The 2 main forms of hereditary neutropenia are cyclic (CN) and severe congenital (SCN) neutropenia. CN is an autosomal dominant disorder in which neutrophil counts fluctuate with 21-day periodicity. SCN consists of static neutropenia, with promyelocytic maturation arrest in the bone marrow. Unlike CN, SCN displays frequent acquisition of somatic mutations in the gene CSF3R . CN is caused by heterozygous mutations in the gene ELANE , encoding neutrophil elastase. SCN is genetically heterogeneous but is most frequently associated with ELANE mutations. We discuss how the mutations provide clues into the pathogenesis of neutropenia and describe current hypotheses for its molecular mechanisms.
Key points
- •
Heterozygous mutations in the gene ELANE , encoding neutrophil elastase, cause cyclic neutropenia and are the most common cause of severe congenital neutropenia.
- •
Although many mutations are known, they all result in a translated polypeptide, indicating that it is not merely haploinsufficiency of the enzyme that is responsible for neutropenia.
- •
Two competing but not mutually exclusive hypotheses suggest that protein mislocalization or protein misfolding may contribute to the pathophysiology of neutropenia.
Introduction
Normal neutrophil counts in peripheral blood typically range between 1500 to 8500 cells/μL after the age of 1 year, although they vary among individuals from different parts of the world and at different ages. “Benign ethnic neutropenia” has been used to describe the normally lower neutrophil levels observed in certain populations, including African Americans and some Middle Eastern groups. Within individuals, neutrophil counts fluctuate in response to environmental and host factors and to circadian cycles.
Introduction
Normal neutrophil counts in peripheral blood typically range between 1500 to 8500 cells/μL after the age of 1 year, although they vary among individuals from different parts of the world and at different ages. “Benign ethnic neutropenia” has been used to describe the normally lower neutrophil levels observed in certain populations, including African Americans and some Middle Eastern groups. Within individuals, neutrophil counts fluctuate in response to environmental and host factors and to circadian cycles.
Heritability of neutrophil counts
Total white blood cell counts, which are largely reflective of the number of neutrophils, are moderately heritable. The Duffy Antigen Receptor for Chemokine ( DARC ) gene is at least partly responsible for the lower neutrophil counts observed in individuals of African ancestry and is associated with resistance to malaria. Several recent genome-wide association studies (GWAS) confirmed association of neutrophil counts in people of African descent with single nucleotide polymorphisms in linkage disequilibrium with DARC on chromosome 1q and identified a locus on chromosome 17q among individuals of European descent, in addition to other genetic regions. (It may be coincidental, but it is interesting that HAX1 and G6PC3 , whose mutations cause SCN, as described later, are located in proximity to the regions identified in GWAS on chromosomes 1q [nearby DARC ] and 17q, respectively. However, the area of chromosome 19p where ELANE is located has not emerged in GWAS of neutrophil counts.)
Mendelian forms of neutropenia
In contrast to the genetically influenced benign variation in neutrophil numbers arising in the general population are distinct, “Mendelian” neutropenic disorders exhibit highly penetrant single gene autosomal dominant, autosomal recessive, or X-linked inheritance.
Historically, there have been 2 primary classes of hereditary neutropenia. The first is cyclic neutropenia (sometimes referred to as cyclic hematopoiesis), in which neutrophil counts oscillate with approximately 21-day periodicity, fluctuating between nearly normal levels and a nadir lasting several days that often reaches zero. Early descriptions of cyclic neutropenia date to the first half of the twentieth century. The periodicity of the neutrophil cycling is exemplified by an unusual case in which a child with acute lymphoblastic leukemia received an allogeneic bone marrow transplant from her sister, who, along with other members of the family, was afflicted with cyclic neutropenia. The recipient was cured of leukemia but developed cyclic neutropenia, which she had not inherited at birth. Remarkably, the neutrophil counts for the 2 sisters subsequently cycled synchronously on the same days of the calendar. Monocytes also cycle in these patients, but their counts typically do so in a phase that is opposite that of neutrophils. Because monocyte counts are typically much lower than neutrophils counts, the cycling is also less apparent, probably because of greater variation in sampling errors with serial blood counts. Nearly all cases of cyclic neutropenia are autosomal dominant or represent de novo autosomal dominant mutations in ELANE , which encodes neutrophil elastase.
