Carbohydrate Counting |
Equivalent 15 g carbohydrate servings Starches: 1 slice of bread, 1/3 cup cooked pasta, ¾ cup dry cereal, or 4–6 crackers Fruit: 1 small piece of fruit or ½ cup of fruit juice Milk: 1 cup of nonfat (skim) milk or ¾ cup yoghurt Desserts: 2 small cookies or ½ cup ice cream | |
Counting the Carbohydrates: Amount of Carbohydrate | Carbohydrate Serving Count |
0–5 g | 0 |
5–10 g | ½ serving |
11–20 g | 1 serving |
21–25 g | 1.5 servings |
26–35 g | 2 servings |
(Data from Kulkarni KD. Carbohydrate counting: a practical meal-planning option for people with diabetes. Clinical Diabetes. 2005;23(3):120–122.)
f. Choose dietary carbohydrates wisely. The management of dietary carbohydrate intake in diabetes had long focused on restricting the intake of simple sugars, primarily the disaccharides such as sucrose, but we now realize that this focus was misplaced. Foods containing simple sugars can generally be accommodated in a diabetic meal plan if adequately covered with appropriate glucose-lowering medications (38). At the same time, in some diabetics, restriction of the intake of simple sugars can be a helpful adjunct to dietary treatment and modest intakes must be maintained in order to avoid displacement of nutrient-dense foods in the diet. Thus, many experts recommend that sucrose and fructose be consumed only in moderation and the ADA recommends limitation of fructose intake (now largely consumed as high-fructose corn syrup in drinks) to less than 12% energy intake to avoid hypertriglyceridemia. The ADA also recommends limitation of sugar-sweetened beverages for this reason and to reduce the risk of weight gain because of the risk of excessive calorie intake. This principle, however, applies to excessive intake of the other macronutrients, protein, or fat as well.
Lately, all expert bodies have appreciated that the correct focus of dietary carbohydrate intake should be on the types of carbohydrates consumed. As discussed in the Dietary Guidelines for Americans (Chapter 1), the most healthful dietary carbohydrates are found in fruits, vegetables, whole grains, and legumes, and these should be the basis of carbohydrate intake in the diabetic diet. Additionally, although the evidence remains inconclusive as far as glycemic control and long-term outcomes are concerned, the consensus recommendation is that diabetics consume at least the dietary reference intake for fiber of 14 g per 1,000 kcal of dietary energy consumption, the same value recommended for the nondiabetic general population.
Despite a very large number of studies and despite some literature support (72–74) for the use of the GI and glycemic load in the management of diabetes, debate remains about whether there is a therapeutic advantage to using the GI of foods as an adjunct to improving postprandial hyperglycemia in diabetics. Thus, the ADA has concluded that there is only Level B evidence for “substituting low-glycemic load foods for high-glycemic load foods” in the management of diabetes (59, Table 13-1). In this context, it is important to remember that the mixed diet consumed by most Americans has a “medium” GI, so that studies comparing low GI foods with high GI foods may overestimate the actual effects that will be found in practice (see also the discussion of GI in Chapter 12, in “Low-Available Carbohydrate Diet”).
g. In addition to diet and lifestyle interventions, employ appropriate drug therapy to ensure optimal control of hyperglycemia. The ADA, the American Association of Clinical Endocrinologists, and the International Diabetes Federation Guideline Development Group each has recently issued consensus guidelines for the successful pharmacologic management of glycemic control in persons with type 2 diabetes (58,60,61).
Strategy of Diet Therapy for Type 1 Diabetics
In persons who do not have diabetes, pancreatic insulin secretion changes abruptly and appropriately in response to a change in blood glucose. After a meal, insulin secretion increases as blood glucose starts to rise. Nondiabetics can vary their caloric intakes severalfold from day to day without becoming hyperglycemic or hypoglycemic because their insulin secretion is exquisitely responsive to changes in blood glucose and the blood glucose level is, thus, tightly regulated. On the other hand, individuals with type 1 diabetes are essentially completely insulin-deficient within, at most, several years, after their initial diagnosis. Thus, type 1 diabetics are unable to secrete insulin in response to a rise in blood glucose. In an attempt to anticipate and regulate glycemia after meals and during the course of normal daily activity, most type 1 diabetics inject, more than once daily, a combination of insulins with different time courses of action. The amount and proportions of the insulin dose are determined by preceding glycemia and anticipated glycemic response. Serum insulin levels are determined largely by the types of insulin injected and by the size and timing of the injected insulin dose. Herbst and Hirsch (75) have published a succinct, informative summary of insulin strategies used in caring for people with diabetes.
None of the currently available insulins, not even those called rapid-acting insulin, can provide a serum insulin profile to precisely match the immediate release of insulin that occurs in nondiabetic subjects following the ingestion of food and the minute-by-minute responsiveness of pancreatic insulin secretion that takes place in nondiabetic subjects. Additionally, no single insulin can match both the initial “first phase,” virtually instantaneous pancreatic insulin release and the more-prolonged, and “second phase,” sustained insulin release that follows carbohydrate ingestion. Thus, the timing of a meal following an injection of insulin is critical. Depending on the type of insulin used, meal ingestion may follow immediately, or almost so (as with the use of rapid-acting insulin), or delayed for 30 to 60 minutes (as with the use of short-acting insulin) in order to try to match the appearance of insulin into the systemic circulation with the absorption of glucose from the meal. Furthermore, it is imperative that the composition and distribution of meals be consistent with the composition and expected actions of the insulin injected. Because most patients with type 1 diabetes inject insulin on a relatively consistent schedule, they also consume meals on a relatively consistent schedule. Insulin doses should be adapted to the patient’s lifestyle, schedule, eating preferences, and level of activity. Tailored regimens of this type are the rule for patients who monitor their blood glucose adequately. However, adjustment of the insulin dose is effective only if the caloric content is regular and the temporal distribution of food is consistent with the insulin regimen employed.
