Thrombocytopenia is the commonest haematological abnormality in neonates, particularly among sick and/or very low birthweight neonates (reviewed in references 1–3). Overall, up to 5% of neonates will have platelet counts of <150 × 109/l in the first week of life, the incidence varying depending on the population studied.1–3 Many of these studies have analysed cord blood platelet counts, which may have over‐estimated the true incidence of thrombocytopenia. The study by de Moerloose et al.4 was also performed on cord blood samples and included samples from more than 8000 consecutive deliveries but, as all cases of thrombocytopenia were subsequently confirmed by heel‐prick samples, their calculated incidence of neonatal thrombocytopenia of 0.9% probably provides the most accurate estimate. When neonates develop thrombocytopenia it is usually mild. Severe thrombocytopenia (platelet count <50 × 109/l) is reported to occur in 0.1–0.5% of neonates, with the variation reflecting differences in the proportion of very low birthweight neonates included in the study.5–7 For example, the incidence of thrombocytopenia is significantly higher in neonates who are admitted to neonatal intensive care units (NICUs), where thrombocytopenia occurs in approximately 40% of all babies.6,8 Several studies have shown that the severity of thrombocytopenia worsens with prematurity and with the severity of the clinical condition; the incidence of thrombocytopenia in sick neonates requiring intensive care can be as high as 70–80%.6,9,10 Apparent thrombocytopenia should normally be verified by repeating the full blood count and by blood film examination, which may show platelet clumps or fibrin strands, and in addition may suggest a cause of the thrombocytopenia, such as sepsis or necrotising enterocolitis (NEC) (see Figs 1.16, 3.6 and 3.15b). The most common cause of spurious thrombocytopenia in neonates is a blood clot in the sample which may not have been detected at the time of analysis. This is a particular problem in neonates who are polycythaemic. Pseudothrombocytopenia, which is usually caused by in vitro ethylene diaminetetra‐acetic acid (EDTA)‐dependent platelet clumping, is not uncommon in neonatal samples. In some cases, this has been shown to be due to transplacental passage of an EDTA‐dependent antibody that leads to in vitro platelet aggregation, in which case it is self‐limiting.11,12 However, severe pseudothrombocytopenia can also occur independently of EDTA and can persist for several months after birth.13 The vast majority of episodes of thrombocytopenia are self‐limiting, occur secondary to pregnancy‐related complications in the mother or to postnatally acquired infection in the baby and are accompanied by no or minor bleeding. The most common sites of minor haemorrhage in thrombocytopenic neonates are bleeding from the renal tract, from the skin or from endotracheal or nasogastric tubes.14 Nevertheless, the risk of significant or serious haemorrhage in thrombocytopenic neonates is high,14 particularly in extremely low birthweight neonates. The most common types of major haemorrhage in neonates are intracranial haemorrhage (ICH), which occurs in about 5% of all thrombocytopenic neonates, followed by gastrointestinal haemorrhage (1–5%), pulmonary haemorrhage (0.6–5%) and macroscopic haematuria (1–2%).6,9,14,15 The treatment of thrombocytopenia, particularly in the context of platelet transfusion thresholds, remains controversial, but considerable progress has been made in recent years as a result of carefully designed clinical observational and intervention trials.16,17 In the majority of babies, thrombocytopenia resolves spontaneously with few long‐term sequelae. This means that expensive and time‐consuming investigations are usually not necessary provided that a clinical diagnostic algorithm, such as those shown in Figs 4.1 and 4.2 for term and preterm neonates, respectively, is followed. For preterm neonates, we have found that a practical approach is to stratify the causes of thrombocytopenia according to the postnatal age of the neonate at presentation and the gestational age, both of which can guide the clinician in making the right clinical and investigative choices. Fig. 4.1 Algorithm for the management of thrombocytopenia in a term baby. ITP, autoimmune thrombocytopenia; FNAIT, fetal/neonatal alloimmune. thrombocytopenia. Fig. 4.2 Algorithm for the management of thrombocytopenia in a preterm baby. Although neonatal thrombocytopenia can have a wide variety of causes, the clinical presentation can broadly be divided into three groups depending on the age at presentation: The main causes of thrombocytopenia presenting in fetal life are shown in Table 4.1 and include mainly constitutional abnormalities and congenital infection. Although many of these disorders may also present after birth, in practice by far the most common causes of thrombocytopenia presenting in the first 72 hours of life are related to complications of pregnancy and/or delivery (Table 4.2). In contrast, the majority of episodes of thrombocytopenia developing later than 72 hours of life are a result of a postnatally acquired bacterial infection and/or NEC (Table 4.3). This classification provides clinicians with guidance for selecting appropriate investigations and management and helps to avoid unnecessary and expensive tests in transient thrombocytopenias where prognosis can be determined by simple clinical and laboratory means alone (Figs 4.1 and 4.2). Table 4.1 Causes of fetal thrombocytopenia ITP, autoimmune thrombocytopenia; SLE, systemic lupus erythematosus. Table 4.2 Causes of early‐onset neonatal thrombocytopenia (presenting before 72 hours) CAMT, congenital amegakaryocytic thrombocytopenia; CMV, cytomegalovirus; HIT, heparin‐induced thrombocytopenia; HIV, human immunodeficiency virus; HLH, haemophagocytic lymphohistiocytosis; ITP, autoimmune thrombocytopenia; IUGR, intrauterine growth restriction; PET, pre‐eclamptic toxaemia; SLE, systemic lupus erythematosus; TAM, transient abnormal myelopoiesis; TAR, thrombocytopenia with absent radii; TTP, thrombotic thrombocytopenic purpura; VWD von Willebrand disease. Table 4.3 Causes of late‐onset neonatal thrombocytopenia (presenting after 72 hours): the most frequently occurring conditions are shown in bold * Thrombocytopenia has been reported but thrombocytosis is more common. CAMT, congenital amegakaryocytic thrombocytopenia; CMV, cytomegalovirus; HIV, human immunodeficiency virus; ITP, autoimmune thrombocytopenia; SLE, systemic lupus erythematosus; TAR, thrombocytopenia with absent radii. Thrombocytopenia is usually identified when fetal blood sampling is performed where there is a history of fetal haemorrhage due to a known history of inherited thrombocytopenia. Other cases are identified during the course of investigations for suspected fetal anomalies or fetal anaemia. Fetal blood sampling also remains an important tool for the diagnosis of thrombocytopenia in suspected congenital cytomegalovirus (CMV) infection18 (discussed later) but is no longer used to monitor known fetal/neonatal alloimmune thrombocytopenia (FNAIT). The most common causes of fetal thrombocytopenia in individual centres tend to be influenced by the particular case‐mix of the local fetal medicine unit but will include viral infection and thrombocytopenia secondary to severe alloimmune anaemia due to Rh antibodies.3 The conditions presenting with thrombocytopenia in fetal life are discussed in more detail later. More than half of all episodes of thrombocytopenia in babies admitted to NICUs occur within the first 72 hours of life.8 The majority of cases of early‐onset thrombocytopenia in this setting occur in preterm neonates born following pregnancies complicated by placental insufficiency and/or chronic fetal hypoxia, secondary either to idiopathic intrauterine growth restriction (IUGR) in the fetus19 or to maternal disease, in particular pre‐eclampsia and other forms of pregnancy‐induced hypertension, diabetes mellitus or HELLP syndrome (haemolysis, elevated liver enzymes and low platelets).20 A large retrospective study of almost 3000 neonates with IUGR (also termed ‘small for gestational age’ [SGA]) showed that 30% of them had early‐onset thrombocytopenia compared with 10% of gestation‐matched neonates of appropriate birthweight.21 Neonates with IUGR also have a number of other characteristic haematological abnormalities at birth, including increased numbers of circulating nucleated red blood cells (NRBC) (Fig. 4.3), often with an associated increased haemoglobin concentration (Hb), haematocrit and red cell count; a variable degree of neutropenia without left‐shifted neutrophils or other signs of acute infection; and red cell changes on the blood film suggestive of hyposplenism, including spherocytes, target cells and Howell–Jolly bodies (see Fig. 1.10).19 The cause of the thrombocytopenia in IUGR appears to be reduced platelet production due to impaired megakaryocytopoiesis. The numbers of megakaryocytes and their precursor and progenitor cells are severely reduced and there is no clear evidence of increased platelet consumption.8,22,23 Similarly, the numbers of neutrophil progenitors are reduced, suggesting that the neutropenia results from reduced neutrophil production. By contrast, erythropoiesis is clearly increased, as shown by the high numbers of NRBC and the raised Hb. Furthermore, the levels of erythropoietin (EPO) are increased in fetuses and neonates with IUGR24,25 and correlate with the severity of the haematological abnormalities.19 These findings suggest that the underlying cause of the thrombocytopenia and other haematological abnormalities associated with IUGR is chronic fetal hypoxia. However, the mechanisms by which chronic fetal hypoxia affects platelet production as well as neutrophil and red cell production are unknown. Fig. 4.3 Blood film of a preterm neonate with intrauterine growth restriction showing erythroblastosis, polychromasia, poikilocytosis and target cells. No leucocytes and only occasional platelets are seen consistent with the blood count showing neutropenia and thrombocytopenia. At least two Howell–Jolly bodies are also apparent. May–Grünwald–Giemsa (MGG), ×20 objective. The natural history of this type of neonatal thrombocytopenia is characteristic. Affected neonates usually have a low normal or moderately reduced platelet count at birth (120–200 × 109/l), which falls to a nadir of 80–100 × 109/l at day 4–5 of life before recovering to >150 × 109/l by 7–10 days of age.26 This pattern, together with the clinical history and blood film abnormalities, is sufficient to make the diagnosis9 (see Figs 1.10 and 4.3). Christensen et al. showed that neonates with this pattern of IUGR‐associated early‐onset thrombocytopenia had a low morbidity and mortality (2%) compared with the SGA neonates with early‐onset thrombocytopenia for other reasons (65%).21 This supports a conservative approach to management of neonatal thrombocytopenia associated with IUGR where there is no other cause for the thrombocytopenia and where the platelet count remains above 50 × 109/l and recovers with the characteristic natural history outlined above. For these neonates, no further investigations are necessary. On the other hand, if the platelet count falls below 50 × 109/l or the thrombocytopenia persists beyond the 10th day of life, additional causes for the thrombocytopenia should be sought. In contrast to the mild to moderate thrombocytopenia seen in most cases of placental insufficiency, severe early‐onset thrombocytopenia (<50 × 109/l) requires urgent investigation. The three most important causes are FNAIT, which is discussed in detail later, perinatal bacterial infection and hypoxic ischaemic encephalopathy (HIE) due to acute perinatal asphyxia/hypoxia (see Table 4.2). Perinatal bacterial infection is commonly related to prolonged rupture of membranes and usually presents as early onset neonatal infection (present by 72 hours). Recent studies have shown that perinatal infection occurs in approximately 3.5 of 1000 births, most commonly due to group B Streptococcus or Escherichia coli.27,28 Infections with these organisms are a relatively common cause of stillbirth and can also cause serious neonatal morbidity, with thrombocytopenia developing in about 50% of cases.29,30 In contrast to late‐onset neonatal sepsis, disseminated intravascular coagulation (DIC) appears to be an important mechanism of thrombocytopenia, probably because of the severity of the sepsis syndrome induced by the causative organisms and the fact that infection has often begun prior to delivery and therefore before effective treatment can be instituted. For this reason it is important to perform a coagulation screen in any neonate with thrombocytopenia secondary to perinatal bacterial infection.31 Typically, the blood film will show neutrophil left shift with toxic granulation evident 1 or 2 days later (see Figs 3.3 and 3.6) and, when DIC is present, thrombocytopenia and fragmented red blood cells (schistocytes) (Fig. 4.4). Worsening thrombocytopenia and neutropenia are poor prognostic signs. Thrombocytopenia in association with HIE is often severe and prolonged and occurs in approximately 30% of affected neonate.32,33 In most cases, thrombocytopenia appears to result principally from DIC31 but it is also reported in the absence of DIC when it tends to be less severe.34 Several studies to investigate the impact of therapeutic induced hypothermia in thrombocytopenic neonates with HIE show no clinically significant effects on the platelet count.33 Late‐onset thrombocytopenia in preterm neonates in NICUs is often severe, with an acute fall in the platelet count over 24 hours, and may be prolonged. Most episodes are secondary to bacterial or fungal sepsis or NEC. Many, but not all, neonates with sepsis‐ or NEC‐associated thrombocytopenia have evidence of DIC (see Case 3.1) and so it is important to carry out a coagulation screen, including measurement of fibrinogen levels in all neonates with sepsis or NEC and a rapidly falling platelet count.35 D‐dimer measurement is not usually helpful in neonates because of the high physiological levels for the first month of life and additional blood samples are also often difficult to obtain in sick neonates. Importantly, thrombocytopenia may be prolonged for several weeks beyond the onset of sepsis/NEC. Sepsis‐ and NEC‐associated thrombocytopenias are among the commonest indications for neonatal platelet transfusion.36 Other causes of late‐onset thrombocytopenia are shown in Table 4.3 and include congenital infections, metabolic disorders, drug‐induced and immune thrombocytopenias and inherited disorders, all of which are discussed