Description of the second major type of hereditary neutropenia is generally attributed to Kostmann, who in 1956 described a now eponymous syndrome of noncyclical “infantile agranulocytosis” observed among families residing in a remote area of northern Sweden. Individuals with this disorder demonstrate noncyclical severe neutropenia with a characteristic arrest of granulocytic differentiation at the promyelocyte stage evident on bone marrow examination. Although a substantial portion of the disorder now most often referred to as SCN is the result of allelic, heterozygous (and therefore dominantly acting) mutations in ELANE (which sometimes overlap the mutations observed in cyclic neutropenia), it is now known that SCN represents a genetically heterogeneous group of disorders. The mutated genes include those encoding the HAX1, G6PC3, WAS, GFI1, STK4, and tafazzin proteins ( Table 1 ).
Disease | Affected Gene | Manner of Inheritance | Syndromic |
---|---|---|---|
Cyclic neutropenia | ELANE | AD | No |
Severe chronic neutropenia | ELANE | AD | No |
Severe chronic neutropenia | CSF3R | AD | No |
Severe chronic neutropenia | HAX1 | AR | Yes, neurodevelopmental features |
Severe chronic neutropenia | G6PC3 | AR | Yes, congenital heart disease and other features |
Severe chronic neutropenia | GFI1 | AR | Yes, lymphocyte abnormalities |
Severe chronic neutropenia | STK4 | AR | Yes, lymphopenia, congenital heart disease |
Neutropenia | SBDS | AR | Yes, Shwachman-Diamond syndrome |
Neutropenia | RMRP | AR | Yes, cartilage-hair hypoplasia syndrome |
Neutropenia | SLC37A4 | AR | Yes, glycogen storage disease 1b |
Severe chronic neutropenia | WAS | X-linked | No (different from Wiskott Aldrich syndrome) |
Severe chronic neutropenia | TAZ | X-linked | Yes, Barth syndrome |
Although indistinguishable in their degree of neutropenia compared with SCN caused by ELANE mutations, the various autosomal recessive or X-linked forms of SCN display nonhematologic clinical features depending on the responsible gene. For example, HAX1 -associated SCN is frequently accompanied by neurodevelopmental complications, including decreased cognitive function and seizures. Individuals with G6PC3 mutations frequently have congenital heart disease and other clinical features. Patients with mutations in GFI1 display a range of alterations in lymphocyte numbers or function. In contrast, patients with either SCN or cyclic neutropenia resulting from ELANE mutations lack associated syndromic features not immediately relatable to disturbance of neutrophil numbers or function, presumably because expression of ELANE , in contrast to the case for HAX1 or G6PC3 and other genes, is mainly restricted to myeloid progenitor cells. Recently, though, neutrophil elastase was found to be expressed in smooth muscle cells derived from neointimal lesions in mice and humans with pulmonary vascular disease.
An important clinical feature of SCN that largely differentiates it from cyclic neutropenia is the risk for disease progression to myelodysplasia (MDS) and/or acute myeloid leukemia (AML). In one series, the cumulative incidence of MDS/AML was 31%, and although leukemia is anecdotally observed with cyclic neutropenia, its occurrence is uncommon. Leukemic transformation arising with hereditary bone marrow failure syndromes is well described and not unique to SCN; it is found at similar frequency, for example, in Shwachman-Diamond syndrome. However, a unique feature of leukemic progression in SCN is the strong (although not completely invariant) association with acquired mutations of the gene CSF3R , encoding the granulocyte-colony stimulating factor (G-CSF) receptor. (Although typically encountered as somatic mutations, there is at least one case report of SCN arising from germline CSF3R mutation. ) Although this is the subject of another article in this issue by Ivo Touw, there are concerns that treatment with recombinant human G-CSF (rhG-CSF) elevates risk for malignant transformation in SCN, particularly in light of the strong association with somatic mutations of the G-CSF Receptor gene and the demonstration in mice that SCN-associated G-CSFR mutations confer clonal dominance only with administration of exogenous G-CSF.