One should aim to achieve consistency in the composition of meals. Some regularity in the proportion of carbohydrates, proteins, and fats is necessary in each meal. The amount of insulin needed to “cover” a meal can be determined empirically, whatever the composition of the meal, and will remain fairly constant if the total number of ingested calories, the carbohydrate content, and the relative mix of the other macronutrients remain reasonably constant. The purpose of the exchange list and/or carbohydrate counting systems is to provide a practical degree of consistency yet allow flexible food choices and, based on the amount of dietary carbohydrate in a meal or snack, the amount and type(s) of injected insulin necessary to “cover” the postprandial glycemic response is estimated. This “insulin to carbohydrate ratio” is largely determined empirically and can vary widely based on the subject’s sensitivity to insulin (and variables like body weight, physical activity, pregnancy, etc., known to alter insulin sensitivity), as well as on the presence (type 2 diabetes) or absence (type 1 diabetes) of the patient’s own pancreatic insulin secretion. Scheiner (76) has written a very brief, entry-level primer describing the practical aspects of implementing insulin-to-carbohydrate ratios in diabetes care. However, there is no single algorithm that will accurately predict, a priori, the insulin to carbohydrate ratio for any specific subject. “First guess” estimates are in the range of about 1 unit of insulin to cover about 10 to 15 g of carbohydrate for adults. Children, particularly very young children, are likely to require much less insulin per gram of carbohydrate than adults. For children less than about 7 years of age, 1 unit of insulin may cover 30 g of carbohydrate and preadolescent children older than about seven may cover 20 g of carbohydrate with 1 unit of insulin.
a. The degree of flexibility in the diet that is consistent with good metabolic control varies from patient to patient. Some patients can vary their caloric intake and dietary composition from day to day and still tightly regulate their blood glucose level by paying careful attention to blood glucose monitoring and insulin dose adjustment. Others require a fixed regimen to control hyperglycemia. Whatever the case, the evidence basis for concluding that there is an unequivocal reduction in diabetic complications following prolonged “normalization” of blood glucose is now extensive. Good control should not be sacrificed for a more liberal diet.
b. The adverse consequences of rigid glycemic control include the risk for obesity because of diminished urinary loss of glucose calories and the antilipolytic effects of insulin. More importantly, however, tight control of blood glucose is associated with a large increased risk of significant, symptomatic hypoglycemia, an unwanted side effect that has now been reported consistently in studies that have achieved near normal blood glucose control. As a rule, the tighter the blood glucose control, the more one will experience clinically significant hypoglycemic episodes. Because we are unable to match plasma insulin and glucose profiles accurately, consistently, and precisely, diabetics who aim for normoglycemia are prone to episodes of serious hypoglycemia requiring the assistance of another person for resolution. Such episodes can lead to permanent detrimental consequences, both to the diabetic patient and others (e.g., auto accidents). Careful and regular monitoring for adverse effects is required, and the insulin dose and calorie intake must be adjusted as necessary. The clinical risk of hypoglycemia is increased when insulin doses are too large or not timed appropriately, when meals or snacks are missed, when physical activity is increased, and when insulin clearance is decreased as renal function declines. Cryer has recently summarized in great detail the evidence for balancing “glycemic goals in diabetes” as a “trade-off between glycemic control and iatrogenic hypoglycemia” (77). This assessment, which includes an exposition of the pathophysiology of hypoglycemia in diabetes, a summary of the evidence base for hypoglycemic risks, and a discussion of therapeutic goals that help minimize risk while preserving the benefit of diabetes control, is essential reading for all those responsible for managing individuals with diabetes (77).
The Dietary Prescription
It is important to individualize the diet prescription to accommodate the diabetic patient’s lifestyle, eating habits, age, and concurrent disease. It is counterproductive to expect the opposite, that is that a patient will change their fundamental habits just to accommodate the diet prescription. The following list can be used to establish such a prescription (78):
1. In order of priority, what are the main general purposes (not strategies or methods) of this patient’s prescription?
2. How much does the patient weigh? How much do the doctor, dietitian, and patient think the patient should weigh? How much would the patient like to weigh?
3. What is the appropriate level of caloric consumption for the patient?
4. Does the patient require insulin? If so, is the blood glucose level relatively stable, moderately labile, or severely labile? What kind of insulin is to be given? At what time? In what amount?
5. What, when, and how much would the patient like to eat if he or she did not have diabetes? Are there any special considerations relating to economic factors or to family or cultural dietary propensities?
6. Is the level of carbohydrate to be limited? To what level or range? To what extent and under what conditions, if any, are concentrated carbohydrates to be used?
7. Are there any special requirements concerning levels of protein?
8. Are there any specific or general requirements with respect to levels of dietary fat, either saturated or unsaturated?
9. How much alcohol is to be permitted? Under what conditions? Should alcohol be exchanged for food? If so, what kind and in what amount?
10. If the temporal distribution of food is of any importance, are there specific requirements concerning the following?
a. The relative size and timing of each of the three main meals
b. The timing, size, and characteristics of any extra feedings
11. To what degree is day-to-day consistency required in
a. Total kilocalories?
b. Size and characteristics of specific feedings, such as lunch?
12. Are dietary adjustments to be made for exercise or marked glycosuria? Of what nature?
13. Are there any special conditions unrelated to diabetes that require a special diet (e.g., gout, hyperlipidemia, renal or cardiac failure)?
14. Can all elements of the prescription be reconciled, and how should this be done? (For example, it is usually not feasible to construct a palatable diet for a lean diabetic if the prescription restricts both carbohydrate and fat.)
15. What kind and degree of changes are to be made subsequently by the dietitian without consulting the physician?
16. What should the patient do if it becomes necessary to postpone or modify a meal (e.g., when attending a dinner meeting or social affair)?
17. Tactical questions:
a. How much precision is required in the various elements of this prescription?
b. What foods can be freely allowed?
c. What foods, if any, are to be weighed or measured?
d. Are any modifications of the standard exchange system appropriate, such as simplification?
e. In general, is food to be unmeasured, estimated, measured, or weighed?
f. Is it necessary or desirable to instruct the patient about the carbohydrate, protein, and fat contents of the common foods?
g. Under what circumstances are artificial sweeteners and diet drinks to be used?
