Effect of Exercise on Dyslipidemia
Mary P. McGowan
The profound benefits of regular physical activity (PA) cannot be disputed. Individuals who engage in the regular expenditure of energy whether as a formal exercise program, or as part of their occupation, enjoy lower rates of both cardiovascular disease (CVD) and all-cause mortality (1). Initiation of exercise following a cardiac event likewise reduces subsequent coronary and all-cause mortality (2).
Regular PA has also been reported to reduce the risk of breast and colon cancers, adult-onset diabetes mellitus, stroke, peripheral vascular disease, and osteoporosis. Evidence also suggests that habitual exercise improves mood and may prevent the development of Alzheimer disease (3).
While it is generally agreed that in part the ability of endurance training to decrease cardiac disease stems from its impact on lipids and lipoproteins, there is substantial interindividual variation in lipoprotein response to exercise. This suggests that genetic factors play a role. This chapter will review findings supporting the belief that genetic variability not only influences baseline lipids but also predicts an individual’s response to exercise.
Not surprisingly, like most things in medicine, the lipoprotein response to PA is complex and is influenced by many nongenetic variables as well. These include frequency, intensity, duration, and type of exercise (aerobic vs. resistance). Likewise, ethnicity and gender influence lipid response and will also be explored.
Finally, because 70% of adult Americans perform no regular leisure-time PA, office-based counseling techniques will be addressed (4).
THEORETICAL CONSIDERATIONS
Genetic Factors
Individuals with dyslipidemia, that is, depressed plasma levels of high-density lipoprotein (HDL) cholesterol (HDL-C), elevated levels of triglyceride (TG)-rich lipoproteins, and elevated low-density lipoprotein (LDL) cholesterol (LDL-C), are at substantially increased risk for the development of coronary heart disease (CHD) (5,6). Regular exercise training has been found to result in a small but significant increase in HDL-C (5,6,7). Significant reductions in TG have been reported less frequently (5,6) and aerobic activity has only infrequently been linked to improved LDL levels (7). One study, however, found that even moderate-intensity aerobic exercise was capable of positively influencing the size and chemical composition of plasma LDL-C, potentially rendering it less atherogenic (8).
As noted above, the significant heterogeneity in lipid response to exercise suggests that underlying genetic factors are at play. The Health, Risk Factors, Exercise Training and Genetics (HERITAGE) Family Study included 527 individuals from 99 white families and 326 individuals from 113 black families. This study assessed major gene effects for baseline HDL-C, LDL-C, TG, and their training response. The training program consisted of three vigorous exercise sessions per week for a total of 20 weeks (9).
In whites, consistent with at least two other large studies (10,11), the HERITAGE Family Study found evidence that baseline LDL was under the influence of major recessive genes accounting for 27% of the phenotypic variance. Approximately 12% of the population was found to be homozygous for a genotype leading to an elevated LDL-C.
No major gene effect was found for LDL-C training response. In contrast to the LDL-C findings, no major gene effect for baseline HDL-C was found in white participants (9). There was, however, evidence of a major gene effect for an HDL-C training response. This gene appeared to have a recessive mode of inheritance and accounted for 19% of the phenotypic variance. Only a very small percentage (3%) of whites were noted to be homozygous for a genotype, which resulted in a significantly greater HDL-C response to endurance training (9).
The findings in black families for LDL and HDL both at baseline and in response to training differed from those in whites. A major dominant gene effect for baseline HDL was established accounting for 45% of the phenotypic variance. But no evidence that either baseline LDL-C or HDL-C training response was under the influence of major genes was found (9).
With regard to TG, both races were found to have evidence of major effects for both baseline and training response, but the mode of inheritance of these major effects could not conclusively be proven to be Mendelian (1).
Apolipoproteins and Exercise Response
Apolipoprotein E Isoforms
Apolipoproteins make up the protein constituent of lipoproteins. Apolipoprotein E (apoE) is associated with chylomicrons, very low density lipoproteins (VLDL), intermediate-density lipoproteins (IDL), and HDL. Following the hydrolysis of TG on the apolipoprotein B (apoB)-containing lipoproteins, apoE facilitates the binding and uptake of chylomicron remnants and VLDL remnants and IDL by receptors on the surface of the hepatocyte (see also Chapters 1, 7, and 8). Due to genetic mutations, apoE has three principal isoforms (E2, E3, E4)
resulting in six genotypes (E2/E2, E2/E3, E2/E4, E3/E3, E3/E4, E4/E4). Of these, three genotypes (E2/E3, E3/E3, E3/E4) account for 10.7%, 62.4%, and 22.3% (or over 95%) of the population, respectively (12).
resulting in six genotypes (E2/E2, E2/E3, E2/E4, E3/E3, E3/E4, E4/E4). Of these, three genotypes (E2/E3, E3/E3, E3/E4) account for 10.7%, 62.4%, and 22.3% (or over 95%) of the population, respectively (12).