Functional deficiency of neutrophils in addition to neutropenia
An often overlooked property of cyclic neutropenia and SCN is that there are likely functional deficiencies of the neutrophils that may contribute to the risk for infection even in addition to the neutropenia observed in such individuals. Electron microscopy of myeloid progenitor cells from patients with SCN reveals ultrastructural abnormalities in the formation of neutrophil primary granules containing the hydrolytic enzymes responsible for effecting antimicrobial defense. Neutrophils from patients with SCN are deficient in antimicrobial peptides, including α-defensins. Reduced abundance of transcripts encoding other neutrophil granule components is also reported. A largely successful form of treatment of cyclic neutropenia and SCN involves administration of rhG-CSF, which increases neutrophil counts in most subjects to within the normal range. However, neutrophils recovered from the peripheral blood from treated patients with SCN display abnormalities in the maturation of granules, and the neutrophils are functionally deficient in antimicrobial activity against fungal and bacterial pathogens. This may be reflected in the types of organisms that are encountered by individuals with cyclic neutropenia and SCN, who may exhibit vulnerability to uncommon pathogens. On the other hand, the phenomenon is not necessarily a unique feature of SCN. When neutrophils are collected from CD34 + cells from peripheral blood from healthy donors then expanded ex vivo in the presence of a cytokine cocktail including G-CSF, the resulting neutrophils similarly exhibit reduced quantities of granule components, including neutrophil elastase, and limited bactericidal activity.
Osteoporosis and other bone mineralization defects are seen in SCN, although they may be a side effect of treatment with rhG-CSF. G-CSF administration reduces bone mineral density by activating osteoclasts and inhibiting osteoblasts. Bone remodeling could conceivably also be secondary to effects of dysregulated neutrophil production and/or activation in the bone marrow microenvironment.
Discovery of mutations in ELANE in cyclic neutropenia and severe congenital neutropenia
In the late 1990s, our group in Seattle performed genome-wide analysis for genetic linkage in 13 families with multiple generations of individuals affected with cyclic neutropenia, using the then-current technology of microsatellite markers, distributed with mean separation of approximately 10-cM genetic distance. In every family, we observed genetic linkage to markers mapping near the distal terminus of the short arm of chromosome 19, where obvious candidate genes included a cluster of 3 paralogous serine chymotryptic-type proteases, ELANE (then known as ELA2 ) encoding neutrophil elastase; PRTN3 encoding proteinase 3, which is the target of “c-ANCA” (cytoplasmic antineutrophil cytoplasmic antibodies) autoantibodies associated with the rheumatologic disorder Wegener’s granulomatosis; and NAZC encoding azurocidin, in which the catalytic serine has disappeared, resulting in a protein that no longer displays proteolytic activity. It is worth noting that these proteins display antimicrobial activity distinct from their enzymatic activity. After sequencing this cluster of genes, we detected 7 different heterozygous mutations in ELANE that segregated with cyclic neutropenia in each of the 13 families we studied.