18. Has the patient’s understanding of dietary principles and methods been systematically evaluated?
Setting Up the Diabetic Diet
The first step in formulating the diabetic diet is to calculate the number of calories the patient requires to achieve or maintain a healthy body weight. This is no easy task because no simple approach to the accurate and precise estimation of a specific patient’s energy requirements in a clinical setting is available. Caloric requirements are related to the patient’s age, level of activity, and body weight. Basal metabolic rates (BMRs) are in the range of 21 to 29 kcal per kg daily for adults below the age of 60, with women, on average, having BMRs slightly lower than those for men. Above the age of 60, the daily BMR is in the range of 19 to 23 kcal per kg. It is most appropriate to start at the low end of these ranges to estimate energy expenditure (see also Chapter 5 for a more detailed discussion of energy expenditure).
A lean individual’s estimated energy requirement (EER) in kilocalories per day can be estimated from his or her age, body weight in kilograms, and height in meters according to the following equations (79,80):
Men:
EER = 662 − (9.53 × age) + PA × [(15.91 × wt) + (539.6 × ht)]
Women:
EER = 354 − (6.91 × age) + PA × [9.36 × wt) + (726 × ht)]
PA is the subject’s physical activity quotient. The value of PA assigned to the activities of usual daily living is 1.0. PA increases to 1.11 to 1.12 (for women and men, respectively) for the activities of daily life plus an additional 30 to 60 minutes of moderate activity daily, to 1.25 to 1.27 for at least an additional 60 minutes of moderate daily activity, and to 1.45 to 48 for the activities of daily life plus an additional 180 minutes of moderate activity or at least an additional 60 minutes of moderate daily activity plus an additional 60 minutes of vigorous activity (15,16).
For overweight and obese men and women, such as most type 2 diabetics, total daily energy expenditure for weight maintenance is better estimated using the following modifications of the above equations (79).
Men:
EER = 1086 − (10.1 × age) + PA × [(13.7 × wt) + (416 × ht)]
Women:
EER = 448 − (7.95 × age) + PA × [11.4 × wt) + (619 × ht)]
Sedentary men and women are assigned a PA of 1.0. Low active men and women are assigned the PA values of 1.12 for men and 1.16 for women. Active men and women are given PA values of 1.29 and 1.27, respectively; and very active men and women have PA values of 1.59 and 1.44, respectively. For most obese, type 2 diabetics, it is best to estimate activity level at the sedentary or low levels.
The next step in defining a diabetic diet is to determine the relative macronutrient contents. Various consensus guidelines today (56,59–61) are considerably less prescriptive than those in the past because of the realization that the evidence basis for former limits is not supported by more recent research. Thus, recommendations for the macronutrient contents of diets have evolved to lie within the bounds of healthful diets recommended for otherwise healthy adults as described in the Dietary Guidelines for Americans and its variants published by other expert bodies and discussed in detail in Chapters 1 to 3 as well as other individual sections of this book, including earlier in this chapter. Recommendations for the amounts and types of dietary carbohydrates, proteins, and fats consumed by diabetics are essentially no different from those for the general population. Likewise, as discussed earlier, carbohydrate choices made on the basis of GI or glycemic load have not been shown consistently and convincingly to improve the glycemic control and/or clinical outcomes of diabetics. Moreover, there is no simple algorithm that can predict the GI of individual foods consumed in a mixed diet. In general, the GIs of complex carbohydrates, especially in fiber-rich foods, are low. Soybeans and peanuts have very low GIs, but the GIs of lentils, chick peas, green peas, kidney beans, and pinto beans are two- to fourfold higher. The GI of fructose is equivalent to that of soybeans, the GI of honey is nearly equivalent to that of glucose, and the GI of table sugar (sucrose) is less than that of a baked potato. The blood glucose response after ingestion of a baked potato is essentially the same as that after oral glucose, but rice and pasta evoke a much lower blood glucose response. The reason for the differences in the glycemic response is not completely understood. The GI is influenced by the rate at which foods are digested and absorbed and the degree to which they raise the blood glucose level. The rate of gastric emptying and the presence of fat and protein affect the glycemic response. The glycemic response in patients with type 2 diabetes is similar to that seen in normal persons, but the glycemic response in patients with type 1 diabetes is more variable. These individual variations limit the usefulness of the GI as a teaching tool for patients with diabetes. Also, in the context of mixed meals, the GI tends to lose its practical usefulness because the distinctions between individual foods are blurred. For these reasons, many experts do not consider the GI to be a valuable adjunct to diabetes management but prefer to simply regulate total carbohydrate intake using the carbohydrate counting method discussed earlier. Finally, and perhaps most importantly, the extra “work” of incorporating the GI into the overall diabetic diet prescription may neither necessary nor dramatically effective because all healthful diets such as the DASH diet, the Mediterranean diet, and the diet recommended in the Dietary Guidelines for Americans are fundamentally low GI diets, if implemented to their full extent.
One must next divide the daily diet prescription into meals. The distribution of calories during the day must be adjusted to the patient’s lifestyle and insulin program. A typical diet provides 20% to 30% of calories at breakfast, 20% to 35% at lunch, 25% to 40% at supper, and none to 15% as snacks. Snacks are usually taken at mid-morning, at mid-afternoon, and near bedtime. Tight control of blood glucose is easier if snacks are part of the diet regimen. Mid-morning and mid-afternoon snacks may prevent hypoglycemia by providing calories at times when levels of regular and intermediate-acting insulin reach their peak. Similarly, a bedtime snack may prevent nighttime hypoglycemia. Snacks also reduce the number of calories that are taken at meals and thus help prevent episodes of postprandial hyperglycemia.
After estimating the patient’s energy requirement and determining the meal distribution, a specific meal plan is formulated using the exchange list or carbohydrate counting algorithms. As detailed earlier, the purpose of both systems is to allow a patient to vary the foods eaten from day to day and still consume a reasonably constant number of calories with a relatively fixed distribution of calories among macronutrients and meals as shown in Tables 13-3 and 13-4.