later. Fig. 4.4 Blood film of a preterm neonate during an acute bacterial infection showing two neutrophils with toxic granulation and some schistocytes together with thrombocytopenia consistent with disseminated intravascular coagulation. Note the large number of echinocytes consistent with the gestation at birth (24 weeks) and the Howell–Jolly body reflecting the underlying intrauterine growth restriction. MGG, ×100. Although the majority of cases of neonatal thrombocytopenia can be diagnosed by their clinical presentation and basic laboratory parameters, there are a small number of other conditions that lead to clinically significant thrombocytopenia that require specific investigation leading to diagnosis and appropriate management. Fetal/neonatal alloimmune thrombocytopenia is the commonest cause of severe thrombocytopenia in well, term infants. It is caused by transplacental passage of maternal anti‐human platelet antigen (HPA) alloantibodies directed against fetal platelet antigens that the mother lacks, leading to fetal platelet destruction and potentially also suppression of fetal megakaryopoiesis.37 In addition to bleeding caused by the thrombocytopenia, in vitro and mouse studies suggest that in some cases of FNAIT bleeding may be aggravated by impaired platelet function caused by binding of anti‐HPA antibodies to the receptors for fibrinogen on platelets and/or by defects in vascular integrity caused by anti‐HPA antibodies binding to vitronectin receptors on endothelial cells.38,39 Population‐based studies indicate an overall prevalence of FNAIT of 0.7 per 1000 births.40,41 Although 41 different HPA antigens have been identified so far (Versiti HPA Database, 2020. Available from: https://www.versiti.org/hpa‐gene), a recent international study found that four of these antigens were responsible for the vast majority of reported cases of FNAIT (antibodies to HPA‐1, HPA‐3, HPA‐5 and HPA‐15).42 In Caucasian populations, HPA‐1a is implicated in 80% cases and HPA‐5b in 10–15%.40,43,44 Around 10% of women who are HPA‐1a‐negative and carrying an HPA‐1a‐positive fetus have anti‐HPA‐1 antibodies.45 Although the frequency of anti‐HPA‐5b antibodies in HPA‐5b‐negative women carrying an HPA‐5b‐positive fetus is much higher (27%), the risk of severe FNAIT‐associated bleeding is lower with anti‐HPA‐5b than anti‐HPA‐1a.46 The development of antibodies against HPA‐1a in HPA‐1a‐negative women is very strongly associated with HLA DRB3 0101 (odds ratio 140), although the HLA DR genotype does not predict the severity of the FNAIT.45,47 Current evidence suggests that antibodies against low‐frequency antigens are rare and play little role in the morbidity of FNAIT in the Caucasian population.41,48 In Asian and African populations FNAIT caused by anti‐HPA‐1a antibodies appears to be uncommon. The most frequent causes of FNAIT in the Asian population are antibodies directed against HPA‐5b or anti‐HPA‐4b.49 In addition, studies from a number of countries have shown that FNAIT in Asian and African populations is frequently caused by maternal anti‐CD36 alloantibodies.50–53 While CD36 deficiency is extremely rare in Caucasians, around 2% of individuals of African origin and 0.5% of individuals of Asian origin lack CD36 expression on their platelets and monocytes and are therefore at risk of developing anti‐CD36 antibodies after platelet transfusion or during pregnancy.50–53 FNAIT can also occur in the babies of women with Glanzmann thrombasthenia or Bernard–Soulier syndrome who have been immunised by platelet transfusion, developing either HLA antibodies or antibodies to the missing glycoproteins.54 Most neonates with FNAIT present with severe thrombocytopenia, often with a platelet count <20 × 109/l. The most feared complication is ICH, which occurs in approximately 20% of neonates with HPA‐1a‐associated FNAIT and is associated with a very high risk of severe neurodevelopmental problems, including cerebral palsy.40,55 A large international study that identified 43 cases of FNAIT‐associated ICH reported that 15 (35%) neonates died within a few days of birth while another 23 (82% of the survivors) had long‐term neurodevelopmental problems, including cerebral palsy.56 A high proportion of ICH (about 80%) occurs in utero and FNAIT has been reported as early as 20 weeks’ gestation.56,57 Bleeding from extracranial sites, such as the gastrointestinal tract or the lungs may also occur and is occasionally fatal58 although the overall risk of major haemorrhage due to FNAIT is fairly low (<5%).42,59 Since FNAIT can affect first pregnancies, the diagnosis at birth is often unsuspected and the clinical presentation varies from asymptomatic thrombocytopenia or petechiae to seizures secondary to ICH. The clinical pattern of FNAIT due to anti‐CD36 antibodies is particularly associated with repeated early fetal loss due to miscarriage and hydrops fetalis, the antigen being also expressed on erythroid cells.60–63 Fig. 4.5 Blood film of a neonate born at 36 weeks’ gestation with severe thrombocytopenia due to fetal/neonatal alloimmune thrombocytopenia showing a single normal‐sized platelet as well as a normal neutrophil and red cells. MGG, ×100. The laboratory diagnosis of FNAIT is usually made using monoclonal antibody‐specific immobilisation of platelet antigens (MAIPA) assays to detect maternal anti‐HPA antibodies. Both parents, where possible, and the infant should also be genotyped for the most common HPA antigens (HPA‐1a, HPA‐3, HPA‐5b and HPA‐15) and HPA‐4 and CD36 if appropriate. Because the field of platelet genotyping is advancing rapidly, an international panel of experts recently worked together to agree evidence‐based guidance on the investigations of FNAIT using a systematic approach and standardised methodology.64 There are no specific haematological features of FNAIT apart from isolated thrombocytopenia. The only finding on the blood film is thrombocytopenia and often it is the absence of additional features (such as evidence of infection) that suggests the diagnosis (Fig. 4.5). This diagnosis should be considered in all cases of severe early‐onset neonatal thrombocytopenia (platelet count <50 × 109/l) (see Fig. 4.1). In a retrospective analysis of 166 cases of severe thrombocytopenia where screening for FNAIT was performed, two‐thirds of the neonates (110/166) were found to have FNAIT and 12 of these neonates were found to have ICH.65 The high mortality and morbidity of FNAIT‐associated ICH means that most experts recommend that all cases of suspected FNAIT should undergo cranial ultrasound scanning to exclude ICH. Most cases of FNAIT resolve within a week without long‐term sequelae. Since the platelet count usually falls over the first 4–7 days of life, all thrombocytopenic neonates with FNAIT should be monitored until there is a sustained rise in the platelet count into the normal range. There have been many changes in the recommendations for both postnatal and antenatal management of FNAIT over the last 10 years. This reflects the difficulty of conducting randomised trials in a relatively rare disease and most recommendations are based on consensus recommendations from expert groups. In well neonates with documented or suspected FNAIT who have no evidence of haemorrhage, transfusion of HPA‐compatible platelets is recommended only when the platelet count is <30 × 109/l.41,64 In some countries, including the UK, HPA‐1a‐, HPA‐5b‐negative platelets are usually given pending a precise diagnosis. A recent systematic review confirmed that HPA‐selected platelet transfusions were more effective than HPA‐unselected platelets.66 However, the same review also showed that random donor platelets were often clinically effective and could therefore be used in regions or countries where HPA‐selected platelets were unavailable. In the event of major haemorrhage, including ICH, the platelet count should be maintained >50 × 109/l using appropriate HPA‐negative platelets by preference. There is currently no clear evidence to demonstrate a benefit for administration of intravenous immunoglobulin (IVIg) in the immediate postnatal period.66 In all cases, platelets for transfusion should be CMV‐negative, single‐donor apheresis units; when neonates have received intrauterine platelet transfusions the platelets should also be irradiated.67 In some cases, thrombocytopenia persists for up to 8–12 weeks. In these cases IVIg is usually considered to be a better option than repeated platelet transfusions, although there is no evidence from clinical trials to confirm the value of IVIg for persistent thrombocytopenia.1 Although the optimal approach to antenatal management of FNAIT has been controversial for many years, a recently published systematic review of the 26 most relevant studies has clearly shown that non‐invasive antenatal management of FNAIT is as effective as fetal blood sampling‐based approaches.59 This is important because, as this review reported, the mortality associated with fetal blood sampling in FNAIT, is substantial (26%). This review also found that weekly maternal administration of IVIg is the most appropriate first‐line antenatal treatment of FNAIT, with no consistent evidence in favour of adding corticosteroids.59 Management of women with known HPA antibodies depends on the HPA genotype of the father and the severity of previously affected neonates. To confirm that there is a risk of FNAIT, HPA typing using non‐invasive methods should be performed in any pregnancy where the father is unavailable or is known to be heterozygous for the implicated antigen.64 Although there is some evidence that antibody titres correlate with disease severity, the single most important predictor of poor outcome is a past history of an affected infant with ICH. As a result, recent international recommendations advise that women with a previous baby with an ICH related to FNAIT should be offered IVIg infusions during subsequent affected pregnancies from 12 weeks’ gestation.64 Neonates born to mothers with a history of autoimmune thrombocytopenia (ITP) or systemic lupus erythematosus (SLE) may develop thrombocytopenia as a result of transplacental passage of maternal platelet autoantibodies even if the mother had a normal platelet count during the pregnancy. Severe thrombocytopenia (<50 × 109/l) occurs in about 10% of neonates with maternal platelet autoantibodies; about half of these have platelet counts of <20 × 109/l.68,69 Several studies have shown that the mortality and risk of major haemorrhage, including ICH, is very low (about 1%)68–70 and most groups no longer recommend fetal blood sampling or caesarean delivery irrespective of the maternal platelet count.68,71 Neonatal lupus due to transplacental passage of maternal autoantibodies to Sjögren syndrome A or B autoantigens has a distinct clinical presentation which includes total atrioventricular heart block as well as the characteristic lupus rash, hepatobiliary disease, thrombocytopenia, anaemia, neutropenia and, rarely, aplastic anaemia.72 The most useful factors predicting whether or not fetal or neonatal thrombocytopenia is likely to be severe are the severity of the maternal disease and/or platelet count during pregnancy, or the occurrence of severe thrombocytopenia in a previous baby.68,69 As with FNAIT, there are no specific features in the blood film of a baby with thrombocytopenia due to maternal autoimmune disease. All neonates with a history of maternal thrombocytopenia should have their platelet count checked at birth; if it is found to be >150 × 109/l, no further action is necessary. Thrombocytopenic neonates should have their platelet count rechecked after 2–3 days, as counts often drop to their lowest levels at this age. In addition, all neonates with severe thrombocytopenia should undergo cranial ultrasound scanning to exclude ICH. For neonates with a platelet count of <20 × 109/l or for thrombocytopenic neonates with active bleeding, the International Consensus Report on Management of ITP recommended treatment with a single dose of IVIg (1 g/kg).73 In most cases the thrombocytopenia resolves spontaneously by the age of 7 days. However, in a small group of neonates, thrombocytopenia persists up to the age of 12 weeks.69 In this situation, where the thrombocytopenia is severe (platelet count <20 × 109/l), treatment with IVIg (400 mg/kg/day for 5 days or 1 g/kg/day for 2 days, total dose 2 g/kg) may be useful.1,69 The classical congenital infections associated with fetal and neonatal thrombocytopenia are often referred to as ‘TORCH’ organisms (toxoplasma, other infections, rubella, CMV and herpes simplex). There is an expanding range of ‘other’ organisms including a range of viruses, mycobacteria and protozoa. Neonatal thrombocytopenia does not appear to be a feature of transplacental infection with severe acute respiratory syndrome coronavirus‐2 (SARS‐CoV‐2)74 and is only rarely described in neonates with congenital human immunodeficiency virus (HIV) infection.75 In most cases, thrombocytopenia will be present in combination with other clinical features suggestive of congenital infection, such as intracranial calcification, hepatosplenomegaly, jaundice or ‘reactive’ lymphocytes in the blood film (see Fig. 3.16). However, severe thrombocytopenia (platelet count <50 × 109/l) that is present in the first days of life and persists for more than the first week is a common feature in congenital infections and may then suggest the diagnosis if other more common clinical features are absent or minimal. The best recognised, and likely commonest, cause of virus‐associated neonatal thrombocytopenia is congenital CMV infection (Case 4.1, see page 227). This is also the most commonly identified congenital viral infection, affecting hundreds of thousands of neonates worldwide every year with an estimated prevalence of 0.67% overall.76,77 Rates of congenital CMV infection are 3‐fold higher in low‐ and middle‐income countries than in high‐income countries.77 The rate of congenital CMV infection largely depends on maternal seroprevalence rates: the highest rates of congenital CMV are seen in populations with high maternal seroprevalence because the majority of affected neonates are currently born to mothers with reactivation of previously acquired CMV or reinfection with a new viral strain.77 Maternal‐to‐fetal transmission of CMV is highest in the third trimester but the severity of infection is highest when transmission occurs during the first trimester.78 Overall, primary or recrudescent maternal CMV infection during pregnancy results in intrauterine transmission of CMV in 30–40% of cases, although only 10–15% of these cases are born with clinical abnormalities.76,79 Around 70% of neonates with congenital CMV disease have