We next evaluated ELANE as a candidate gene in 27 SCN unrelated cases. In 21 of the 27 cases, we detected 1 of 15 different heterozygous ELANE mutations. Many of the cases occurred sporadically, but when DNA samples from other affected individuals in the family were available, in nearly all cases when there was evidence of parent-to-child transmission, consistent with autosomal dominant forms of inheritance, the same ELANE mutation was detected in all members of a pedigree. For the most part, ELANE mutations occurring in patients with SCN are distinct from those found in patients with cyclic neutropenia. Nevertheless, at times the same particular mutation is found in individuals with decidedly clinically distinct forms of neutropenia (ie, cyclic versus chronic). There are several possible explanations. First, the clinical evaluation of cyclic neutropenia can be challenging, because observing cycles requires frequent serial blood counts. Oscillation patterns may exhibit irregularity, may disappear entirely at times, and are influenced by therapy, particularly rhG-CSF treatment, which tends to shorten the periodicity of cycling and increase the amplitude of the cycles, without abrogating cycling completely. Some individuals may cycle but exhibit low peak amplitudes resulting in a classification of SCN. Another possibility is that the clinical assignment was made in light of knowledge of the mutation, so genotype-phenotype correlations could conceivably be biased as a result of knowledge of the particular mutation. Finally, there is some recent evidence to suggest that there could be modifier genes and that the modifiers might themselves include variants of ELANE , HAX1 , and G6PC3. In particular, 2 individuals with SCN who seemed to be heterozygous for a pathogenic mutation in ELANE were also heterozygous for either HAX1 or G6PC3 mutations. (Ordinarily, HAX1 and G6PC3 act recessively, and homozygous mutation is thought to be required to produce the SCN phenotype.) Another patient was a compound heterozygote for 2 different pathogenic HAX1 mutations but was also heterozygous for a G6PC3 mutation. Finally, one other SCN patient was heterozygous for both G6PC3 and HAX1 mutations. It is worth emphasizing that although recessive forms of SCN are rare, consideration of Hardy-Weinberg equilibrium indicates that carriers are markedly more common, raising the possibility that heterozygosity for HAX1 or G6PC3 is an incidental finding in these cases. In another study, 2 patients with SCN had novel variants in GFI1 , which can also be a cause of SCN (see later). In yet another study, an SCN patient was found to have 2 ELANE amino acid missense substitution mutations (V53M and V69M), both occurring in cis on the maternally inherited allele. The mother, however, exhibited only one of the variants (V69M, which has not apparently been seen before in either cases or controls) and was asymptomatic with normal neutrophil counts. (There was also a third variant within an intron whose parental inheritance, if any, was uncertain.) Thus, V53M must have arisen de novo. It is interesting that V53M had only previously been found in patients with cyclic neutropenia. The authors therefore concluded that the combination of V69M, which alone had no effect on granulopoiesis (as found in the mother), with the V53M mutation, ordinarily producing cyclic neutropenia, yielded SCN with a particularly severe clinical course, including invasive pulmonary mycosis and development of MDS with progression to AML at age 8 years.
Another suggestion of modifier genes comes from an unusual case in which a healthy sperm donor fathered (at least) 8 neutropenic children with 6 different women. Seven of the 8 children had SCN, whereas one of the children demonstrated evidence of neutrophil cycling. The authors interpreted this observation as indicating phenotype determination by modifying genes, given that all the affected children inherited the same paternal allele. (Sanger dideoxy DNA sequence analysis of the father’s sperm was consistent with mosaic representation of the ELANE mutation. See further discussion of genetic mosaicism later.)
As noted, de novo mutation of ELANE is fairly common, more so among SCN than among cyclic neutropenia cases. In fact, the occurrence of de novo ELANE mutation complicated the search for autosomal recessive forms of SCN. There are individuals with SCN residing in Sweden who are descendants of the cases first reported by Kostmann more than 50 years ago. The gene responsible for autosomal recessive SCN in this population, HAX1 , was mapped by linkage analysis and positionally cloned by making use of this Swedish family as well as other families from ethnically isolated populations in the Middle East. Four members of Kostmann’s original family with SCN were studied. Although 3 were found to be homozygous for the same HAX1 mutation, one individual whose disease was thought to be clinically indistinguishable from that of his affected siblings (and therefore was attributed to the same ancestral gene) evidently lacked the segregating HAX1 mutation and was reported to have a new ELANE mutation not present in his parents. This is not a genetically surprising phenomenon, given that disorders with reduced reproductive fitness—noting that severe infectious complications, as well as the risk for leukemic progression, severely shorten life—must necessarily arise from new mutations, because affected individuals are less likely to survive to reproductive age. Nevertheless, it is possible that ELANE may represent a hotspot or new germline mutation. We have reported several cases in which there have been 2 de novo substitutions occurring in cis on the same allele of ELANE , representing a phenomenon that has only been extremely rarely reported for other disorders. In the 2 cases we described in which the origin of the mutation could be determined, the mutations had arisen on paternally inherited alleles. This could possibly reflect vulnerability of this locus to mutational phenomenon or, by analogy to study of de novo FGFR3 mutations causing achondroplasia and other new mutations showing paternal bias, it could reflect selective advantage during male germ cell formation. Arguing against that possibility, though, is the previously discussed case of another patient with 2 cis ELANE mutations ; one was de novo and one was inherited, but both came from the mother.