A widespread misconception among diabetics is that by spending more money for special “diabetic foods” they can eat what they like and still control their disease. Although sugar alcohols such as sorbitol have, on average, about half the calories of nutritive sweeteners such as sucrose, most dietetic foods contain a significant number of calories. In fact, some products contain as many calories as the comparable regular foods. More importantly, however, is the fact that there is no evidence to indicate that such foods have any therapeutic advantage over regular foods in the care of, control of, or complications of diabetes. Nonnutritive sweeteners are commonly used as adjuncts in the management of both weight and glycemia. While these agents are generally recognized as safe by various expert scientific panels that have studied the issue, there remain somewhat mixed data of variable quality on whether their use, per se, is particularly helpful in achieving weight loss (81–84).
Alcohol is a component of the diet of many Americans. Most diabetics can consume limited amounts of alcohol, but several considerations peculiar to diabetes must be kept in mind. First, as with healthy people, daily alcohol intake should be limited to modest consumption, generally interpreted to mean no more than two drinks daily in men and one in women. Because alcohol contains a significant number of calories (7 kcal per g), it is difficult to fit much alcohol into a weight loss diet and still obtain adequate protein, vitamins, and minerals. In the obese diabetic, alcohol consumption should be minimized or eliminated until the excess weight is lost. Further some alcoholic beverages, especially beer and sweet wines, contain a substantial number of calories in the form of carbohydrate in addition to those in alcohol. These may contribute to hyperglycemia and must be considered in the diet. When using the exchange list system, alcohol is substituted for fat exchanges: 1 oz of whiskey is the equivalent of two to three fat exchanges; 12 oz of low-calorie beer is the equivalent of two fat exchanges.
It is also important to recognize the effect of alcohol consumption on blood glucose. This effect depends on such factors as time, consumption of other foods, and type of beverage. In fasting, insulin-dependent diabetics, the ingestion of a large amount of alcohol can induce profound hypoglycemia by inhibiting the release of glucose from the liver. Alcohol can also impair hepatic glucose production and, consequently, counter-regulation in insulin-induced hypoglycemia (79). This effect may be most pronounced in tightly controlled patients with an increased incidence of hypoglycemia. Because the symptoms of hypoglycemia closely resemble those of alcohol intoxication, the hypoglycemia may go unrecognized, leading to significant detrimental consequences. To minimize the risk of nocturnal hypoglycemia when taking insulin or oral hypoglycemic agents, diabetics should only consume alcohol with food. Alcoholism and insulin-dependent diabetes are an unfortunate combination of diseases.
Alcohol intake can also cause an increase in circulating triglyceride concentration and diabetes is frequently accompanied by hyperlipidemia. Fortunately, control of the blood glucose and achievement of ideal body weight may return the triglycerides levels to normal, so that moderate alcohol consumption can be allowed. Finally, some patients taking sulfonylureas experience flushing, nausea, dyspnea, and palpitations when they drink alcohol. This reaction, which resembles the effect of disulfiram (Antabuse), can be prevented by taking antihistamines before alcohol, although the preferred solution is abstinence.
Modifying the Diet for Illness
In both type 1 and type 2 diabetics who are acutely ill, two general principles always apply. First, insulin or oral hypoglycemic agents should be continued. Second, enhanced testing of plasma glucose and ketone values are necessary, as are drinking adequate amounts of fluids and ingesting sufficient amounts of carbohydrates. Cohen and Edelstein (85) have provided practical guidelines for sick-day management of individuals with diabetes, and Nyenwe and Abbas (86) have published extensive, evidence-based guidelines for the management of hyperglycemic crises in diabetics.
Acute illnesses are frequently accompanied by nausea, vomiting, and anorexia. In patients with type 2 diabetes, acute illness usually does not have a markedly adverse effect on diabetic control. For these patients, the major concern is avoiding dehydration by ensuring adequate fluid intake, usually by frequently ingesting small volumes of liquids and soft foods. Ingestion of at least 150 g of carbohydrate are recommended to reduce starvation ketosis. Occasionally, during an intercurrent illness such as influenza, insulin dependence develops in a patient with non–insulin-dependent diabetes; all diabetic patients should carefully monitor their blood glucose and their urine for the appearance of ketonuria during acute illness (85).
In individuals with type 1 diabetes, acute illness may result in profound hypoglycemia or in profound hyperglycemia and ketosis. Usually, the acute illness tends to increase insulin requirements. The only way to assess the insulin need is by frequent monitoring of blood glucose and urine ketones. The diabetic patient must take in enough carbohydrate (>150 g per day) to prevent starvation ketosis. To prevent hypoglycemia during bouts of illness when appetite is depressed, adequate carbohydrate can be obtained by the frequent ingestion of sweetened fluids and soft, easily digested foods such as ice cream, juices, sweetened Jell-O, and soups. The ingestion of small amounts of fluid on a 15- to 30-minute basis helps to prevent dehydration (see Tables 7-10 and 10-17 for selected oral rehydration solutions and Reference 86 for a detailed discussion of fluid management in hyperglycemic patients).
Modifying the Diet for Activity
Patients with non–insulin-dependent diabetes do not have to alter their diet to accommodate changes in exercise patterns. Exercise is a useful adjunct to caloric restriction in the attempt to lose weight. The overweight diabetic who begins an exercise program should gradually lose weight if the caloric intake is constant. However, it should be emphasized that daily regular physical activity of all kinds is beneficial to persons with type 2 diabetes. The diabetic patient should not be led to interpret “physical activity” as traditional “exercise” (e.g., running, tennis) but made to understand that all forms of nonsedentary behavior are valuable. Brisk walking is one of the most beneficial activities for obese diabetics and has been shown to be an effective adjunct in reducing the risk for coronary heart disease.
Changes in exercise patterns in patients with insulin-dependent diabetes, however, must be accompanied by adjustments in the diet and insulin doses. Obviously, regular exercise every day at a set time, at a set level of activity, for a set length of time is easily accommodated to the person’s diabetic dietary regimen. Irregular exercise is a more difficult problem. An hour of moderate to vigorous exercise (e.g., cycling, basketball) will likely require extra dietary carbohydrate intake. If the exercise is to last for less than 2 hours, the consumption of extra food can be delayed until the exercise is complete and the patient determines whether any food is necessary by measuring the blood glucose. Often, however, depending on the subject’s prior history of hypoglycemia with moderate exercise for this length of time, a snack of 10 to 15 g of carbohydrate may be consumed before exercise. Among the foods that may be eaten before exercise are low-fat cottage cheese, fruit, yogurt, bread, and crackers. These should be eaten about 30 minutes before exercise. If exercise is vigorous or of long duration, the athlete may need to consume a carbohydrate-rich snack every 30 minutes. The amount of food required to cover exercise depends on body size and the duration and intensity of activity.