The genotypic variations in apoE result in different affinities of apoE for the receptors on the hepatocyte. For example, TG are higher in apoE2 homozygotes and heterozygotes. In large part, this is due to the reduced affinity of apoE2 for the chylomicron remnant receptor (also called low-density lipoprotein receptor like protein [LRP]) and for the LDL receptor (LDLR) (that removes VLDL remnants and IDL). Another consequence of this diminished affinity of apoE2 is a reduced delivery of cholesterol to the liver, resulting in an upregulation of hepatic LDLR and an enhancement of LDL clearance from the plasma and lower LDL levels in apoE2 homozygotes and heterozygotes (12,13).
ApoE3/E4 subjects tend to have higher LDL levels than apoE3/E3 homozygotes. The mechanism has not been completely elucidated. ApoE4 has a greater affinity for the LDLR and may therefore promote a greater hepatic uptake of VLDL and IDL remnants. These lipoproteins are in turn delivered to the liver resulting in downregulation of LDLR activity and increased plasma LDL levels (13) (see also Chapter 8). It has also been proposed that the association of apoE4 with HDL is less than that of apoE3 and apoE2 and that the apoE4 isoform is more rapidly transferred from HDL to the TG-rich lipoproteins, resulting in their enhanced uptake by the liver (13) (see also Chapter 7).
Given the importance of apoE in metabolism of TG, the Exercise and Genetics Collaborative Research Group sort to evaluate the influence of baseline apoE genotype on lipoprotein response to exercise in 120 previously sedentary men and women. In order to be included in this 6-month supervised exercise program, subjects were required to have one of the three most common apoE genotypes. Participants exercised four times a week for 60 minutes achieving 60% to 85% of Vo2max (13).
As would be predicted, although not statistically significant (perhaps due to small numbers), pretraining TG were higher and LDL-C levels lower in the apoE2/E3, compared with either the apoE3/E3 or apoE3/E4 subjects (13).
Training resulted in an 11% reduction in TG for the entire group. The reduction was not uniform, falling by 7%, 12%, and 14% for the apoE2/E3, apoE3/E4, and apoE3/E3, respectively. And while LDL did not change appreciably for the group as a whole, it was noted to decrease marginally in the apoE2/E3 and apoE3/E3 but increased 4% among the apoE3/E4 subjects. HDL rose 2% for the entire cohort, due primarily to a 6% increase in the E3/E3 group. While none of these individual lipoprotein changes achieved statistical significance, it is notable that the total cholesterol (TC)/HDL and LDL/HDL ratios decreased in the E2/E3 and apoE3/E3 groups but increased in the apoE3/E4 subjects. This change was statistically significant suggesting that exercise training may reduce cardiac risk more in some groups than in others (13).
Apolipoprotein A-I
Apolipoprotein A-I (apoA-I) is synthesized in the liver and gut epithelium and is found on both HDL and chylomicrons. ApoA-I accounts for approximately 70% of the protein in HDL (6). Previous work has determined that the —75 G/A single-nucleotide polymorphism (SNP) in the apoA-I promoter influences HDL concentrations as well as response to dietary changes (14). The Exercise and Genetics Collaborative Research Group evaluated the effect of this apoA-I SNP on HDL subfractions in 75 of the 120 subjects participating in the previously mentioned 6-month exercise study. Of the 75 subjects, 53 were G homozygotes (G/G), while 22 were A carriers (either A/A or A/G). Exercise training increased levels of small HDL-C and decreased levels of large HDL-C in A carriers. Just the opposite finding occurred in the G homozygotes. Since large HDL particles appear to be antiatherogenic and increased small HDL particles may reflect the presence of overproduction of VLDL and small, dense LDL particles, an atherogenic lipoprotein phenotype (see also Chapter 8), this finding suggests that G homozygotes may benefit more from training than A carriers (14).