thrombocytopenia at birth, which often manifests as a petechial rash and occasionally as a ‘blueberry muffin’ skin rash (see Chapter 3). CMV‐associated thrombocytopenia may be severe and may persist for several months.76,80 The diagnosis is often suggested by the combination of the petechial rash with jaundice, hepatosplenomegaly, raised transaminases, IUGR, microcephaly and/or chorioretinitis as well as the characteristic appearance of intracranial periventricular calcification.76 Sensorineural hearing loss is an important cause of long‐term morbidity.81 The most severe cases of congenital CMV infection are detected during fetal life, usually as a result of routine ultrasound scanning. In these cases the majority of fetuses with severe neurological abnormalities are thrombocytopenic and the outlook is poor.18 The diagnosis of congenital CMV infection in neonates is based on the detection of CMV deoxyribonucleic acid (DNA) in urine or saliva using the polymerase chain reaction (PCR), which is quicker and more sensitive than viral culture.82 It is important to make the diagnosis on samples collected soon after birth to differentiate congenital from nosocomially acquired CMV infection because of the implications for screening and follow‐up of neonates with congenital CMV.76,83 Although there appear to be no direct long‐term haematological consequences of congenital CMV infection, the severity of fetal thrombocytopenia is likely to contribute to the considerable morbidity of this disease: up to 60% will have some form of long‐term neurodevelopmental disability, three‐quarters will have hearing impairment and one‐third will have visual problems, including blindness.76 Prenatal diagnosis of CMV infection is currently based on quantification of CMV DNA in amniotic fluid rather than fetal blood because of the potential risk of fetal blood sampling.84 However, it is important to be aware that the sensitivity of CMV PCR is maximal (90%) when amniocentesis is performed after the first 20 weeks of pregnancy and at least 8 weeks after the onset of maternal infection and that a negative result therefore does not rule out CMV infection of the fetus.84 Antiviral treatment with valganciclovir has been shown to be beneficial for hearing loss and neurodevelopmental outcome in symptomatic cases but is not recommended for asymptomatic CMV infection.85 Congenital rubella, though now very rare in countries with an active immunisation programme,86 causes severe disease (congenital rubella syndrome) in an estimated 105 000 children per year worldwide, mostly in Southeast Asia,87 although World Health Organization (WHO) estimates are lower than this. Occasional cases of congenital rubella syndrome still occur in high‐income countries, including cases where thrombocytopenia is a prominent feature.88 The frequency of thrombocytopenia due to rubella infection is difficult to determine because blood counts are often not reported. Estimates vary from 10% to 90%,88–91 with the majority of studies indicating that more than 50% of cases are associated with neonatal thrombocytopenia. A review of a rubella outbreak in the USA in 1964 reported that more than 90% of neonates with congenital rubella syndrome had thrombocytopenia.91 A retrospective study of severe cases in Japan after a rubella outbreak noted that 76% of neonates had thrombocytopenia90 and two of the three cases reported in the USA in 2012 also had thrombocytopenia.92 Infection results from transplacental infection due to primary infection of the mother, with the major risk occurring in the first trimester. As in many congenital infections, the diagnosis is usually suggested by the associated clinical features which, for rubella, are IUGR, sensorineural hearing loss, cardiac defects, hepatosplenomegaly, retinopathy, cataracts and, occasionally, a ‘blueberry muffin’ skin rash.87 In neonates the diagnosis is confirmed by PCR for viral DNA and/or virus culture and/or positive rubella‐specific immunoglobulin M (IgM) antibodies.88 Prenatal diagnosis is made by PCR for rubella virus DNA on amniotic fluid, chorionic villus tissue or fetal blood.93 There is no specific treatment for congenital rubella syndrome or the associated thrombocytopenia. Congenital parvovirus B19 infection, although more usually associated with isolated fetal anaemia, also not infrequently causes fetal and neonatal thrombocytopenia in addition to anaemia.71 As with congenital CMV and rubella infection, the likely mechanism of thrombocytopenia is reduced platelet production although the evidence for this is sparse. Thrombocytopenia secondary to congenital mumps infection is also reported but appears to be extremely rare.94 By contrast, in congenital herpes simplex infection, thrombocytopenia is due to the associated DIC and occurs where there is disseminated disease.95 In addition, severe coagulopathy is an important cause of fatality in neonatal herpes simplex infection.95 Similarly, thrombocytopenia secondary to congenital infection with enteroviruses (coxsackievirus A and B and echovirus) is due to DIC (Case 4.2, see page 230)96,97 and is a key indicator of severe disease.98 Importantly, neonatal enterovirus infection may also be complicated by haemophagocytic lymphohistiocytosis (HLH)99,100 or by bone marrow failure.101 Sporadic reports describe the successful treatment of enterovirus‐associated HLH or of other severe enterovirus infection in neonates by IVIg.98,100 Thrombocytopenia is also reported in association with neonatal adenovirus infection, although this is relatively uncommon.102 A review of hospitalised neonates in Dallas, Texas, USA identified 26 cases over a 17‐year period of which 5 (19%) had disseminated disease and a poor outcome while all of the cases with localised respiratory tract disease survived.102 Notably, thrombocytopenia without any evidence of coagulopathy was present in 4 of 5 neonates with disseminated disease compared with 3 of 19 with localised disease, suggesting that adenovirus infection itself may cause thrombocytopenia directly rather than in conjunction with DIC. Congenital arbovirus infections, such as dengue, chikungunya virus and zika virus, have also all been reported to cause thrombocytopenia and occasionally to underlie the subsequent development of HLH.103,104 In a recent series of 32 cases of neonatal dengue, almost 90% of the neonates had a petechial rash and around half had severe thrombocytopenia (platelet count <20 × 109/l).105 Although most cases have only mild bleeding despite severe thrombocytopenia,105 ICH and gastrointestinal haemorrhage have been reported.106,107 Importantly, the platelet count may be normal at birth before dropping rapidly during the febrile phase of the disease.108 This suggests that dengue‐associated thrombocytopenia is secondary to increased platelet consumption or destruction. Interestingly, in the series reported by Nguyen and colleagues,105 just over 10% of the neonates were initially misdiagnosed as neonatal immune thrombocytopenia. Thrombocytopenia is similarly common in congenital chikungunya virus infection. In one of the largest case series, 17 of 19 neonates reported in a study from La Réunion had thrombocytopenia; this was severe in over half of the cases and associated with DIC in half of these.109 Several of these neonates had major haemorrhage including ICH and gastrointestinal haemorrhage although none died. A recent systematic review which included 28 case reports, 14 small series and a total of 266 cases of congenital chikungunya virus infection found that thrombocytopenia was reported in around one‐third of the cases and, in contrast to neonatal dengue, was often associated with major haemorrhage, including ICH, gastrointestinal and pulmonary haemorrhage.110 This supported the findings of Gerardin et al.109 Thrombocytopenia is also an occasional feature of congenital zika virus infection.111 Congenital parasitic infection is an uncommon cause of neonatal thrombocytopenia but remains important because of the importance of prompt diagnosis and treatment to prevent further damage. Congenital toxoplasmosis affects between 1 in 2000 and 1 in 3000 newborns, depending on geographical location and dietary practices.112–114 In contrast to many infections, the risk to the fetus increases with the gestation at which the infection occurs and can be as high as 70% in the third trimester.115 The classical clinical features of congenital toxoplasmosis are fever, jaundice, hepatosplenomegaly, central nervous system (CNS) disease (microcephaly, intracranial calcification) and ocular disease (microphthalmia, chorioretinitis, cataracts).116 The incidence of thrombocytopenia in affected neonates is approximately 40%,113 which is similar to the percentage of fetuses with toxoplasmosis with platelets of less than 100 × 109/l.117 The mechanism of toxoplasmosis‐associated thrombocytopenia is likely to include a combination of reduced platelet production and increased platelet consumption as several cases of associated DIC have been reported in severely affected fetuses and neonates.117–119 Occasional cases of ‘blueberry muffin’ skin rash due to congenital toxoplasmosis have also been reported.120 The recommended treatment for congenital toxoplasmosis is prolonged (12 months) and includes pyrimethamine and sulfadiazine together with folinic acid to prevent pyrimethamine‐associated haematological toxicity.116 Follow‐up during treatment should include regular full blood counts because almost half of the infants will develop treatment‐related haematological toxicity, including thrombocytopenia, neutropenia and anaemia.121 Congenital malaria occurs with all malarial species and remains a common clinical problem in endemic areas122 as well as an occasional cause of congenital infection in non‐endemic countries, where lack of awareness may delay diagnosis.123,124 The most frequent clinical features are anaemia, fever, hepatosplenomegaly, jaundice and thrombocytopenia, which is often severe.123–128 The first clinical signs usually appear at 10–28 days after birth but may be delayed by up to 8 weeks.129 Neonates born to mothers who have been successfully treated for malaria do not need to be screened for congenital malaria unless they develop signs of the disease. Where the mother has not been successfully treated, both the neonate and the placenta should be examined for malarial parasites. Although rare, both congenital tuberculosis and congenital syphilis remain clinical problems because of the high associated mortality if the diagnosis is delayed; both may cause thrombocytopenia.130,131 In recent years the term ‘perinatal’ tuberculosis has largely replaced ‘congenital’ because it is often unclear whether the infection was acquired during the pregnancy or at delivery. While neonates with tuberculosis usually present with non‐specific signs of fever, hepatomegaly and respiratory distress, thrombocytopenia in association with DIC has been reported.132 As in congenital malaria, neonates with tuberculosis usually present at the age of 2–4 weeks and the diagnosis is often difficult, requiring microbial specimens from multiple sites, including gastric aspirates, lymph nodes, endotracheal secretions, bone marrow and/or cerebrospinal fluid.130 The numbers of cases of congenital syphilis remain low in many countries131 but a recent increase in some countries, including in the USA, means that it remains a concern in view of the high morbidity and mortality.133 Neonates with congenital syphilis typically present 3–4 weeks after birth with clinical features that include rhinitis, hepatosplenomegaly, fever, jaundice, anaemia, thrombocytopenia and a characteristic maculopapular rash on the palms and soles that becomes copper‐coloured with desquamation.134 Thrombocytopenia may be the only sign of disease.134 However, congenital syphilis may also present with hydrops fetalis131,134 and a study of fetal syphilis has shown that just over one‐third of fetuses have thrombocytopenia.135 The hepatosplenomegaly results from infiltration by the causative organisms (Treponema pallidum)136,137 and/or extramedullary haemopoiesis and some affected neonates also have a ‘blueberry muffin’ rash.131 Fetal thrombocytopenia is common in aneuploidy. Hohlfeld et al.117 reported frequencies of 86% for trisomy 18, 31% for trisomy 13, 75% for triploidy and 31% for Turner syndrome at fetal diagnosis. Thrombocytopenia was rarely severe, with a platelet count of less than 50 × 109/l seen in only one of the 43 fetal cases reported. Thrombocytopenia is also common in neonates with trisomy 13 and trisomy 18. Wiedmeier and colleagues reported that thrombocytopenia was the most common haematological abnormality detected in a retrospective study of 50 neonates (22 with trisomy 13 and 28 with trisomy 18), although full blood counts were only performed in 24 of them.138 Of the 12 neonates with trisomy 13 where a platelet count was obtained, 9 (75%) were thrombocytopenic but only 1 developed severe thrombocytopenia (platelet count less than 50 × 109/l). Similarly, of the 12 neonates with trisomy 18, thrombocytopenia was recorded in 10 (83%) and almost all the platelet counts were more than 50 × 109/l. Thrombocytopenia is also reported in neonates with partial trisomies, such as isochromosome 18q.139 The natural history of thrombocytopenia associated with trisomy 13 or trisomy 18 is not known because the vast majority of neonates do not survive beyond the first few months of life.140 The mechanism of the thrombocytopenia is also not known, although the pathogenesis may be similar to that seen in neonates with IUGR, given that the majority of neonates with trisomy 13 and trisomy 18 also have IUGR. However, in contrast to the high Hb and NRBC values seen in neonates with IUGR, the majority of neonates with trisomy 13 and trisomy 18 had normal Hb and NRBC values.138 The most common chromosomal abnormality associated with neonatal thrombocytopenia is trisomy 21 (Down syndrome). A recent large prospective study reported thrombocytopenia in 51.8% of a cohort of 200 neonates.141 Approximately 10% of neonates with Down syndrome develop a clonal pre‐leukaemic condition called transient abnormal myelopoiesis (TAM), also known as transient myeloproliferative disorder, which is characterised by increased peripheral blood myeloblasts and megakaryoblasts, abnormal megakaryocytes and variable thrombocytopenia (discussed in more detail in Chapter 3). TAM is caused by somatic mutations in the GATA1 gene, which encodes a transcription factor essential for normal red blood cell and platelet production. An additional 15% of Down syndrome neonates have a clinically and haematologically silent disease (‘silent TAM’) in which the percentage of peripheral