Diabetes in Special Groups
Diabetes in Pregnant Women
Insulin-requiring diabetes is frequently more difficult to control during pregnancy, and pregnancy may cause glucose intolerance in a woman with previously normal glucose control. Special modifications of the diabetic diet are not needed to accommodate the additional requirements of pregnancy other than those recommended for pregnant women in general, as described in detail in Chapter 3. However, the normal consequences of pregnancy, such a morning sickness, may disrupt the patient’s previously established eating patterns and established insulin regimens.
Weight gain during pregnancy is frequently excessive in diabetic patients and the consequences of obesity in pregnancy are substantial as discussed in Chapter 3. It is preferable to control body weight before pregnancy or, if not possible, to prevent excessive weight gain during pregnancy (Chapter 3). Excessive weight gain during pregnancy also magnifies the physiologic increase in insulin resistance that is a normal consequence of pregnancy. During the second and third trimesters, insulin requirements increase substantially, often as much as 50% to 100%, and additional dietary manipulations may be necessary to accommodate this increase. At the same time, the practitioner must be alert to the fact that the insulin requirement will drop immediately after delivery and the insulin dose lowered to prevent hypoglycemia.
Diabetes in Children
Diabetes is one of the most common serious chronic diseases of childhood. Whereas most adults with diabetes do not require insulin and are obese, 98% of children below the age of 10 with diabetes require insulin, and few are obese. More recently, however, as discussed earlier, the incidence and prevalence of obese, type 2 diabetics has increased substantially in adolescents.
The goals of dietary therapy in children with diabetes are the same as in adults with diabetes, but in addition, dietary therapy must promote normal growth and development. The calorie requirements for children are based on sex, age, size, growth rate, and physical activity and age and gender-specific energy intake recommendations are available elsewhere (79,80). Nevertheless, diets whose calculated energy intakes are constructed from these algorithms are only general guidelines and each child’s actual caloric requirement must be determined individually by trial and error. During adolescence, caloric requirements increase with the rate of growth. The energy cost of growth is about 120 kcal per day at peak growth during adolescence, although this still represents only a small percent of the total energy requirement. The energy requirements of individual adolescents vary markedly. The total daily expenditure of energy in adolescent boys ranges from about 50 to 75 kcal per kg and in adolescent girls is between about 50 and 65 kcal per kg, respectively, depending on their resting metabolic rate and their habitual level of physical activity. The calorie allotments for children should be modified according to their longitudinal progress on their own, individual weight, and height charts. Many juvenile diabetics are underweight at the time of diagnosis. A common error is to place underweight children on a diet calculated to meet the needs of children of normal weight of the same age. Underweight children may require an additional several hundred calories per day to regain lost weight and maintain growth.
Diabetes in Patients with Hypertension, Hyperlipidemia, or Renal Disease
Diabetes is frequently associated with other chronic illnesses for which the management includes diet therapy. Chronic renal failure is a common complication of diabetes. Elevated plasma cholesterol and triglyceride levels are common among diabetics. Finally, hypertension, although not directly related to diabetes, is common among the obese, middle-aged patients who comprise the majority of the diabetic population. Because each of these illnesses is treated in part by dietary measures, the formulation of a diet for patients with more than one of these illnesses requires additional planning. Fortunately, the diets used in the management of these several illnesses do not conflict with, but frequently complement, each other.
Patients with Diabetes and Chronic Kidney Disease
Recently, the National Kidney Foundation reported clinical practice guidelines for individuals with diabetes and kidney disease (87) and the subject was also recently reviewed by others (88). For diabetics with kidney disease, other than end-stage renal disease, a target daily protein intake of 0.7 to 0.8 g per kg body weight is recommended, with additional protein intake, as necessary, to replace urinary protein losses in patients with significant albuminuria and minimize the risk of protein–energy wasting (89). Nonetheless, the results of the Modification of Diet in Renal Disease Study, the largest of its kind ever conducted, were inconclusive when attempting to show whether a reduction in dietary protein intake from 1.3 g/kg/d to 0.58 g/k/d would slow the progression of nondiabetic renal kidney disease (90). When consuming dietary protein intakes at the level of 0.8 g/kg/d, individuals must take care to consume dietary proteins of high biologic value, as described later in this chapter. In addition, patients must restrict their intakes of phosphorus, potassium, and sodium. None of these requirements should interfere with the recommended diabetic diet. The principal medical and pharmacologic therapeutic guidelines for the care of diabetics with kidney disease are directed toward control of hyperglycemia, hypertension, and hyperlipidemia. The dietary recommendations for nutritional control of these entities are fundamentally encompass the healthful diet patterns that are proposed for normal healthy adults (Chapters 1 to 3). In this context, one must be aware that the DASH and DASH-sodium diets that are fruit, vegetable, and grain-based diets that have been shown to be effective in reducing blood pressure are relatively high-protein diets with a daily protein intake on the order of 1.4 g/kg/d. Thus, applying the DASH diets to chronic kidney disease requires modification of the protein intake to comply with consensus recommendations (89), although modifications may not necessarily be especially restrictive based on the results of the Modification of Diet in Renal Disease Study (90).
Patients with Diabetes and Hyperlipidemia
Hypertriglyceridemia occurs in about 20% of patients with type 2 diabetes. Management regimens, including appropriate blood glucose control with insulin or oral agents, reduction of alcohol intake, and caloric restriction to attain ideal body weight, should result in reduced blood levels of both lipids and sugar. The dietary approach to hyperlipidemia is discussed in more detail later in this chapter.