Cholesteryl Ester Transfer Protein
Genetic variation of key enzymes and transfer proteins involved in lipoprotein metabolism has also been found to influence lipid response to exercise training. Cholesteryl ester transfer protein (CETP) catalyzes the heteroexchange of cholesterol esters from HDL to apoB-containing particles in exchange for TG. As a result, LDL-C levels are directly and HDL-C levels are inversely correlated with CETP activity. Several different polymorphisms of CETP have been identified. These polymorphisms influence plasma CETP concentration, HDL-C level, and risk of coronary atherosclerosis (30). Two common polymorphisms are the B1/B1 and B2/B2 (6).
One small study involving 16 middle-older aged B1/B1 and 14 B1/B2 men and women examined the impact of genotype on HDL response to 6 months of endurance training. HDL and its subfractions were measured by nuclear magnetic resonance (NMR) spectroscopy. The initial lipoprofile did not differ between the groups. At the conclusion of the study, B1/B1 subjects appeared to have an increase in HDL2nmr-C levels and a reduction in HDL3nmr-C levels. On the contrary, the B1/B2 subjects experienced a reduction in HDL2nmr-C levels. Although limited by its small size, this study provides initial evidence suggesting that changes in HDL subfractions in response to exercise training may be dependent on CETP genotype (15).
While by no means exhaustive, these examples provide evidence supporting the concept that individual variability in lipoprotein response to exercise training is in part due to genetic variation.
Duration, Frequency, and Intensity of Exercise
Although genetic makeup cannot be altered, there are many other factors that determine an individual’s response to exercise. Three of these factors include duration, frequency, and intensity of exercise.
Duration is the length of time (expressed in minutes) an exercise is performed. Frequency is the number of times an activity is performed and is typically expressed as number of sessions per week (3).
Intensity, which can be expressed in either absolute or relative terms, refers to exactly how much work is being performed or how much effort is expended to perform a particular activity (3).
Intensity of Exercise Energy Expended
The absolute intensity of an exercise is expressed in terms of energy expended or metabolic equivalents (METS). An MET is the ratio of the rate of energy expended during a specific activity to the rate of energy expended at rest. The rate of energy expended at rest is deemed 1 MET. A 5-MET activity would then by definition be an activity that expends five times the energy used by a body at rest. If a person performs a 5-MET activity for a period of 60 minutes, he or she will have performed 5 × 60 or 300 MET minutes (or 5 MET hours) of PA (3).
According to the 2008 Physical Activity Guidelines for Americans, light-intensity activities are those expending 1.1 to 2.9 METs, moderate-intensity activities are defined as those expending 3.0 to 5.9 METs, and vigorous-intensity activities involve the expenditure of 6.0 or more METs. An example of a moderate-intensity activity is walking at 3.0 miles per hour (MPH) (a 3.3-MET activity). Running at 6.0 MPH is a 10-MET activity and as such is considered to be a vigorous-intensity activity (3).
In contrast, describing activities in terms of their relative intensity takes into account an individual’s exercise capacity. In this situation, intensity is expressed in terms of a percent of a person’s maximal heart rate or maximum aerobic capacity (Vo2max). For simplicity sake, relative intensity is often defined using a scale of perceived exertion ranging from 0 to 10, with 0 being rest and 10 being maximum effort. In this setting, moderate-intensity activities are generally considered to be 5 to 6 on the perceived exertion scale and 7 or higher considered to be vigorous activities. The Borg Scale of Rated Perceived Exertion (RPE) (Table 22.1) is still another commonly used scale. Activities are rated from 7 to 19 with 7 being very, very light and 19 being very, very hard (16).
Current guidelines recommend that adult Americans strive for at least 500 to 1,000 MET minutes per week. This translates to 2.5 hours of moderate-intensity activities or 1 hour and 15 minutes of vigorous-intensity activities. This level of physical has been conclusively shown to provide health benefits. More substantial benefits can be gained with greater amounts of exercise (3). As noted earlier, one of the health benefits resulting from regular aerobic activity is improved lipid levels.