The phenomenon of de novo mutation leads to discussion of the significance of germline mosaic ELANE mutations. It is now well recognized that in a variety of autosomal dominant disorders, unaffected individuals lacking evidence of the mutation in DNA obtained from peripheral blood or epithelial or other cells obtained via buccal swab or skin biopsy may be a parent to multiple children exhibiting the disorder and sharing a common mutation (the aforementioned sperm donor being one such example). In such cases, the “transmitting” parent is understood to harbor the mutation in only a fraction of the cells required to exhibit symptoms in the relevant tissue (eg, bone marrow for neutropenic disorders) yet sufficiently populates the germline so that its inheritance follows Mendelian expectations.
In fact, documentation of such “germline mosaicism” has allowed for drawing some interesting conclusions about how ELANE mutations cause neutropenia. In the first instance in which germline mosaicism was reported, an unaffected father was found in peripheral blood from his DNA to demonstrate the same ELANE mutation as present in his affected child. Closer inspection revealed that the mutation was detectable in about half of his lymphocytes but less than 10% of neutrophils. Individual hematopoietic colonies obtained from peripheral blood were either heterozygous for the mutation or homozygous wild-type. One suggested explanation for this and other demonstrated or likely cases of ELANE mosaicism was that it must mean that ELANE mutations are, by themselves, not causative of neutropenia. We think, however, that a more probable—and provocative—conclusion is just the opposite: that neutrophil progenitors bearing the mutation do not eventuate in mature neutrophils yet are unable to block differentiation of wild-type cells. These observations implicate cell autonomous phenomenon instead of involvement of paracrine effects, at least with respect to how mutations in ELANE lead to SCN, if not also to cyclic neutropenia.
ELANE gene mutations
It is, first, worth noting that the pathogenic role of ELANE mutations in hereditary forms of neutropenia has been repeatedly called into question. We find this baffling. Families with cyclic neutropenia or SCN exhibit clear-cut multigenerational patterns of inheritance consistent with single gene autosomal dominant transmission. The initial linkage analysis identified just a single locus in 13 of 13 pedigrees with cyclic neutropenia, and ELANE mutations, some different and some recurrent yet appearing on different ancestral haplotypes (meaning that they arose independently within the population), were detected in all 13 families. Since then, hundreds of patients with either cyclic neutropenia or SCN who have ELANE mutations have been identified —to list but just a few of the many cases reported in the literature. Multiple examples of de novo mutation of ELANE , which is generally accepted as evidence of causality—particularly when examining only a single gene, are now known. Cases of germline mosaicism are also consistent with a causative role. Commercial genetic testing has become routine. Finally, as whole genome or whole exome sequencing becomes increasingly common and databases of common variants are compiled by sequencing thousands of controls, other causative genes can be excluded and the absence of the observed ELANE mutations from control populations can be confirmed. We assert that there are few other genes for which the evidence of causality is so strong.