Patients with Diabetes and Hypertension
The major dietary manipulations in the treatment of hypertension are caloric restriction, to help obese patients lose weight, sodium restriction (see Chapter 12) and the implementation of a healthful dietary pattern such as the DASH diet plan based on increased fruit and vegetable intake with elimination of foods that are both high in calories and in sodium, such as processed dinners and snacks, cold cuts, sausage, French fries, salted nuts, and snack chips. In this context, to be successful it is critical to read the nutrition facts label of every purchased product. Eliminating these items helps control both diabetes and hypertension (see Chapter 12 for a discussion of the low-sodium diet). Regular exercise and a reduced alcohol intake are also useful proven adjuncts in the treatment of hypertensive diabetics. The recommended pharmacologic approaches can be found in References 87 and 88.
RENAL DISEASE
General Principles
Diet therapy is an essential component of the management of renal disease. As mentioned above, although overall clinical practice evidence points to the fact that low-protein diets will retard the progression of chronic renal disease, unequivocal demonstration of this thesis remains elusive following the inconclusive (but largely negative) outcome of the largest systematic, randomized trial conducted to test this hypothesis (90,91). Mitch and Ikizler (92) and Kopple et al. (93) have published the most recent, detailed analyses of nutritional management of individuals with impaired kidney function.
The goals of diet therapy are to (a) provide adequate nutrition, (b) minimize uremia and other metabolic derangements, (c) reduce the risk factors for cardiovascular disease and other comorbid conditions, and (d) delay the progression of renal failure. Improvement in the patient’s metabolic status reduces the symptoms associated with uremia, including fatigue, nausea, pruritus, and anorexia.
A wasting syndrome in uremic patients is secondary to inadequate dietary intake of protein and energy, altered protein metabolism, and the endocrine abnormalities associated with renal failure (hyperparathyroidism, insulin resistance) (89,92,93). In addition, patients on dialysis lose nutrients into the dialysate, which further contributes to wasting. A major factor contributing to malnutrition in chronic renal failure is the anorexia caused by uremia. Uremia also diminishes taste acuity, so that food seems bland and unappealing. Proper nutrition may help reverse the wasting syndrome. For example, the correct manipulation of dietary protein can reduce the degree of uremia; if the patient’s appetite improves, the caloric intake increases and wasting is reversed. Total parenteral nutrition (TPN) can be used in patients with chronic renal failure, whether they are managed conservatively or on dialysis, although this represents a management aid in complex rather than routine situations. The use of TPN in the renal patient is covered in Chapter 11 (Table 11-11). However, what has also become apparent in the last several years is that protein loss is associated with increased inflammatory cytokines that augment protein wasting by as yet incompletely understood mechanisms (94–96). As a consequence, trying to correct protein loss by simply feeding more protein is unsuccessful. Additionally, there is now substantial evidence that metabolic acidosis is a major contributor to both protein and bone loss in chronic kidney disease (97,98). The latter realization has led to therapeutic regimens aimed at treating the acidosis and raising serum bicarbonate levels into the normal range. Such treatment regimens have been shown to slow the loss of residual renal function (97). Perhaps as surprising is recognition that increasing dietary fruit and vegetable intakes as a means of decreasing metabolic acidosis are also effective and may provide a mechanism for the beneficial effects of the DASH diet plan (98).
The dietary management of chronic renal disease depends on the degree of renal failure and the need for dialysis. The National Kidney Foundation has published its clinical practice guidelines for chronic kidney disease that divides chronic renal disease into five stages (99):
Stage 1: Kidney damage with normal or increased glomerular filtration rate (GFR) ≥greater than or equal to 90 mL/min/1.73 m2
Stage 2: Kidney damage with mildly decreased GFR between 60 and 89 mL/min/1.73 m2
Stage 3: Moderately decreased GFR (30 to 59 mL/min/1.73 m2)
Stage 4: Severely decreased GFR (15 to 29 mL/min/1.73 m2)
Stage 5: Renal failure (GFR less than 15 mL/min/1.73 m2) (or receiving dialysis)
While nutritional modifications are important in every stage of declining renal function, clearly the most stringent attention to nutrient intake must take place in Stage 5 renal disease when every aspect of nutrient ingestion and excretion are under conscious control of both the patient and the physician.
Phases of Management
Chronic Kidney Disease in Nondiaylzed Patients
Protein Quantity
In the predialysis phases of chronic kidney disease, renal function has not progressed to the point at which dialysis is indicated, but dietary therapy is a critically important component of medical care. Dietary protein intake is generally not restricted in people with Stage 1 or Stage 2 renal disease (GFR greater than 60 mL/min/1.73 m2). For individuals with Stage 3 renal disease, recommendations for dietary protein restriction are somewhat variable since the data to support these recommendations are less clear. However, recommended dietary protein intakes in the range of 0.6 to 0.75 g protein/kg/d is generally the rule (92,93) with the initial goal toward the lower end of this range. It is important that the dietary proteins consumed are those of high biologic value such as found in meat, fish, and eggs. When renal disease progresses to Stage 4, dietary protein intake should be reduced to 0.60 g protein/kg/d (92,93). Available evidence supports the fact that this dietary approach can maintain good nutritional status, good metabolic status, limit symptoms of renal disease, and its secondary effects (e.g., renal osteodystrophy), while possibly slowing the progression of renal failure. Individuals with chronic renal disease who have nephrotic syndrome represent a special therapeutic category because of body protein loss via proteinuria. In these cases, dietary protein intake is liberalized somewhat to replace the irreversible loss of protein in the urine. Recommendations for protein intake average about 0.8 g protein/kg/d, but values range from about 0.7 g to 1.0 g (92,93). There is another very important modification of the dietary prescription, specifically recommended consumption of an additional 1.0 g of high biologic value protein per day for each gram of urinary protein lost above 5 g per day (93). In all instances above, compliance with dietary protein intake recommendations is followed by measuring urinary nitrogen excretion (92,93).