blood blasts is ≤10% and the mutant GATA1 clone is small.141 However, as the frequency of neonatal thrombocytopenia in Down syndrome is the same whether GATA1 mutations are present or not, the presence of thrombocytopenia in a neonate with Down syndrome is not evidence suggestive of TAM or silent TAM. The number of known causes of inherited thrombocytopenia has grown very rapidly in recent years with increasing use of next generation sequencing and the formation of national and international consortia that together facilitate the identification and characterisation of previously unexplained familial thrombocytopenias.142,143 Almost 100 bleeding, thrombotic and platelet disorder genes have now been definitively identified and validated for clinical diagnostics144 (see also https://www.isth.org/page/GinTh_GeneLists). In neonates, inherited thrombocytopenias typically present either in the context of a more complex genetic disorder where the main features at birth are congenital anomalies (syndromic inherited thrombocytopenias) or they present with isolated thrombocytopenia, usually because of bleeding but sometimes as an incidental finding (reviewed in references 3 and 145). In the majority of cases, thrombocytopenia occurs as a result of impaired megakaryopoiesis and/or abnormal stem cell development. These include several of the disorders known collectively as inherited bone marrow failure syndromes (IBMFS), such as Fanconi anaemia and dyskeratosis congenita, which may present with thrombocytopenia even though the other haemopoietic lineages are also affected. Although the IBMFS very rarely have haematological problems in the neonatal period, the pattern of congenital anomalies present at birth may suggest the diagnosis and prompt specific investigations.146 In the absence of congenital anomalies, a key clue to a diagnosis of inherited thrombocytopenia is thrombocytopenia that is present at birth and that persists in the absence of other causes for the thrombocytopenia, such as sepsis or NEC. The principal inherited thrombocytopenias that present in the neonate, rather than later in childhood, are discussed here (see also Table 4.4 for disorders presenting with isolated thrombocytopenia, Table 4.5 for syndromic disorders and Table 4.6 for inherited thrombocytopenias associated with leukaemia and bone marrow failure). All of these disorders are rare. However, precise diagnosis has become more straightforward with the introduction of next generation sequencing into routine diagnosis in many countries although great care has to be taken to make sure that novel variants are fully validated.143,144,147 Since several of these disorders include increased susceptibility to other severe haematological disorders, including bone marrow failure and leukaemia, it is important to establish the genetic basis for any unexplained familial thrombocytopenia whenever possible (reviewed in references 148 and 149). Table 4.4 Inherited thrombocytopenias that may present in neonates as isolated thrombocytopenia With the exception of Bernard–Soulier syndrome (BSS), most of the inherited thrombocytopenias that present as isolated thrombocytopenia (Table 4.4) are rarely recognised in the neonatal period unless there is a family history. In all of these disorders the severity of bleeding correlates with the degree of thrombocytopenia unless there is associated platelet dysfunction as seen, for example, in BSS and the grey platelet syndrome.150 Table 4.5 Syndromic inherited thrombocytopenias that may present in the neonatal period CNS, central nervous system; IUGR, intrauterine growth retardation. Bernard–Soulier syndrome, one of the best recognised inherited platelet disorders, not infrequently presents with mild or moderate thrombocytopenia in the neonatal period. BSS is usually an autosomal recessive disorder caused by qualitative or quantitative defects of the glycoprotein (Gp)Ib‐IX‐V complex, a platelet receptor for von Willebrand factor (VWF), due to mutations in one of the genes encoding components of the complex.145 To date, bi‐allelic mutations in three of these genes – GP1BA, GP1BB and GP9 – have been described.151 Occasional cases of autosomal dominant BSS have also been reported.152 Table 4.6 Inherited thrombocytopenias associated with predisposition to leukaemia, bone marrow failure or both ALL, acute lymphoblastic leukaemia; AML, acute myeloid leukaemia. Bleeding is not usually severe in the neonatal period but can occur and is typically mucocutaneous, such as bruising, purpura or bleeding associated with minor procedures.153,154 The key diagnostic sign of BSS is the presence of macrothrombocytopenia on automated analysers and the presence of platelets that often appear larger than, or the same size as, red blood cells in the blood film.151 The platelet count is usually in the 20–100 × 109/l range and often fluctuates.155 The automated platelet count may underestimate the true platelet count because of the large size of the platelets, and where there is a discrepancy with the blood film appearance, the platelet count may need to be performed manually. The other most useful initial investigation in the neonatal period is demonstration of reduced expression of the GpIb‐IX‐V complex by platelet flow cytometry using markers for GpIX (CD42a) and GpIbα (CD42b).156 Treatment by platelet transfusion is effective but should be reserved for life‐threatening haemorrhage since transfused patients may form alloantibodies against GpIb, GpIX or GpV.156 Indeed, platelet alloantibodies are a cause of FNAIT in offspring of mothers with BSS.157 Around half of the known genetic causes of isolated thrombocytopenia have been known to present occasionally in neonates with a history of bleeding and/or thrombocytopenia, including FLI1‐related thrombocytopenia,158 FYB‐related thrombocytopenia,159 grey platelet syndrome due to mutations in NBEAL2,160 ITGA2B/ITGB3‐related thrombocytopenia161 and PRKACG‐related thrombocytopenia.162 Mutations in the GFI1b and SLFN14 genes can also present in early childhood, with at least one case of each disease reported to have had recurrent episodes of minor bleeding since infancy.163,164 Other, usually milder, autosomal dominant forms of inherited thrombocytopenia, such as ACTN1‐related, CYCS‐related and IKZF5, THPO, TRPM7, TPM4 and TUBB1‐related thrombocytopenia have not yet been documented to present in the neonatal period.145,165 A number of inherited thrombocytopenias can present with syndromic features during the neonatal period (Table 4.5). These include Wiskott–Aldrich syndrome and its variants, X‐linked thrombocytopenia due to GATA1 mutations, thrombocytopenia with absent radii (TAR) syndrome, Stormorken syndrome and FLNA‐associated thrombocytopenia (reviewed in references 145 and 149). For other disorders, such as Chédiak–Higashi syndrome (CHS), the disease usually presents later in infancy although neonatal cases have been reported.166,167 Chédiak–Higashi syndrome is caused by bi‐allelic mutations in the lysosomal trafficking regulator (LYST) gene168 and carries a high risk of progression to HLH.167 A recent single‐centre study of 14 patients found that one‐third of the cases presented at or within 1 month of birth and that the median age at which clinical signs appeared was 2 months.167 CHS is characterised by a combination of partial oculocutaneous albinism, photosensitivity and frequent bacterial infections. Often the first clinical signs are the abnormalities in skin and hair colour and 13 of 14 children in the recent study by Carneiro et al.167 were noted to have silvery hair. Bleeding problems usually develop in infants rather than the newborn, although occasional cases have been reported in association with a known family history.166 Bleeding is generally attributed to the platelet storage pool defect rather than thrombocytopenia, which may not develop until much later.169 The diagnosis is established by the presence of giant granules within neutrophils in a peripheral blood film and is confirmed by molecular genetic testing for LYST mutation. This rare X‐linked disorder is caused by mutations in the FLNA (filamin A) gene and has been clearly shown to cause thrombocytopenia in adults.170,171 However, FLNA‐associated disease in neonates presents with severe interstitial lung disease together with otopalatodigital spectrum disorders, congenital heart disease and/or brain periventricular nodular heterotopia. The perinatal mortality due to these non‐haematological complications is very high and the presence of thrombocytopenia is not reported in most of these cases even though it may be a feature in adult survivors.172 The absence of a bleeding history, despite many of these affected neonates being extremely unwell, suggests that even if thrombocytopenia is present in the neonatal period it is unlikely to be clinically significant.171,172 The Paris–Trousseau syndrome describes the congenital platelet disorder found in more than 90% of patients with Jacobsen syndrome; the remaining 10% or so of patients having syndromic features but without the platelet abnormalities.173 Jacobsen syndrome is caused by deletion of part of the long arm of chromosome 11 (11q23.3); this contains the FLI1 and ETS1 genes but their role in the pathogenesis of the condition is not clear.158 The platelet disorder is characterised by macrothrombocytopenia with dysmegakaryopoiesis. More than 25 cases have been reported in which presentation of Jacobsen syndrome was before or shortly after birth; the majority of these were thrombocytopenic.174,175 The severity of the clinical manifestation depends on the extent of the 11q23 deletion and can include IUGR, developmental delay, malformations of the heart, gastrointestinal tract, urinary tract and CNS, and immunodeficiency as well as thrombocytopenia.173,176 Around 20% of patients die during the first 2 years of life either from congenital heart disease, which is often severe, or less commonly from bleeding.176 As with several other inherited platelet disorders, in infants that survive the platelet count may spontaneously improve over the first few years of life, although the long‐term outlook is unknown.176 A number of rare giant platelet syndromes caused by mutations in the MYH9 gene can present with thrombocytopenia in the neonate or fetus.177 The MYH9‐related diseases include the conditions previously known as May–Hegglin anomaly, Epstein syndrome, Fechtner syndrome and Sebastian syndrome. These syndromes are autosomal dominant and characterised by macrothrombocytopenia and a high risk of developing deafness (about 60%), nephropathy (about 30%) and cataracts (about 20%) during childhood or later in life.177 The platelet count varies from less than 20 × 109/l up to normal, but is usually 40–80 × 109/l. Although MYH9‐related thrombocytopenia usually presents in older children, thrombocytopenia may be severe enough to cause fetal or neonatal ICH,178,179 leading to misdiagnosis as FNAIT.180 The diagnosis is made by observation of Döhle‐like bodies in neutrophils together with giant platelets, which may cause inaccurate automated platelet counts. Both Stormorken syndrome and York platelet syndrome are caused by heterozygous gain‐of‐function mutations in the STIM1 gene, which encodes a calcium channel regulating protein. Both syndromes are characterised by tubular aggregate myopathy, thrombocytopenia, hyposplenism or asplenia, miosis, short stature, migraine and ichthyosis and have variable presentations in different patients. Several families in which the thrombocytopenia was present in the neonatal period have been described and where the thrombocytopenia preceded signs of myopathy both in Stormorken syndrome181 and in York platelet syndrome.182 In these cases the neonatal thrombocytopenia was severe and in one case was accompanied by petechiae and intraventricular haemorrhage. Electron microscopy of platelets from patients with York platelet syndrome shows characteristic ultrastructural platelet abnormalities including giant organelles and multi‐layered target bodies as well as deficiency of platelet calcium storage in the delta granules.182 Loss‐of‐function mutations in STIM1 are also reported but cause a different spectrum of disease characterised by immunodeficiency, muscle hypotonia, hepatosplenomegaly, autoimmune haemolytic anaemia and intermittent, immune‐related thrombocytopenia which has occasionally been reported to present within the neonatal period.183 Thrombocytopenia with absent radii (reviewed in reference 3) is characterised by bilateral absence of the radii; both thumbs are present, which may be useful in making a distinction from Fanconi anaemia. Most babies with TAR syndrome develop thrombocytopenia within the first week of life. The platelet count is usually less than 50 × 109/l and the white cell count is elevated in more than 90% of patients, sometimes exceeding 100 × 109/l and mimicking congenital leukaemia. There may be anaemia and eosinophilia. There is a high frequency of associated abnormalities, particularly cow’s milk intolerance, facial dysmorphism and lower limb anomalies.184 Although older studies have reported high infant mortality in TAR syndrome, in the study by Greenhalgh and colleagues only 2 of the 34 patients died and only 1 of these was due to major bleeding (ICH),184 while a recent study of 26 cases of TAR documented only a single death, due to neonatal sepsis at the age of 3 weeks.185 Those infants that survive the first year of life generally do well, because the platelet count improves spontaneously and is usually maintained at low normal levels thereafter. Inheritance of the TAR syndrome is complex. Both apparently autosomal recessive and autosomal dominant inheritance patterns occur. The majority of cases of apparently autosomal recessive disease are due to coexistence of a microdeletion (either inherited or de novo) of chromosome 1q21 including the RBM8A gene and one of two rare polymorphisms in the non‐coding region of the other RBM8A gene, a gene which is involved in RNA processing.185,186 However, occasional children with the same molecular abnormalities and a TAR‐like phenotype but without neonatal thrombocytopenia have recently been described187 and it is not clear how this genetic abnormality causes the thrombocytopenia and non‐haematological features of TAR syndrome. Wiskott–Aldrich syndrome and X‐linked thrombocytopenia (XLT) together form a spectrum of disorders caused by mutations in the Wiskott–Aldrich syndrome protein gene (WAS) on the short arm of the X chromosome (Xp11‐12). Over 300 different mutations have been identified.188 The syndrome typically presents as microthrombocytopenia, eczema, recurrent infections and/or increased susceptibility to