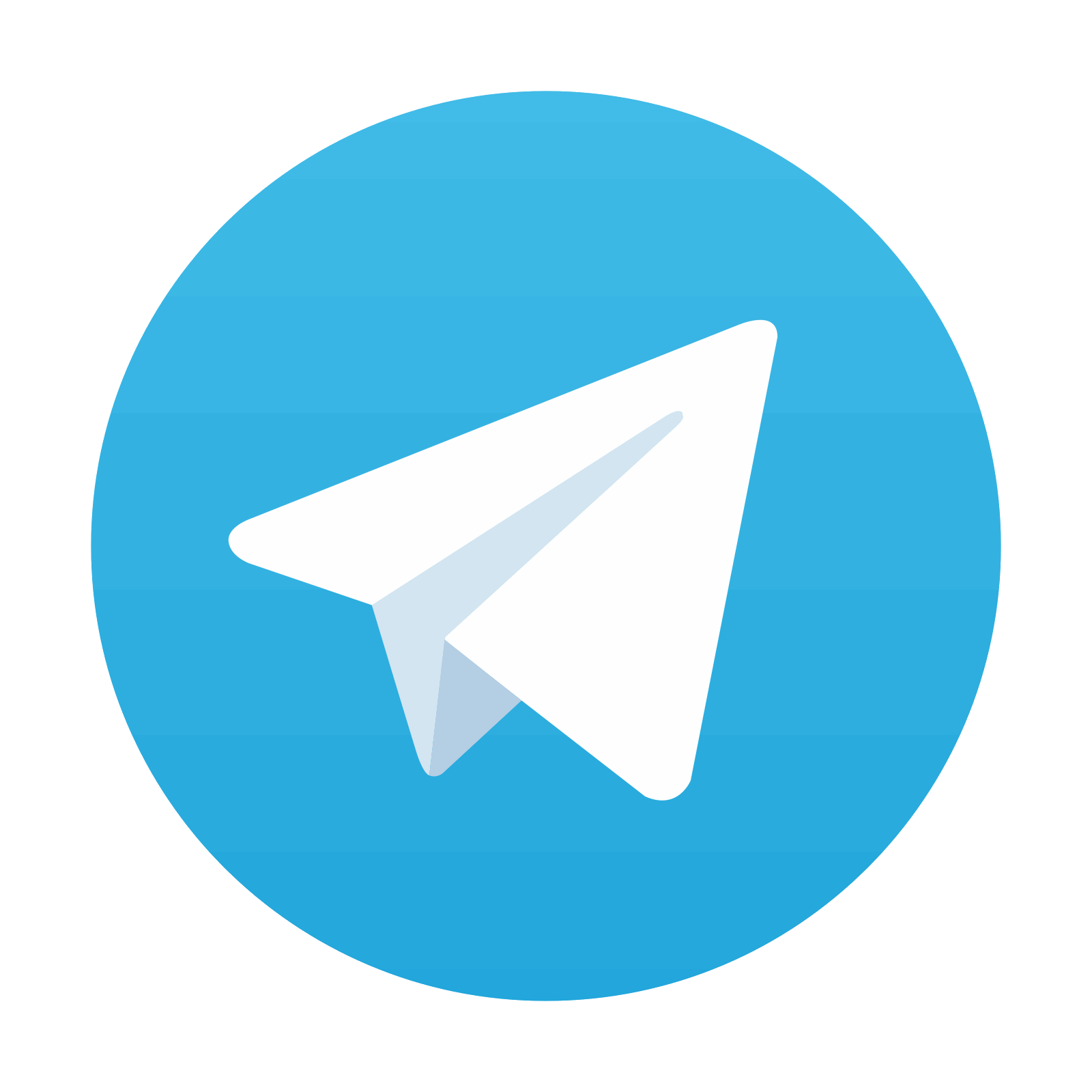
Stay updated, free articles. Join our Telegram channel

Full access? Get Clinical Tree
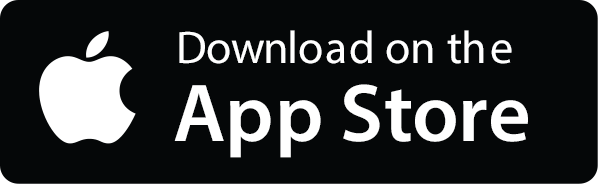
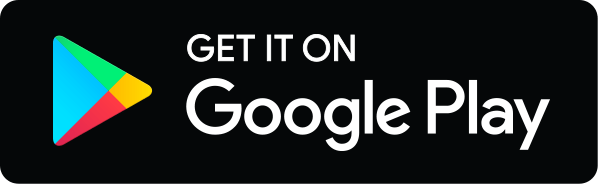