Protein Quality
As renal disease progresses, the ability of the kidneys to excrete urea diminishes. The load of urea presented to the kidneys is usually proportional to the amount of protein in the diet; however, even patients on a zero-protein diet produce some urea as a result of the breakdown of tissue proteins. If too much protein is included in the diet, the level of blood urea nitrogen (BUN) rises and symptoms of uremia develop. However, when dietary protein intake is restricted too much as a therapeutic or preventive strategy, the supply of essential amino acids required for the synthesis of necessary proteins becomes inadequate and protein malnutrition is the consequence. Further, it is not only important for a patient to consume an adequate amount of protein; it is also important that the protein be of the right type or quality. Dietary amino acids are classified as either nonessential or essential. Because the body cannot synthesize essential amino acids, they must come from the diet. Nonessential amino acids can be ingested or synthesized. In chronic renal failure, endogenous synthesis of the nonessential amino acids is preferable to ingestion for two reasons: The synthesis of an amino acid uses up an amino nitrogen that would otherwise go into urea, and the synthesis of an amino acid reduces the amount that amino acid that must be consumed in dietary protein. The net effect of both processes is diminished substrate for urea production. An alternative to this approach is the use of dietary α-keto acid analogues of essential amino acids. Via transamination, these keto acids are converted into the respective essential amino acids, so that an amino acid group is salvaged, while simultaneously an essential amino acid is provided. However, the evidence basis for whether this option can retard the progression of renal failure is largely negative and diets containing keto acid supplements are not recommended (93).
Essential amino acids, on the other hand, must be present in the diet (as protein) in quantities adequate to allow the required level of body protein synthesis. Moreover, in uremia, the conversion of phenylalanine to tyrosine is impaired and uremics also appear unable to synthesize enough histidine to meet their needs. Thus, these two amino acids (not normally essential in healthy individuals) become conditionally essential amino acids in patients with chronic kidney disease. The need to supply a sufficient amount of essential amino acids in the diet can become problematic in patients with chronic kidney disease since, to reduce generation of urea, they must restrict dietary protein intake. To accomplish both goals (sufficient essential amino acids and limited urea production) while maintaining adequate synthesis of body proteins, it is essential that the proteins these individuals consume have the maximum amounts of essential amino acids per gram of protein. Proteins of high biologic value meet this requirement, since most of the nitrogen is in the form of essential amino acids, all essential amino acids are present in the protein, and the essential amino acids are present in concentrations proportional to the minimum daily requirements. Eggs are the food with proteins of the highest biologic value. Other foods with proteins of high biologic value include fish, poultry, lean meat, and dairy products, while proteins of low biologic value are found in various grains, nuts, seeds, and legumes.
Other Diet and Lifestyle Recommendations
With few exceptions, such as dietary protein intake discussed above and the others discussed below, overall nutrition and lifestyle guidelines for individuals whose GFR are greater than about 30mL/min/1.73 m2 are largely consistent with those recommended in the Dietary Guidelines for Americans and the American Heart Association dietary guidelines (as discussed in Chapter 1), those discussed for people with diabetes earlier in this chapter, and the report of the Joint National Committee on Prevention, Detection, Evaluation and Treatment of High Blood Pressure (JNC 7) (100). Blood pressure control in patients with chronic renal disease is particularly important because lower systolic blood pressure is predictive of a more positive clinical outcome (101–105) and antihypertensive drug therapy is part of routine clinical care (106–107). Nonetheless, more recent data have questioned overly aggressive attempts to control blood pressure because very strict control of both systolic and diastolic blood pressures is associated with increased all-cause mortality in individuals with chronic kidney disease (108,109). Thus, some have recently recommended an optimal blood pressure range of 130 to 159 systolic and 70 to 89 diastolic in patients with chronic renal disease (108,109). In the context of blood pressure and extracellular fluid volume control, dietary sodium restriction has long served as a mainstay of the clinical management of patients with chronic renal disease (92,93). Like the data above suggesting moderation in blood pressure goals, recent dietary sodium intake data and their relationship with mortality, both in the general population and in patients with chronic renal disease, have suggested that more modest sodium restriction is the most prudent course of action in chronic renal disease subjects whose GFR are not extremely low, requiring dialysis (110–116).
In addition to the restricted protein and sodium intakes discussed above, individuals with chronic kidney disease require attention to their dietary intakes of potassium and phosphorus. Since the kidney is the predominant route for potassium excretion, one might expect that limitation of potassium intake is necessary once GFR begins to fall. However, as GFR falls, serum potassium levels tend to remain normal as long as urine volume is more than about 1 L daily (93) because both renal tubular and fecal excretion of potassium increase (92,93). Thus, it is generally not necessary to restrict dietary potassium intake until it is necessary to limit sodium intake in persons with chronic renal disease as the GFR declines. In fact, if hyperkalemia develops, one should be alert to the possibility of constipations, increased catabolism, academia, hyperaldosteronism, and unwanted side effects of antihypertensive drugs (92,93). As GFR declines to the lower levels found in Stage 3, potassium restriction to under about 4 g daily is sometimes necessary. In Stage 4, potassium intake is restricted to approximately 2 to 2.5 g daily (92,93).
Physicians are now increasingly recognizing the role of excess phosphate intake and hyperphosphatemia in elevating the risk of detrimental cardiovascular events in patients with chronic kidney disease (117,118). Furthermore, serum phosphorus control is a significant clinical issue because hyperphosphatemia can lead to the development of hyperparathyroidism as well as calcium phosphate deposits in soft tissues and arteries (92,93). Since phosphorus is widely present in foods, especially in animal products, as phytates in plants and as additives in a broad array of food products, reducing dietary phosphorus intake is no trivial undertaking. It is especially important to recognize what is now called “hidden phosphorus” present in many processed foods and popular beverages (119–131). Although there is some lack of consensus and because it is very difficult to keep a very-low-phosphorus diet, there is some lack of consensus on the optimal intake of phosphorous in clinical practice, but recommended intakes are largely in the range of 0.8 to 1.0 g phosphorus per day (92,93,99).
Table 13-5 shows a summary of the major nutritional recommendations for individuals with chronic kidney disease who do not require dialysis (93) and recommendations that are similar overall in principle, but not necessarily identical in practice, may be found in other expert assessments (Reference 92, for example). Thus, it is important to recognize that the values in Table 13-5 are only initial estimates. In clinical practice, it is essential to tailor dietary nutrient intakes on an individualized basis. This is particularly true because required essential nutrient intakes are not well defined in patients with chronic renal disease and because they are known to vary because of the progressive decline in GFR.