TABLE 22.1 BORG SCALE OF RATED PERCEIVED EXERTION (RPE) | ||||||||||||||||||||||||
---|---|---|---|---|---|---|---|---|---|---|---|---|---|---|---|---|---|---|---|---|---|---|---|---|
|
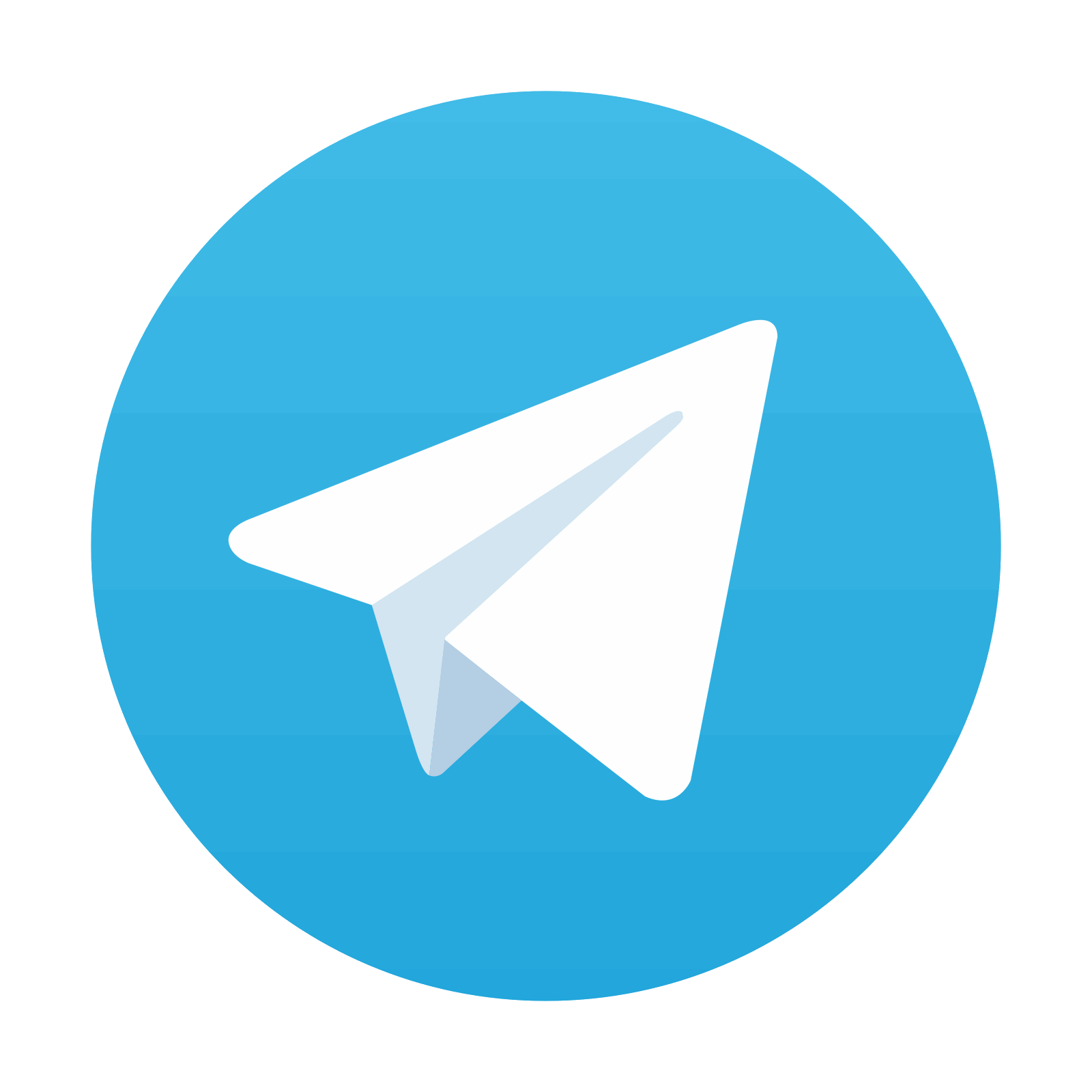
Stay updated, free articles. Join our Telegram channel

Full access? Get Clinical Tree
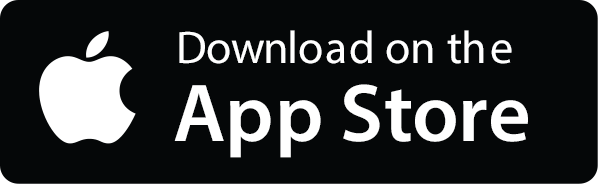
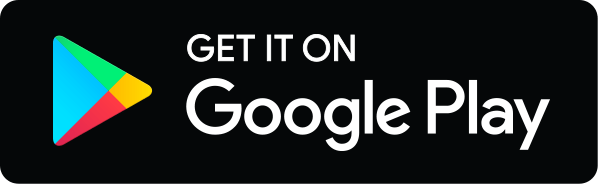