autoimmune disorders in a male infant.189 Although Wiskott–Aldrich syndrome typically presents in infancy, some cases do present with thrombocytopenia in the neonatal period, usually without bleeding,190,191 although bleeding after circumcision in the neonatal period has been reported.192 Later in infancy, the bleeding may be severe and may include gastrointestinal haemorrhage and purpura.189 Bleeding is due both to the degree of thrombocytopenia and to abnormal platelet function. XLT causes a milder, isolated thrombocytopenia.193 In addition to contributing to TAM and acute megakaryoblastic leukaemia seen specifically in children with Down syndrome (see earlier), GATA1 mutations also cause two different forms of inherited thrombocytopenia, which differ mainly in their associated red cell abnormalities: XLT with dyserythropoiesis and XLT with β thalassaemia (reviewed in reference 194). XLT with dyserythropoiesis, which is caused by mutations in GATA1 that affect binding to its cofactor FOG1, can present either at birth, with bleeding secondary to severe thrombocytopenia, or as fetal hydrops secondary to the dyserythropoietic anaemia.195,196 XLT with β thalassaemia is caused by mutations in the N‐terminal zinc finger of GATA1, which affects DNA binding,194 and presents towards the end of the first year of life rather than in the neonatal period.197,198 Mutations in a number of other genes with a wide variety of clinical manifestations, including thrombocytopenia, have also been identified, including those in the ACTB, ARPC1B, CDC42, GALE, KDSR and MPIG6B genes.149 Although thrombocytopenia during the first year of life is reported for most of these disorders, information about the presentation of these disorders, which are all very rare, in the neonatal period is sparse. There is a range of inherited thrombocytopenias that are now known to predispose to other severe haematological disorders, including leukaemia, bone marrow failure (aplastic anaemia) and myelofibrosis (Table 4.6). Almost all patients with congenital amegakaryocytic thrombocytopenia (CAMT) and an unknown proportion of those with radio‐ulnar synostosis with amegakaryocytic thrombocytopenia (RUSAT1) progress to bone marrow failure,199,200 as does MECOM‐associated syndrome.201 All of these disorders are briefly summarised below as they typically present during the neonatal period. Several disorders that initially present as isolated thrombocytopenia have a very significant risk of subsequent evolution to acute leukaemia, particularly familial platelet disorder with propensity to acute myeloid leukaemia (FPD/AML),202,203 ETV6‐related thrombocytopenia204 and ANKRD26‐related thrombocytopenia.177,205 These disorders more often present later in childhood unless a family history leads to specific screening tests and earlier diagnosis. Congenital amegakaryocytic thrombocytopenia is an autosomal recessive disorder that typically presents at birth with petechiae and/or other evidence of bleeding, thrombocytopenia and morphologically normal platelets.199 The platelet count is usually less than 20 × 109/l at birth and the bone marrow shows normal cellularity with a specific reduction in megakaryocytes. A recent series of 56 patients with CAMT found that 43 (77%) had thrombocytopenia at birth and most of the affected babies had purpura and/or petechiae.206 However, 10% of the neonates had severe bleeding including ICH and haematemesis. Physical anomalies are present in around 50% of babies, including CNS and eye anomalies, developmental delay and craniofacial abnormalities.206 Although the thrombocytopenia in CAMT is usually isolated, in the series described by Germeshausen and Ballmaier,206 4 of 56 patients (7%) were also anaemic at birth. In the majority of patients CAMT is caused by mutation in the MPL gene, encoding the thrombopoietin receptor (reviewed in references 200 and 206) or, more rarely, in the thrombopoietin gene (THPO).207 Diagnosis by platelet flow cytometry is feasible but unreliable and genetic confirmation is advisable both because of the risk of leukaemia and aplasia and also to identify cases with mutations in THPO, as these children respond to treatment with thrombopoietin mimetics.207 Almost all CAMT patients subsequently develop aplastic anaemia and the only curative treatment is haemopoietic stem cell transplantation, which is successful in around 80% of cases.208 Occasional cases of clonal chromosomal abnormalities have been described,209 suggesting that these children may be at risk of developing myelodysplasia and acute myeloid leukaemia (AML) but the only case of leukaemia reported to date was a B acute lymphoblastic leukaemia (ALL).210 Rarely, pancytopenia develops in the first month of life.211 The only treatment for CAMT in the neonatal period is platelet transfusion for bleeding episodes. This group of inherited thrombocytopenias carry a risk of subsequent development of leukaemia that varies from over 40% in FPD/AML, which is caused by mutations in the RUNX1 transcription factor gene, to 25–30% in ETV6‐related thrombocytopenia and 8–10% in ANKRD26‐related thrombocytopenia.177202–205 All three disorders are autosomal dominant and present with mild to moderate thrombocytopenia with little or no associated bleeding.212 Although most cases present in adulthood, cases of persistent neonatal thrombocytopenia are reported,213,214 the diagnosis often prompted by a family history that leads to a full blood count being performed.215 The first comprehensive RUNX1 germline registry has recently been established to act as a reference source to assess the clinical and prognostic significance of RUNX1 variants, including those identified in FPD/AML cases.216,217 The leukaemias generally present in adulthood and, in families with ETV6‐related thrombocytopenia and FPD/AML, ALL is reported as well as AML and myelodysplastic syndromes.204,215 As thrombocytopenia‐related bleeding is usually mild or absent, the main value of making the diagnosis in the neonatal period is to put in place haematological follow‐up and accurate genetic counselling.212 Patients with amegakaryocytic thrombocytopenia and radio‐ulnar synostosis (ATRUS) present at birth with severe thrombocytopenia, virtually absent bone marrow megakaryocytes and characteristic skeletal abnormalities. In addition to the radio‐ulnar synostosis, affected infants may have clinodactyly and shallow acetabulae. Two kindreds show that ATRUS is caused by mutation in the HOXA11 gene that results in disruption of DNA binding.218 A second disorder in which radio‐ulnar synostosis is associated with inherited thrombocytopenia is MECOM syndrome due to mutations in MECOM, the gene that encodes the transcriptional regulators EVI1 and MDS1‐EVI1.201 This condition may present in the neonatal period with severe macrocytic anaemia and very severe thrombocytopenia (platelets less than 10 × 109/l).201,219 A severe form of inherited thrombocytopenia has recently been shown to be caused by mutations in the GNE gene that encodes an enzyme important in sialic acid biosynthesis (UDP‐N‐acetyl‐glucosamine 2‐epimerase/N‐acetylmannosamine kinase).220,221 While some families also have a congenital myopathy others have isolated thrombocytopenia without any evidence of other associated disease.220,221 Several of the cases have presented in the neonatal period and are notable because of the severity of the thrombocytopenia (platelet count less than 10 × 109/l) and, while bleeding has been reported to be minor,221 at least one neonate had bilateral intraventricular haemorrhages and long‐term neurodevelopmental delay.220 DIAPH1‐related thrombocytopenia is a rare form of autosomal dominant thrombocytopenia in which, like MYH9‐related disease, patients are at risk of developing sensorineural deafness during infancy and some also develop mild transient leucopenia.222 Cases presenting with bleeding or thrombocytopenia in the neonatal period have not yet been reported. Although SRC‐related thrombocytopenia is mainly recognised in adults and children and, importantly, is associated with childhood myelofibrosis,149,223 a recent case of an affected neonate with an autosomal dominant gain‐of‐function mutation in the SRC gene has been reported where the thrombocytopenia was moderate and associated with bilateral cephalohaematomata followed, at the age of 3 months, by a stroke.224 We recommend a pragmatic approach to investigating neonatal thrombocytopenia, based on gestational age and age at presentation, to limit over‐investigation of mild or self‐limiting cases. Figures 4.1 and 4.2 show a practical diagnostic algorithm, which can be applied to the diagnosis and investigation of thrombocytopenia in preterm and term neonates, respectively. The sequence of diagnostic investigations differs in preterm neonates compared with term neonates because of the most frequent causes of neonatal thrombocytopenia are also different in preterm neonates from those in term neonates. For example, isolated thrombocytopenia in a term neonate should immediately raise suspicion of possible FNAIT whereas in preterm neonates FNAIT is usually considered only after other more frequent causes, such as infection, have been excluded (Case 4.3, see page 231). The cause of each episode can usually be determined by a combination of the clinical history, the timing of the onset of thrombocytopenia (Tables 4.1 and 4.2) and the blood count and film. A small proportion of term and preterm neonates (<1%) will have persistent thrombocytopenia and it is these who should have further specialised investigations performed, guided by the presence or absence of dysmorphic features or a family history. The main causes of inherited thrombocytopenia are shown in Tables 4.4–4.6 and next generation sequencing is increasingly used as a more rapid, and ultimately cost effective, way of establishing a precise diagnosis. Overall, most episodes of thrombocytopenia are transient, self‐limiting and relatively mild and therefore unlikely to have short‐term or long‐term consequences. Although receiving a platelet transfusion was reported in earlier studies to increase the risk of death in thrombocytopenic neonates,225 more recent studies show that the relationship between platelet count and risk of bleeding is not straightforward.14,16,36 The most important clinical factors that affect the frequency of major haemorrhage are low birthweight, prematurity, thrombocytopenia developing in the first 10 days of life and a diagnosis of NEC.14 The most common site of major haemorrhage in neonates is intracranial (particularly intraventricular), which is documented in about 5% of all thrombocytopenic neonates, while gastrointestinal haemorrhage occurs in 1–5%, pulmonary haemorrhage in 0.6–5% and haematuria in 1–2% of thrombocytopenic neonates.36 Low birthweight preterm neonates have one of the highest rates of ICH of any age group (up to 25%), and whether the aetiology of the thrombocytopenia is immune or genetic, ICH in the perinatal period is often fatal or leads to long‐term neurodevelopmental disability.5 Platelet transfusions remain the mainstay of treatment of neonatal thrombocytopenia. The current British Committee for Standards in Haematology (BCSH) guidelines for platelet transfusion67 employ different platelet transfusion thresholds for neonates predicted to be at ‘high’ or ‘very high’ risk of haemorrhage. ‘High risk’ neonates are those less than 28 weeks’ gestation, or with a birthweight less than 1000 g, or who are clinically unstable, are due for planned surgery, or have minor bleeding or coagulopathy. In these children, the transfusion threshold of 25 × 109/l is currently recommended. For ‘very high risk’ neonates, those with active major haemorrhage, who are considered to be at a higher risk of ICH and adverse neurodevelopmental outcome, a threshold of 50 × 109/l is recommended (Table 4.7). Although these recommendations remain a useful guide, there have now been a number of carefully conducted studies to evaluate much more precisely the clinical signs and consequences of thrombocytopenia in neonates and to begin to define the most appropriate platelet transfusion thresholds based on more objective measures. As discussed in detail in several recent reviews, thresholds for neonatal platelet transfusion have been controversial for many years and are likely to continue to change as data from large randomised trials become available.16,17,226,227 As an example of the complexity of the relationship between bleeding, platelet count and platelet transfusion in neonates, the recent PlaNeT2 MATISSE Collaborators Trial involving more than 600 preterm neonates randomised to receive platelet transfusion when their platelet count fell below a previously accepted threshold of 50 × 109/l or to be transfused only when their platelets fell below 25 × 109/l, demonstrated that platelet transfusion at the lower threshold of less than 25 × 109/l was beneficial both in reducing bleeding and the risk of death.16 At the same time, perhaps unexpectedly, the same trial showed that transfusing platelets to neonates using the higher threshold (i.e. with platelet counts of 25–49 × 109/l) was associated with an increased risk of bleeding or death. Table 4.7 Suggested thresholds of platelet count for neonatal platelet transfusion Adapted from reference 67. New et al. (2016) Br J Haematol, 175, 784–828. John Wiley & Sons. ICH, intracranial haemorrhage; FNAIT, fetal/neonatal alloimmune thrombocytopenia. The principal causes of abnormal platelet function in the neonatal period are summarised in Table 4.8. The majority of platelet function disorders in neonates are acquired and often secondary to various types of therapy. Their true incidence is unknown as they are rarely systematically sought or investigated. Secondary causes of impaired platelet function include a number of drugs, such as indomethacin, which is widely used to treat patent ductus arteriosus in preterm neonates,228 therapeutic hypothermia, which is used to preserve cerebral function in neonates with perinatal asphyxia,229 and extracorporeal membrane oxygenation (ECMO).230 The inherited platelet function disorders that may present in the neonatal period are rare and many also cause thrombocytopenia, as discussed earlier. Abnormal platelet function, whether acquired or inherited, manifests with bleeding, particularly from mucocutaneous sites including the nose and mouth. More severe blood loss may occur as a result of haematuria or gastrointestinal bleeding. Assessment of platelet function in neonates has been notoriously difficult because of the large volumes of blood required for platelet aggregometry and the practical difficulties in performing and interpreting the bleeding