The nature of the mutations observed in ELANE also provides clues about their pathogenicity
ELANE contains 5 exons. All known ELANE chain-terminating mutations (nonsense or frameshift mutations leading to altered reading frames with incorrect translation of an out-of-frame stop codon) occur in the fifth and final exon. (Chain-terminating mutations within ELANE are, for the most part, confined to patients with SCN and are not observed with cyclic neutropenia.) Although chain-terminating mutations occurring within internal exons generally produce transcripts that are selectively removed before translation through the process of nonsense-mediated decay and therefore do not produce an abbreviated polypeptide, mutations occurring in the final exon are typically exempted from such cellular quality control mechanisms and do actually lead to peptides truncated at the carboxyl terminus. This important observation leads to 2 significant conclusions. First, haploinsufficiency of neutrophil elastase is unlikely to be causative of the disorder; otherwise, chain-terminating mutations or whole gene deletions, which have never been observed, should be expected in the first 4 exons. Second, the carboxyl-terminal portion of the polypeptide likely contributes functionally to prevent neutropenia (as discussed later, potentially a binding site for adapter protein 3 (AP3), involved in intracellular trafficking of neutrophil elastase).
Initially, shortly after the discovery of ELANE mutations in cyclic neutropenia and SCN, there seemed to be possible clustering patterns related to either the lineal or tertiary distribution of mutations with respect to gene and protein structure, respectively, that predicted their occurrence within either subtype of inherited neutropenia. Potential correlation between mutation location and phenotype has substantially weakened as the number of unique mutations has grown as genetic analysis of ELANE has become increasingly common in the evaluation of early childhood neutropenia. Mutations, typically single base missense substitutions or small in-frame indels or splice site mutations producing in-frame deletion or insertion of a few amino acid residues, are now found distributed throughout the length of the gene, rendering any clear pattern, if one actually exists, less obvious ( Fig. 1 ). It has become difficult to maintain an accurate database of the various mutations now described because of the plurality of research and commercial laboratories performing testing. Still, some general observations regarding the nature of the mutations hold. The splice donor site at intron 4 is perhaps the most frequent site at which mutations occur. Base substitutions at the first, third, or fifth position of the intron force use of a cryptic splice donor site 30 nucleotides upstream of the canonical site, leading to deletion of 10 amino acid residues from within a region of the protein containing the catalytic site (Δ161-F170). These mutations are nearly exclusively found in individuals with cyclic neutropenia and are the overwhelmingly most common mutations, with respect to their common effect on protein coding, in cases of cyclic neutropenia. In addition to the observation of chain-terminating mutations in the final exon being nearly exclusively found among patients with SCN, a fairly commonly seen mutation, G815R, seems to confer a particularly severe clinical course. Individuals with this mutation tend to have lower neutrophil counts, are refractory to rhG-CSF treatment, and seem to progress to MDS and/or AML at high frequency.
Biochemistry of neutrophil elastase
ELANE encodes neutrophil elastase, a monomeric approximately 30-kDa glycoprotein. As noted, neutrophil elastase is closely related to azurocidin and proteinase 3, which are encoded by adjacent genes in the same cluster on chromosome 19p. All 3 enzymes are also closely related to cathepsin G, whose gene is found on a different chromosome (14q). All 4 proteins are major component of neutrophil azurophilic granules.
Neutrophil elastase takes its name from the fact that the connective tissue protein elastin was among the first of its known substrates ; however, neutrophil elastase claims a large number of different proteins among its substrates. Its ability to accept virulence factors from a variety of Gram-negative bacterial species as substrates, including the outer membrane protein of E coli , contributes to its antimicrobial activity.
Neutrophil elastase is also involved in the processing of cytokines, chemokines, and growth factors, including tumor necrosis factor-α and stromal cell–derived factor-1α (also referred to as CXCL12), which serves as a chemoattractant for lymphocytes, monocytes, and dendritic cells. It is interesting that inactivation of patients with stromal cell–derived factor-1α in mice leads to deficient myelopoiesis, and inactivation of its receptor, CXCR4, is the cause of the WHIM syndrome (warts, hypogammaglobulinemia, infections, and myelokathexis). In the WHIM syndrome, peripheral neutropenia is a consequence of myelokathexis, the retention of neutrophils within the bone marrow. Of unknown relevance to SCN, neutrophil elastase also cleaves G-CSF and its receptor.