time, especially in preterm neonates. These approaches have largely been replaced by next generation sequencing using gene panels containing known pathogenic variants responsible for inherited platelet function disorders.143,144 Table 4.8 Causes of abnormal neonatal platelet function ECMO, extracorporeal membrane oxygenation. Inherited disorders of platelet function where the platelet count is normal that may present during the neonatal period include Glanzmann thrombasthenia, leucocyte adhesion deficiency type III and RASGRP2‐related disease. In contrast, the majority of inherited platelet function disorders present much later, in adulthood. although occasional cases of bleeding in infancy due to bi‐allelic mutations in GP6, the gene encoding glycoprotein 6, have been reported.231 Glanzmann thrombasthenia is caused by bi‐allelic mutations in the ITGA2B or the ITGB3 gene. The disease often presents with extensive purpura at or shortly after birth.232 Diagnosis is based on a combination of the normal platelet count and morphology despite the bleeding phenotype and platelet flow cytometry showing reduced or absent expression of glycoprotein IIb (CD41) and glycoprotein IIIa (CD61). Note that patients with qualitative defects in glycoproteins IIb/IIIa may have normal platelet glycoprotein flow cytometry profiles. Genetic analysis, preferably using a targeted next generation sequencing panel, should also be performed to confirm the diagnosis.233 Classically, platelets from patients with Glanzmann thrombasthenia fail to aggregate in vitro with all agonists apart from ristocetin but in practice these tests are neither necessary nor feasible in neonates. The main differential diagnoses to consider in neonates with suspected Glanzmann thrombasthenia are leucocyte adhesion deficiency type III and RASGRP2‐related disease, both of which cause similar defects in platelet function and also have normal platelet counts and normal platelet morphology. Leucocyte deficiency type III is due to bi‐allelic mutations in the FERMT3 gene, which causes an activation defect of β‐integrins on both platelets and leucocytes.234 This disorder also usually presents in the neonatal period with bleeding defects very similar to those in Glanzmann thrombasthenia together with persistent neutrophilia, early‐onset bacterial infection and delayed separation of the umbilical cord.233,235 Prompt diagnosis through a combination of platelet flow cytometry and genetic testing is important because the mortality in the first 2 years of life is more than 75% and haemopoietic stem cell transplantation is curative for both the bleeding disorder and the associated immunodeficiency.236 RASGRP2‐related disease is caused by bi‐allelic mutations in the RASGRP2 gene and also typically presents in the neonatal period with mucocutaneous bleeding or bleeding after circumcision. The disease is very rare and in several families has been misdiagnosed as Glanzmann thrombasthenia on the basis of the clinical phenotype; the two diseases are usually distinguishable using platelet flow cytometry, when CD41 and CD61 expression will be normal in RASGRP2‐related disease.237 There is no agreed upper limit of normal for the platelet count in neonates. A large retrospective study of platelet counts retrieved from hospital records in the USA from over 47 000 neonates found that the 95th percentile upper limit was 750 × 109/l.238 On the other hand, there is no evidence that platelet counts above this threshold cause an increase in the risk of thrombosis in neonates. Causes of neonatal thrombocytosis are shown in Table 4.9. The largest systematic study of the causes and implications of extreme thrombocytosis (>1000 × 109/l) in neonates was reported by the same group.239 They found 25 cases in a retrospective review of hospital records from 40 471 neonates (1 in 1600), all of which were judged to be secondary, most often in association with infection and/or surgery, and clinically insignificant. Our experience is similar. In particular, thrombocytosis is more common where there is chronic or prolonged infection, such as osteomyelitis or CMV infection and has also been reported recently after SARS‐CoV‐2 infection.240 Thrombocytosis has also been reported as a complication of subcutaneous fat necrosis of the newborn, a form of panniculitis of unclear aetiology.241 Table 4.9 Causes of neonatal thrombocytosis CMV, cytomegalovirus; SARS‐CoV‐2, severe acute respiratory syndrome coronavirus‐2; TAM, transient abnormal myelopoiesis. Although more often presenting later in infancy, a number of cases of Kawasaki disease have been reported in neonates and a recent review of the literature that included 20 neonatal cases found that two‐thirds of them had thrombocytosis,242 although occasional cases of neonatal thrombocytopenia have also been reported.243 Kawasaki disease characteristically causes acute systemic vasculitis in young children and presents with fever, rash, lymphadenopathy, bilateral conjunctivitis (‘red eyes’) and peripheral oedema.242 The pathogenesis of Kawasaki disease remains poorly understood but it is believed to be triggered by viral or bacterial infection and to result from immune dysregulation, which likely drives both the vasculitis and secondary thrombocytosis in infants with an underlying genetic susceptibility. The condition is self‐limiting and responds rapidly to treatment with IVIg but a high index of suspicion for the diagnosis is crucial because prompt treatment is essential to prevent long‐term cardiac complication.244 Of note, neonates with TAM may also develop thrombocytosis,141 perhaps indicating that the abnormal megakaryoblasts in this condition retain the ability to differentiate into megakaryocytes and platelets. Furthermore, in utero acquisition of the JAK2 V617F variant associated with myeloproliferative neoplasms has been reported in children with essential thrombocythaemia or polycythaemia vera,245 suggesting that neonates with persistent, unexplained thrombocytosis should be further investigated; however, it should be noted that although the mutation was present at the time of birth in the two reported cases, there were no data available on the platelet count in the neonatal period.245 By contrast, familial essential thrombocythaemia due to autosomal dominant mutation in the thrombopoietin gene (THPO) has been shown to present in the neonatal period, as well as in older children.246 The platelet count may be elevated at birth or may rise rapidly over the following weeks.247 In some families the THPO mutation and thrombocytosis are also associated with congenital transverse limb abnormalities, such as malformations of the foot or absent digits.246 Finally, there have been a number of reports of neonatal thrombocytosis secondary to maternal ingestion, during pregnancy, of drugs such as methadone, psychopharmaceutical drugs, zidovudine and thiopurines, such as azathioprine and mercaptopurine.248–251 Neonatal coagulation disorders have been comprehensively detailed in a number of recent reviews226,252,253 and are only briefly described here. It is important to note that although coagulation and thrombotic problems in the neonate are occasionally inherited, much more often they are secondary to intrauterine or neonatal events. In comparison with older infants and children, neonates are more at risk both of haemorrhage and of thrombosis. This partly reflects the physiologically low levels of many of the coagulation factors and of inhibitors of coagulation, particularly in preterm neonates.226,252,254 The changes in synthesis and activity of the principal coagulation proteins during fetal development were described more than 20 years ago.255 Most of the principal proteins can be detected from the 10th week of gestation onwards and gradually rise during fetal life. As the coagulation factors do not cross the placenta, or do so only in small quantities, the fetus is reliant on its own ability to synthesise adequate amounts of these proteins. It is important to note that normal values both indicating the functional activity of the major coagulation pathways (e.g. the prothrombin time) and for the individual factors vary with postnatal age as well as gestational age.256–259 These show that the levels of the vitamin‐K‐dependent factors (factors II, VII, IX and X) and of factors XI and XII are all low compared with adult values and remain so during the first month of life. Importantly, the levels of factors V and XIII and fibrinogen are similar to adult values at birth, even in preterm babies born at 30–37 weeks’ gestation and levels of factor VIII and VWF are normal or increased. Most significant bleeding disorders in neonates can be identified using simple screening tests, the exceptions being factor XIII deficiency and platelet function disorders. Suspicion of a coagulation defect in a neonate requires a prothrombin time (PT), activated partial thromboplastin time (APTT), thrombin time (TT), fibrinogen assay and platelet count, with results being compared with a reference range appropriate for the gestational age.260 It may be useful to test both parents for coagulation abnormalities when an inherited disorder is suspected as there is considerable overlap between coagulation factor levels in inherited or acquired deficiency states and neonatal normal ranges, particularly in preterm neonates. D‐dimer levels are not usually helpful for diagnosis because the normal neonatal values are markedly elevated at birth compared with those in older children and adults and only return to the levels seen in older children by 1 month of age.261 The two most common practical problems in interpreting the results of coagulation screens in the newborn are: the absence of laboratory‐specific reference values that reflect the normal ranges for neonates born at different gestations; the frequency of artefacts caused by partially clotted samples; incorrectly filled coagulation screen bottles with the resultant incorrect concentration of anticoagulant; and samples contaminated with heparin from indwelling intravascular catheters. Causes of acquired coagulopathy in neonates include DIC, vitamin K deficiency, liver disease, metabolic disorders and giant haemangioendotheliomas (Kasabach–Merritt syndrome). Uncommonly, a coagulation abnormality in a neonate is the result of transplacental transfer of a maternal inhibitor, such as anti‐VWF.262 The usual pattern of coagulation abnormalities in neonatal DIC is prolongation of the PT, APTT and TT, together with a low platelet count and fibrinogen.35 The most common causes of DIC in the neonatal period are shown in Table 4.10. Coagulation abnormalities in the first few days of life are most often the result of perinatal hypoxia, for example due to placental abruption, especially where there is HIE. The mechanism in such cases is DIC which is also a feature of NEC, severe sepsis and some types of congenital viral infection, such as that caused by coxsackievirus (see Case 4.2) and chikungunya virus. DIC may also complicate twin pregnancies either through retention of a dead second twin, which can trigger DIC in the surviving twin, or through hyperviscosity in the recipient twin of twin‐to‐twin transfusion. The vast majority of neonates with DIC are clinically very unwell and bleeding is often generalised from multiple sites, including oozing from venepuncture sites. Pulmonary haemorrhage is a major cause of death. Table 4.10 Causes of disseminated intravascular coagulation (DIC) in neonates Vitamin K deficiency remains an important cause of bleeding problems in the newborn (reviewed in references 226 and 263). Levels of vitamin K‐dependent procoagulant factors (factors II, VII, IX and X), protein C and protein S are low at and after birth because of poor placental transfer of vitamin K, low vitamin K stores at birth, low vitamin K in breast milk and the lack of bacterial vitamin K synthesis in the sterile neonatal gut. Classical vitamin K‐dependent bleeding (VKDB), also termed haemorrhagic disease of the newborn, typically presents with ICH or gastrointestinal bleeding 2–7 days after birth and affects babies who have not received prophylactic vitamin K at birth, especially if breastfed or with poor oral intake. The deficiency may be even more severe in neonates of mothers on anticonvulsant therapy. Late VKDB occurs between 2 and 8 weeks after birth and usually presents with ICH in an otherwise well, breastfed term baby or in babies with malabsorption or liver disease. These infants may present with prolonged or excessive bleeding after circumcision as well as ICH or gastrointestinal bleeding. The diagnosis of vitamin K deficiency is usually straightforward. The most useful indicator is the presence of an isolated prolonged PT, which corrects following vitamin K administration. Importantly, the results of these tests need to be interpreted with reference to gestational age control values. In cases where there is any doubt, assays of the inactive form of factor II (undercarboxylated prothrombin; PIVKA‐II [protein induced by vitamin K absence or antagonist]) can be used to confirm the diagnosis. Treatment of VKDB is vitamin K 1 mg intravenously or subcutaneously with fresh frozen plasma (FFP) only in the case of severe haemorrhage. As part of an ongoing WHO project on child health care, existing recommendations for vitamin K prophylaxis in the prevention of haemorrhagic disease of the newborn were recently reviewed.264 In the UK, the National Institute for Health and Care Excellence (NICE) recommends that, for prevention of haemorrhagic disease of the newborn, all full‐term babies should receive 1 mg of intramuscular vitamin K (phytomenadione) prophylactically at or soon after birth. A lower dose is appropriate for babies of less than 36 weeks’ gestation. If the mother declines intramuscular vitamin K, oral vitamin K should be offered, multiple doses then being required (e.g. 2 mg at birth, at 4–7 days and at 1 month). Incidence of deficiency has been estimated at 0.64 per 100 000 live births; around half of cases are attributable to no prophylaxis being given, mainly because parental consent has been withheld.265 The need for administration of vitamin K is greater in breastfed infants because infant formulae are fortified. Parenteral rather than oral vitamin K is particularly important in babies with unrecognised biliary atresia266 or other causes of malabsorption. The most common inherited coagulation disorders presenting in the neonatal period are factor VIII deficiency (haemophilia A), which has a frequency of 1 in 5000 male births, and factor IX deficiency (haemophilia B), which occurs in 1 in 30 000 male births. Other inherited coagulation disorders that can present in the neonate include some types of von Willebrand disease, severe hypofibrinogenaemia, factor XIII deficiency and combined factor V and factor VIII deficiency. The most serious complication of these disorders is ICH (reviewed in reference 267). Other clinical presentations in the neonatal period include: cephalohaematoma; bleeding at the sites of venepuncture, heel‐prick or intramuscular injections; gastrointestinal haemorrhage and bleeding following circumcision (reviewed in references 268 and 269). Up to half of babies with haemophilia A present in the first month of life.268 The presentation of haemophilia B in the neonate is similar to that of haemophilia A. If haemophilia A or B is suspected or has already been confirmed by antenatal diagnosis, close collaboration between obstetricians, neonatologists and haematologists to manage both the pregnancy and delivery is essential. A number of consensus national and international guidelines are available.267,269–272 A peripheral blood or cord blood sample should be obtained for an APTT and urgent factor assay and intramuscular vitamin K should be withheld until the results of the assay are available.65 Treatment should be with the relevant recombinant factor. The two types of von Willebrand disease (VWD) that may present in the neonatal period are type 2B VWD and type 3 VWD. Type 3 VWD presents with similar clinical findings to haemophilia, as levels of both VWF and factor VIII are low. In contrast to haemophilia A and B, type 2B VWD is autosomal recessive and may therefore cause initial diagnostic uncertainty in male neonates. Presentation may be with cephalohaematoma, cheek haematoma (following suckling), post‐venepuncture bleeding, conjunctival haemorrhage and bleeding from the umbilical stump or following circumcision.273 The diagnosis is made by measuring VWF, factor VIII and the pattern of VWF multimers. At present, type 3 VWD is treated with a high purity VWF:factor VIII concentrate (such as Wilate). Type 2B disease is rare and typically presents with thrombocytopenia. Factor XIII deficiency and severe hypofibrinogenaemia show a particular association with umbilical cord bleeding and delayed separation of the cord. Importantly, factor XIII deficiency is an important cause of perinatal ICH, a recent report noting that almost half of the 27 neonates in a retrospective study had CNS bleeding.274 In addition, as for many severe inherited coagulation and platelet disorders, factor XIII deficiency may present with bleeding following circumcision.275 The inheritance is autosomal recessive. The diagnosis of factor XIII deficiency is made using a screening test based on measuring clot solubility in 5 mol/l urea solution followed by assay of factor XIII levels; routine coagulation tests (APTT, PT and TT) are normal. The diagnosis can be confirmed using next generation sequencing to detect mutation of F13A1 or F13B, the genes encoding the subunits of factor XIII. Bleeding is treated with factor XIII concentrate. A number of other rare deficiencies may also present with umbilical cord bleeding and/or delayed separation of the cord, including factor V deficiency, combined factor V and factor VIII deficiency, factor X deficiency and inherited deficiency of all vitamin K‐dependent factors.276 Neonates have an increased risk of thrombosis compared with older infants and children. This is mainly due to the physiologically low levels of many of the inhibitors of coagulation and the use of indwelling vascular catheters. Concentrations of antithrombin, heparin cofactor II and protein C are decreased at birth compared with those in older children and adults; protein S levels are also low due to the virtual absence of its binding protein (C4b‐BP), but overall protein S activity is normal as it exists mainly in its free active form. Plasminogen levels at birth are only 50% of adult values so neonates have a reduced ability to generate plasmin in response to fibrinolytic agents. Neonatal thrombotic disorders have been comprehensively detailed in a number of recent reviews277–282 and are only briefly described here. The International Pediatric Thrombosis Network is an additional source of useful and up‐to‐date information: https://www.isth.org/iptn. The only inherited deficiencies for which there is a proven role in neonatal thrombosis are deficiencies in protein C, protein S and antithrombin. Homozygous protein C deficiency and homozygous protein S deficiency can both cause purpura fulminans. Homozygous antithrombin deficiency usually presents with thrombosis much later in childhood but neonatal thrombosis has been reported.282 Specific mutations in the factor V gene (factor V Leiden) and the prothrombin gene (F2 G20210A) are known prothrombotic risk factors in adults but have not yet been reported to cause neonatal thrombotic problems in isolation. Guidelines from the Haemostasis and Thrombosis Task Force of the BCSH state that congenital thrombophilia should be considered and screened for where there is a family history of neonatal purpura fulminans or in any child with clinically significant thrombosis, including spontaneous thrombotic events, unanticipated or extensive venous thrombosis, ischaemic skin lesions or purpura fulminans.280 Importantly, thrombosis related to an intravascular catheter has been shown not to predict an underlying inherited thrombophilia and investigation is not currently recommended unless there is a family history or other risk factors suggestive of an underlying congenital deficiency.278 Similarly, although controversial for many years, well‐designed case‐control studies have clearly shown that thrombophilia is not associated with a risk of perinatal stroke.283,284 The screening tests that should be performed in all suspected cases of neonatal thrombophilia are assays of protein C, protein S and antithrombin.270,285 In addition, babies with thrombosis born to mothers with SLE and/or antiphospholipid syndrome should be tested for lupus anticoagulant, as antiphospholipid antibodies may cross the placenta and are a rare cause of neonatal thrombosis in such babies. The value of molecular analysis to identify the factor V Leiden and the prothrombin F2 G20210A mutation in neonates is still unclear and such testing is not routinely recommended.7 The relevance of serum lipoprotein a and of the MTHFR (methylenetetrahydrofolate reductase) genotype to neonatal management is unclear at present. The most common risk factors for neonatal thrombosis are the presence of an intravascular catheter and shock secondary to sepsis, hypoxaemia or hypovolaemia.281,286 Catheter‐related thrombosis causes more than 80% of venous thromboses and more than 90% of arterial thromboses in neonates.278 Renal vein thrombosis, which may be bilateral, is the most common thrombotic event unrelated to the presence of a catheter, with presentation being with haematuria, proteinuria and a loin mass;287 renal atrophy, hypertension and impaired renal function are frequent consequences.287,288 Other sites of thrombosis include the aorta or aortic arch, cerebral vessels and intracardiac; the latter may be complicated by venous obstruction, pulmonary embolism or peripheral embolism. The diagnosis is made by Doppler ultrasound or contrast angiography. Treatment of catheter‐related thrombosis depends on the severity and extent of thrombosis and is based on prompt catheter removal as the first step.289 If signs progress despite removal, unfractionated heparin, low molecular weight heparin or thrombolytic therapy with urokinase or tissue plasminogen activator may be used. It is important to maintain fibrinogen at levels lower than 1 g/l and the platelet count greater than 50 × 109/l in neonates receiving thrombolytic therapy. Excellent guidelines for antithrombotic therapy in neonates have been published by the American Society for Hematology279 and the special considerations needed for preterm neonates in particular are reviewed in reference 285. Most recently, direct inhibitors of activated factor Xa or thrombin, such as rivaroxaban or bivalirudin, have been introduced as alternatives to heparin and thrombolytics, but experience in neonates is extremely limited.285,290 Protein C deficiency occurs in 1 in 160 000–360 000 births. It is caused by bi‐allelic mutations in the PROC gene and usually presents within hours or days of birth with purpura fulminans (defined as haemorrhagic necrosis of the skin resulting from dermal thrombosis), stroke (thrombosis or haemorrhage), ophthalmic damage and hypotension.291,292 There is not only thrombosis but also haemorrhage as a result of DIC and thrombocytopenia may be severe. The skin lesions are caused by rapidly progressive life‐threatening haemorrhagic necrosis due to dermal vessel thrombosis. The diagnosis of protein C deficiency is made from the clinical features and undetectable levels of protein C (<0.01 u/ml), together with heterozygote levels in the parents. Therapy with subcutaneous protein C concentrate (40 u/kg, aiming to maintain a plasma level >0.25 u/ml) is effective but irreversible tissue damage, leading to hemiplegia or blindness, may already have occurred, sometimes in utero.291 Heterozygosity for protein C deficiency can predispose to neonatal venous thrombosis. However protein‐C‐deficient heterozygotes rarely present as neonates and their protein C levels can overlap with the lower limit of normal in the first few months of life, delaying diagnosis until 6 months or later. Homozygosity for protein S deficiency has a similar clinical presentation with purpura fulminans but low or undetectable levels of protein S. Treatment is with FFP (10–20 ml/kg every 12 hours) until the lesions resolve. In the long term, severe deficiency of protein C or S can be treated with oral anticoagulants. Homozygous antithrombin deficiency is extremely rare. It may present with neonatal deep vein thrombosis and inferior vena cava thrombosis but more often manifests later in childhood. Heterozygous antithrombin deficiency may also present with arterial or venous thrombosis in the neonatal period; aortic and middle cerebral artery thrombosis, myocardial infarction, cerebral dural sinus and renal vein thrombosis have all been reported in neonates,282 particularly following instrumental delivery293 although presentation in the second decade of life is much more typical. The importance of restricting transfusions to those neonates most likely to benefit is increasingly recognised. The general principles governing this more cautious approach to transfusion in neonates have been described in detail in several recent reviews and national guidelines as well as in international consensus statements.67,227,294–297 Red cell transfusion approaches that have proved successful include late clamping of the umbilical cord (unless resuscitation is needed) and limiting the volume of blood taken for laboratory tests to a minimum as discussed in Chapter 2.298,299 For platelet transfusion the emphasis has been on rigorously evaluating the relevance of the platelet count to the risk of bleeding.300 Additional points to consider when transfusion of any blood component or blood product to a neonate is planned include the need for extra care with identification of a neonate’s blood sample because of the increased risks of misidentification that arise from name changes in babies and confusion between a maternal specimen and a baby’s specimen. When intrauterine transfusion has previously been employed, blood components for transfusion in a neonate should always be irradiated to avoid the risk of transfusion‐associated graft‐versus‐host disease. Otherwise, routine irradiation of blood products for transfusion to neonates is not necessary. The principles and indications for neonatal red cell transfusion are discussed in Chapter 2 and summarised in Table 2.17. Platelets for use in neonates are leuco‐depleted, are from CMV‐negative donors and are usually obtained by apheresis of selected donors who lack high‐titre anti‐A and anti‐B. Platelet transfusion is required for thrombocytopenic babies with bleeding; a transfusion threshold of <50 × 109/l is suggested.67 The threshold for prophylactic transfusion depends on the indications and detailed guidance is available for asymptomatic babies with severe thrombocytopenia and those requiring lumbar puncture or minor or major surgery.67 Recent data point to the value of a restrictive policy for neonatal platelet transfusion.16 In many countries FFP for neonates and infants is pathogen‐inactivated (solvent detergent or methylene blue), negative for high‐titre anti‐A and anti‐B antibodies and sourced from male donors.67 FFP may be required in the management of DIC and when there is bleeding in neonates with abnormal coagulation tests. Its use may be appropriate in DIC, in the presence of bleeding, when the fibrinogen concentration is less than 1.0 g/l or when the PT or APTT is prolonged to more than 1.5 times the midpoint of an appropriate reference range.21 FFP is required for congenital factor deficiencies and for purpura fulminans resulting from protein S or protein C deficiency when the specific concentrate is not available.226 FFP is often over‐used in NICUs for babies with abnormal laboratory tests but without bleeding or the need for invasive procedures.67,226,301 FFP should not be used for volume replacement. Cryoprecipitate contains fibrinogen, factor VIII, VWF, factor XIII and fibronectin. It is pathogen‐inactivated with methylene blue and in the UK is now from male donors only.67 Cryoprecipitate is required for severe hypofibrinogenaemia and may be required in DIC. A threshold fibrinogen concentration of 1 g/l is advised unless there is rapid consumption of fibrinogen, when a higher threshold may be advisable. Fibrinogen concentrate is licensed in the UK for congenital hypofibrinogenaemia but in the acute situation, in a neonate, cryoprecipitate is more likely to be used. Four‐factor prothrombin complex concentrate (PCC), which contains factors II, IX and X and a variable concentration of factor VII, is now the preferred product, in addition to vitamin K, for patients with bleeding due to vitamin K deficiency. Small retrospective studies and case reports have shown that PCC is effective in neonates302 but there are no data from randomised controlled studies in this population. Similarly, a number of retrospective studies have reported good responses to recombinant factor VIIa (rFVIIa) in preterm and term neonates with intractable bleeding and severe coagulopathy.303,304 However, there are no data available at present to identify the specific indications and safety of rFVIIa in neonates.226 (For abbreviations used in the text of the illustrative cases, see page 233–234.)