Neutrophil elastase is synthesized as a 267-amino-acid residue inactive zymogen. It is posttranslationally processed to first remove a 27-residue “pre” signal sequence required for intracellular membrane insertion and ultimately extracellular secretion. Then a “pro” peptide of just 2 amino acids is removed from the amino terminus by dipeptidyl peptidase I (also known as cathepsin C). Neutrophil elastase also contains a carboxyl-terminal propeptide of 20 residues that is cleaved by an as-yet-unidentified protease. Fully processed mature neutrophil elastase contains 218 amino acid residues. Unlike other serine proteases, such as trypsin, in which proteolytic conversion from zymogen to active enzyme yields 2 intertwined polypeptides, the processed portions of neutrophil elastase are not found to associate with the resulting mature single protein chain. Regarding posttranslational modifications, neutrophil elastase contains 2 sites of N -glycosylation.
Deficiency of DPPI, responsible for cleaving the 2-residue propeptide of neutrophil elastase, causes an autosomal recessive human disorder, Papillon-Lefevre syndrome, consisting of skin and periodontal disease. Individuals with Papillon-Lefevre syndrome therefore fail to appropriately excise the carboxyl-terminal propeptide domain from neutrophil elastase, proteinase 3, cathepsin G, and azurocidin but yet are not described as neutropenic.
Neutrophil elastase’s tertiary structure is composed of 2 β-barrels, containing 6 antiparallel β-sheets connected through a linker segment, and a carboxyl-terminal α-helical domain. As with other serine proteases, there is a triad of catalytic residues (S195, D102, and H57) forming a “charge relay” system. Initially, the histidine is deprotonated by the carboxylate side chain of aspartate. The histidine, in turn, deprotonates serine. The proton originally bound to the serine hydroxyl group is transferred to the amino group in the substrate’s peptide bond, allowing the histidine to accept a proton from water, which then attacks the acyl enzyme intermediate, leading to reformation of the enzyme.
Neutrophil elastase and related chymotryptic serine proteases have several endogenous inhibitors. Most prominent perhaps are the serpins (serine proteinase inhibitors), of which the most well-characterized is α1-protease inhibitor (also known as α1–anti-trypsin, but which may play a more significant role in inhibiting neutrophil elastase). Human genetic deficiency of α1-protease inhibitor leads to early onset of emphysematous lung disease, resulting from unopposed neutrophil elastase–mediated destruction of pulmonary elastic fibers and other connective tissues. Certain genetic variants of α1-protease inhibitor can also cause cirrhosis because of their ability to misfold and form protein aggregates that prove toxic to hepatocytes. Among other serpins, monocyte neutrophil elastase (now referred to as SerpinB1) seems to account for a substantial portion of physiologic inhibition of neutrophil elastase. Serpins achieve inhibition of neutrophil elastase and other serine proteases through an irreversible suicide substrate mechanism in which the targeted enzyme cleaves the serpin and generates a covalently bound inhibitory complex. “Canonical inhibitors,” including elafin and secretory leukocyte protease inhibitor, form another group of endogenous inhibitors of neutrophil elastase and related serine proteases. Although encoded by different gene families, they share a canonical formation of their inhibitory protease binding loop.
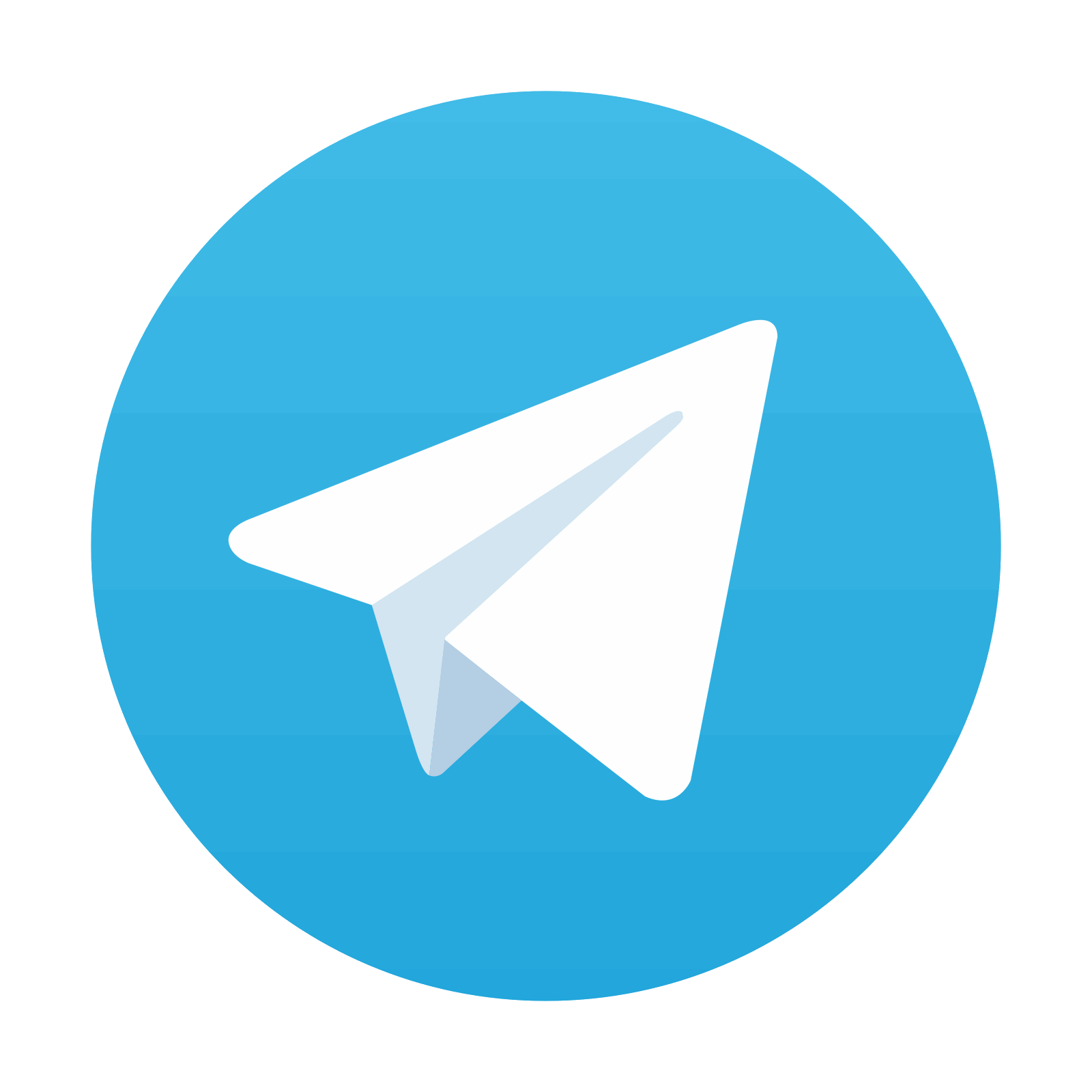
Stay updated, free articles. Join our Telegram channel

Full access? Get Clinical Tree
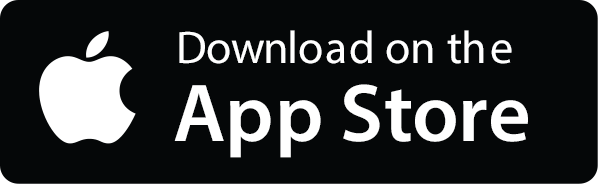
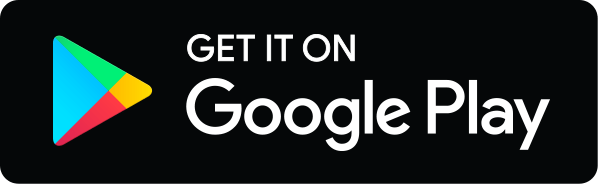