4
Disorders of platelets and coagulation, thrombosis and blood transfusion
Thrombocytopenia overview
Causes of neonatal thrombocytopenia: a practical classification based on age at onset
Cause
Condition
Immune
Alloimmune thrombocytopenia
Secondary to maternal platelet autoantibodies (e.g. SLE, ITP)
Congenital infection
Cytomegalovirus
Parvovirus B19
Rubella
Human immunodeficiency virus
Toxoplasma gondii
Malaria
Aneuploidy
Trisomy 21
Trisomy 13
Trisomy 18
Triploidy
Malignancy
Transient abnormal myelopoiesis associated with Down syndrome
Congenital leukaemia
Genetic/inherited
Congenital amegakaryocytic thrombocytopenia
Wiskott–Aldrich syndrome
Fas deficiency
Other
Severe Rh haemolytic disease of the newborn
Most common
Placental insufficiency, e.g. IUGR, secondary to maternal hypertension or maternal diabetes
Perinatal asphyxia
Perinatal bacterial infection, e.g. Escherichia coli, group B Streptococcus, Haemophilus influenzae
Disseminated intravascular coagulation
Alloimmune thrombocytopenia
Autoimmune thrombocytopenia, e.g. maternal ITP/SLE, neonatal lupus erythematosus
Less common or rare
Congenital infection, e.g. CMV, rubella, HIV, enteroviruses, parvovirus B19, dengue, chikungunya virus, toxoplasma, malaria
Aneuploidy, e.g. trisomy 21, trisomy 13, trisomy 18 and TAM in neonates with Down syndrome
Associated with thrombosis, e.g. HIT, TTP (congenital ADAMTS13 deficiency), renal vein thrombosis
Bone marrow replacement, e.g. congenital leukaemia, osteopetrosis, HLH
Kasabach–Merritt syndrome
Metabolic disease, e.g. propionic and methylmalonic acidaemia, Gaucher disease, subcutaneous fat necrosis of the newborn
Inherited, e.g. TAR, CAMT, type 2B VWD (see Table 4.4 and text for a more complete list)
Late‐onset sepsis
Necrotising enterocolitis
Congenital infection, e.g. CMV, rubella, HIV, enteroviruses, parvovirus B19, dengue, chikungunya virus, toxoplasma, malaria
Immune: maternal ITP/SLE, neonatal Kawasaki disease*
Kasabach–Merritt syndrome
Metabolic disease, e.g. propionic and methylmalonic acidaemia
Drug‐induced
Inherited, e.g. TAR, CAMT (see Table 4.4 and text for a more complete list)
Fetal thrombocytopenia
Early‐onset neonatal thrombocytopenia (presenting at <72 hours of age)
Neonatal thrombocytopenia associated with intrauterine growth restriction and other placental insufficiency syndromes
Causes of severe early‐onset thrombocytopenia
Late‐onset neonatal thrombocytopenia (presenting at >72 hours of age)
Conditions leading to clinically significant thrombocytopenia in the neonate
Fetal/neonatal alloimmune thrombocytopenia
Management of fetal/neonatal alloimmune thrombocytopenia
Antenatal management of fetal/neonatal alloimmune thrombocytopenia
Neonatal thrombocytopenia due to maternal autoimmune disease
Thrombocytopenia due to congenital infections
Congenital cytomegalovirus infection
Other congenital viral infections
Congenital parasitic infections
Other congenital infections associated with neonatal thrombocytopenia: tuberculosis and syphilis
Neonatal thrombocytopenia associated with chromosomal abnormalities
Inherited thrombocytopenia
Disease
Gene
Comments
Bernard–Soulier syndrome
GP1BA*
GP1BB*
GP9
Autosomal recessive (* rare autosomal dominant forms reported)
Macrothrombocytopenia
Impaired platelet function
FLI1‐related thrombocytopenia
FLI1
Autosomal recessive, autosomal dominant or contiguous gene syndrome, Macrothrombocytopenia, giant α granules
Impaired platelet function
FYB‐related thrombocytopenia
FYB1
Autosomal recessive, small platelets
Grey platelet syndrome
NBEAL2
Autosomal recessive Macrothrombocytopenia
Impaired platelet function
ITGA2B/ITGB3‐related thrombocytopenia (Glanzmann thrombasthenia variant)
ITGA2B/ITGB3
Autosomal dominant and recessive forms
Macrothrombocytopenia
Impaired platelet function
PRKACG‐related thrombocytopenia
PRKACG
Autosomal recessive, macrothrombocytopenia
Impaired platelet function
Thrombocytopenia presenting from infancy
Mild to moderate bleeding/bruising
GFI1B‐related
GFI1B
Autosomal dominant or recessive
PTPRJ‐related
PTPRJ
Autosomal recessive
SLFN14‐related
SLFN14
Autosomal dominant
Mild thrombocytopenias
All autosomal dominantAll autosomal dominant
No neonatal problems reported
ACTN1‐related
ACTN1
CYCS‐related
CYCS
IKZF5‐related
IKZF5
TRPM7‐related
TRPM7
TPM4‐related
TRM4
THPO‐related
THPO
TUBB1‐related
TUBB1
Non‐syndromic inherited thrombocytopenia presenting as isolated thrombocytopenia
Disease
Gene
Comment
Chédiak–Higashi syndrome
LYST
Autosomal recessive
Partial oculocutaneous albinism, predisposition to pyogenic infections, abnormal large granules in many cells, impaired platelet function; usually presents in infancy but bleeding in neonates has been reported
FLNA‐associated thrombocytopenia
FLNA
X‐linked, neonatal lung disease
Otopalatodigital spectrum disorders
Brain periventricular nodular heterotopia
Thrombocytopenia may develop later
Jacobsen syndrome/Paris–Trousseau thrombocytopenia
Deletions at 11q23
Autosomal dominant
IUGR, developmental delay, craniofacial abnormalities, malformations of the heart, gastrointestinal tract, urinary tract, CNS and/or skeleton; immunodeficiency
Impaired platelet function
MYH9‐related thrombocytopenia
MYH9
Autosomal dominant, Macrothrombocytopenia
Most patients develop sensorineural deafness (60%), nephropathy (30%) and/or cataracts (20%)
Neutrophils show Döhle body‐like inclusions
Stormorken syndrome/York platelet syndrome
STIM1
Autosomal dominant, tubular aggregate myopathy
Impaired platelet function
Mild anaemia, asplenia, miosis, ichthyosis
Thrombocytopenia absent radii syndrome
RBM8A
Autosomal recessive, bilateral radial aplasia
Renal, cardiac ± CNS malformations
Cow’s milk intolerance
Platelet count may rise over time
Wiskott–Aldrich syndrome and X‐linked thrombocytopenia
WAS
X‐linked, immunodeficiency
Small or normal sized platelets
X‐linked thrombocytopenia with GATA1 mutation
GATA1
X‐linked, macrothrombocytopenia
Anaemia – dyserythropoietic or thalassaemic
Bernard–Soulier syndrome
Disease
Gene
Comment
Congenital amegakaryocytic thrombocytopenia
MPL
THPO (rarely)
Autosomal recessive
All patients develop progressive bone marrow failure (aplastic anaemia)
Familial platelet disorder with predisposition to AML
RUNX1
Autosomal dominant
Normal sized platelets
>40% develop AML, 25% develop ALL
ANKRD26‐related thrombocytopenia
ANKRD26
Autosomal dominant
Normal sized platelets
~10% develop myeloid malignancies
ETV6‐related thrombocytopenia
ETV6
Autosomal dominant
Normal sized platelets
~30% develop myeloid malignancies, especially ALL
Radio‐ulnar synostosis with amegakaryocytic thrombocytopenia
HOXA11
Autosomal dominant
Possible evolution to aplastic anaemia
MECOM‐associated syndrome
MECOM
Autosomal dominant
Almost all patients develop bone marrow failure (aplastic anaemia)
Inherited bone marrow failure syndromes (trilineage)
Fanconi anaemia
22 genes
Autosomal recessive, occasionally X‐linked
Dyskeratosis congenita
16 genes
X‐linked, autosomal recessive or autosomal dominant
Other causes of isolated inherited thrombocytopenia presenting in the neonatal period
Syndromic inherited thrombocytopenias that may present in the neonatal period
Chédiak–Higashi syndrome
FLNA ‐associated thrombocytopenia
Jacobsen syndrome/Paris–Trousseau thrombocytopenia
MYH9 ‐related thrombocytopenia
Stormorken syndrome and York platelet syndrome
Thrombocytopenia with absent radii syndrome
Wiskott–Aldrich syndrome and X‐linked thrombocytopenia
X‐linked thrombocytopenia due to GATA1 mutation
Other rare syndromic inherited platelet disorders
Inherited thrombocytopenias associated with predisposition to leukaemia and bone marrow failure
Congenital amegakaryocytic thrombocytopenia
Inherited thrombocytopenias with increased susceptibility to leukaemia (FPD/AML, ANKRD26 ‐related thrombocytopenia and ETV6 ‐related thrombocytopenia)
Radio‐ulnar synostosis: amegakaryocytic thrombocytopenia and MECOM syndrome
Other rare inherited thrombocytopenias presenting in the neonatal period
Investigation of neonatal thrombocytopenia
Management of neonatal thrombocytopenia
Indications for platelet transfusion
Platelet count (×10 9 /l)
Indication for platelet transfusion
<25
Neonates with no bleeding (including neonates with FNAIT if no bleeding and no history of ICH in a sibling)
<50
Neonates with bleeding, current coagulopathy, before surgery, or infants with FNAIT if previously affected sibling with ICH
<100
Neonates with major bleeding or requiring major surgery (e.g. neurosurgery)
Platelet function disorders
Disease
Specific causes
Acquired
Drugs
e.g. indomethacin, prostacyclin, sodium valproate
Therapeutic procedures
e.g. ECMO, therapeutic hypothermia (for perinatal asphyxia)
Uraemia
Inherited
Glanzmann thrombasthenia
Bi‐allelic ITGA2B/ITGB3 mutations
Leucocyte adhesion deficiency type III
Bi‐allelic FERMT3 mutations
RASGRP2‐related disease
Bi‐allelic RASGRP2 mutations
Inherited platelet function disorders with normal platelet counts presenting in the neonate
Thrombocytosis
Conditions
Specific disorders
Acute inflammatory conditions
Kawasaki disease
Subcutaneous fat necrosis
Infections
SARS‐CoV‐2
CMV
Myeloproliferative disorders
TAM
Familial myeloproliferative neoplasms, e.g essential thrombocythaemia due to THPO or MPL mutation
Chronic inflammation
Following surgery
Chronic infection, e.g. osteomyelitis
In utero exposure to drugs
Methadone
Psychopharmaceutical drugs
Thiopurines, e.g. azathioprine, mercaptopurine
Zidovudine
Abnormalities of coagulation
Developmental haemostasis
Laboratory investigation of coagulation disorders in the neonate
Acquired coagulation abnormalities
Disseminated intravascular coagulation
Cause
Condition
Bacterial sepsis/necrotising enterocolitis
Group B Streptococcus
Gram‐negative infections
Necrotising enterocolitis
Congenital infection
Enteroviruses, e.g. coxsackievirus, cytomegalovirus
Perinatal events
Hypoxic ischaemic encephalopathy
Severe meconium aspiration
Death of a twin in utero
Twin‐to‐twin transfusion
Severe fetomaternal haemorrhage/placental abruption
Hypothermia
Vitamin K deficiency
Inherited coagulation disorders
Thrombosis
Screening tests for thrombophilia in neonates
Acquired thrombotic abnormalities
Inherited thrombotic abnormalities
General principles of neonatal platelet and plasma component transfusion
Platelet transfusion
Fresh frozen plasma
Cryoprecipitate
Prothrombin complex and recombinant factor VIIa
Illustrative cases
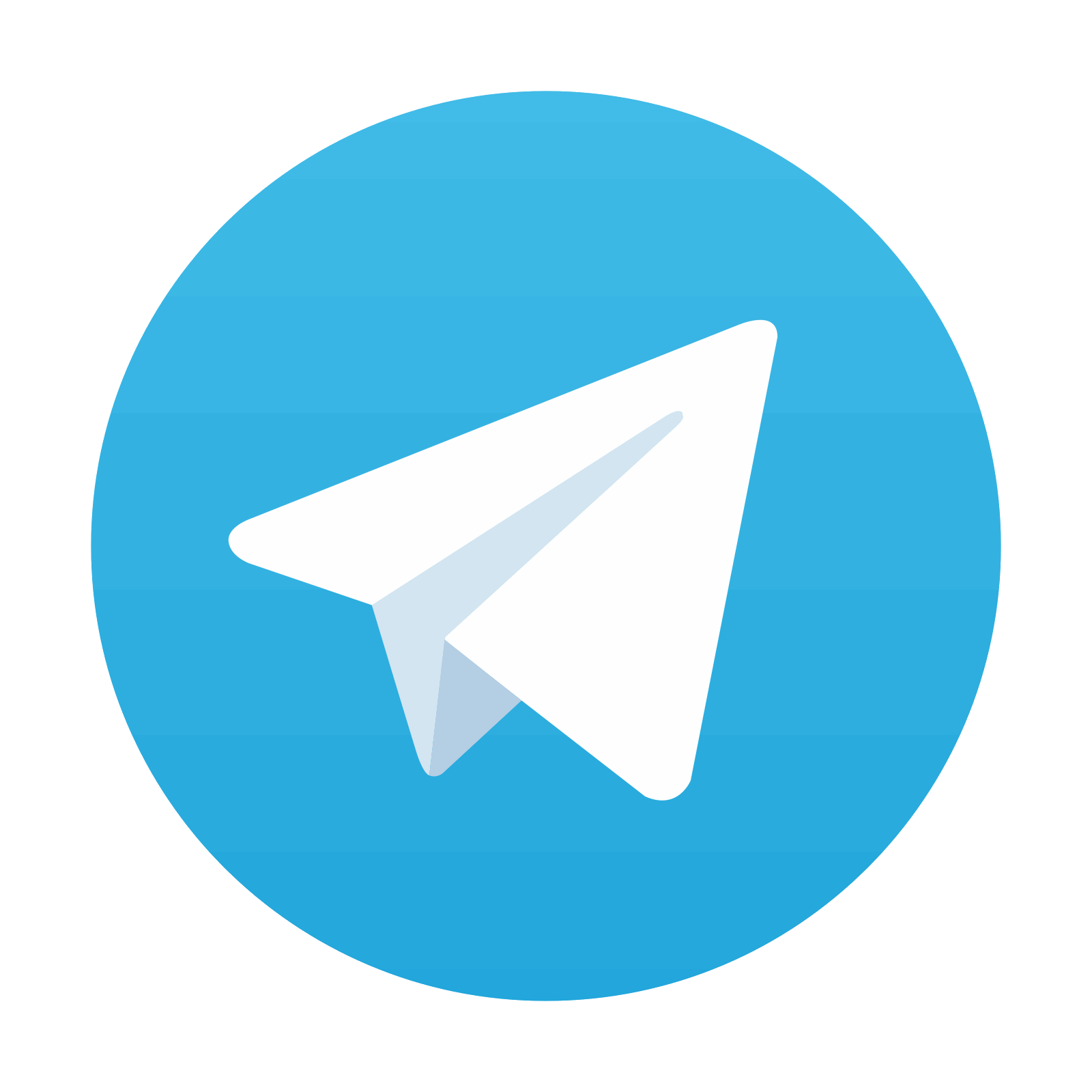
Stay updated, free articles. Join our Telegram channel

Full access? Get Clinical Tree
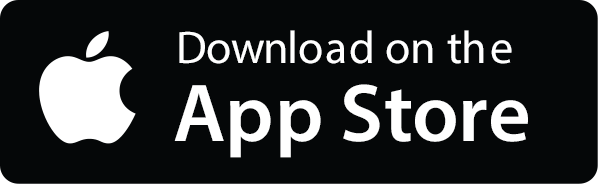
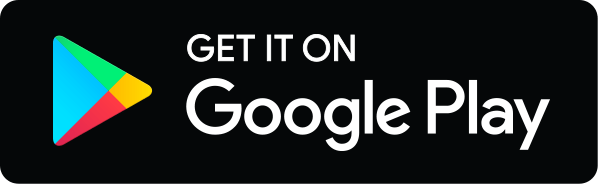