Chronic Kidney Disease Requiring Dialysis
Protein Intake
When the GFR falls to approximately 5 mL/1.73 m2/min (advanced Stage 5 chronic kidney disease), dietary manipulation alone can no longer control the metabolic abnormalities associated with renal failure, and transplantation or dialysis is required. Even if the subject’s GFR is in the upper ranges of Stage 5 chronic kidney disease, dialysis should be considered if nutritional status and body weight are difficult to maintain. The goals of diet therapy for patients on dialysis are to minimize metabolic abnormalities, correct for nutrients lost during dialysis, and provide sufficient nutritional support to prevent the protein–energy malnutrition that is commonly seen in patients undergoing maintenance dialysis (92,93,99).
Nutritional Requirements for Patients with Chronic Renal Failure (93) |
| Chronic Kidney Disease Prior to Dialysis | Chronic Renal Failure Requiring Maintenance Dialysis |
Dietary Nutrients |
|
|
Protein | 0.6–0.75 g/kg/d (of which ≥0.35 g/kg/d is protein of high biologic value) | Hemodialysis: 1.1–1.2 g/kg/d (≥50% high biologic value) |
Peritoneal dialysis: 1.2–1.3 g/kg/d |
|
|
Energy | >35 kcal/kg/da | >35 kcal/kg/da |
Fat | 30%–40% energy | 30%–40% energy |
P:S fat ratio | 1:1 | 1:1 |
Carbohydrate | Rest of nonprotein calories | Rest of nonprotein calories |
Fiber | 20–25 g/d | 20–25 g/d |
Fluids | Up to 3 L/db | Usually 750–1,500 mL/db |
Sodium | 1–3 g/db | 0.75–1.0 g/db |
Potassium | 40–70 mEq/d | 40–70 mEq/d |
Phosphorus | 5–10 mg/kg/d | 8–17 mg/kg/d |
Calcium | 800 mg/d | 800 mg/d |
Magnesium | 200–300 mg/d | 200–300 mg/d |
Iron | ≥10–18 mg/dc | ≥10–18 mg/dc |
Zinc | 15 mg/d | 15 mg/d |
Vitamin Supplements |
|
|
Thiamine | 1.5 mg/d | 1.5 mg/d |
Riboflavin | 1.8 mg/d | 1.8 mg/d |
Pantothenic acid | 5 mg/d | 5 mg/d |
Niacin | 20 mg/d | 20 mg/d |
Pyridoxine HCl | 5 mg/d | 5–10 mg/d |
Vitamin A | 0 | 0 |
Folic acid | 1 mg/d | 1 mg/d |
Vitamin B12 | 3 µg/d | 3 µg/d |
Vitamin C | 70 mg/d | 730 mg/d |
Vitamin D | 800 mg/dd | See textd |
Vitamin E | 15 mg/de | 15 mg/de |
Vitamin K | 0 | 0 |
aUnless patient is overweight, obese, or is gaining unwanted weight. See Reference 93.
bCan be higher in patients with greater urinary or dialysis losses. See Reference 93.
cTen milligrams for men and nonmenstruating women; 18 mg for menstruating women.
dInitial estimates only. Adjustments to intake recommendations are best made on the basis of circulating 25OHD levels. See Reference 93 for discussion.
eAs α-tocopherol (includes RRR-α-tocopherol and the 2R-stereoisomeric forms).
(Data from Kopple JD, Ross AC, Caballerio B, et al. Nutrition, diet and the kidney. In: Modern Nutrition in Health and Disease. 11th ed. Philadelphia: Wolters Kluwer; 2014; see text.)
In the absence of dialysis, the argument for limiting dietary protein intake is far more compelling in Stage 5 chronic renal disease. In this circumstance, a reduction in dietary protein intake not only reduces the generation of deleterious nitrogenous metabolic products but also leads to a concomitant reduction in the intake of both phosphorus and potassium, which generate additional deleterious metabolites. However, since these patients are at high risk of malnutrition and the long-term consequences of uremia, and since there is no evidence that withholding dialysis and limiting protein intake leads to a better outcome than instituting dialysis and providing a more liberal diet, most nephrologists recommend that maintenance dialysis (or renal transplantation) commence at this stage of deterioration of renal function.
When dialysis is successfully established, protein intake can be liberalized somewhat because of the nitrogen removed during dialysis. For patients undergoing maintenance hemodialysis, the recommended daily dietary protein intake is 1.2 g protein per kg body weight and for those undergoing peritoneal dialysis, it is 1.2 to 1.3 g protein per kg body weight (74,75) (Table 13-5). At least half of the dietary proteins consumed should be high-biologic-value proteins. This level of protein intake avoids the protein–energy malnutrition that can occur at lower intake levels due to the reduced capacity of these patients to conserve body proteins, amino acids, and other biologically important nitrogenous compounds are lost in the dialysate. In hemodialysis, losses are estimated to be as high as 1 g of free amino acids per hour and 3 g of total (free plus protein-bound) amino acids per hour. Thus, a standard 4-hour session of hemodialysis results in losses equivalent to 5 to 10 g of protein. Because a large proportion (30% to 40%) of the amino acids lost in the dialysate are essential amino acids, it is critical that at least half of the dietary protein be of high biologic value, as noted above. For similar reasons, patients undergoing long-term peritoneal dialysis may require even a greater intake of protein than patients on hemodialysis. The “pores” of the peritoneum are larger than those of dialysis tubing and so allow even larger proteins to be lost into the dialysate. On average, these patients lose 10 to 14 g of protein, peptides, and amino acids daily into the dialysate fluid.
Energy Intake
Daily maintenance energy intake in dialyzed patients is set at 35 kcal per kg body weight for those who are less than 60 years of age and 30 to 35 kcal per kg body weight for those older than 60. These are target values. If an individual is obese, overweight, or gaining weight at an accelerated rate, energy intake should be reduced. Likewise, individuals who are losing weight may require a higher energy intake.
An adequate intake of calories in the form of carbohydrates and fats is required to prevent the use of dietary or tissue protein as a source of energy. The catabolism of proteins results in increased urea production. The ideal calorie intake is whatever is required to achieve and maintain an ideal body weight. Patients who are below their ideal body weight need more calories. Unfortunately, the average calorie intake of dialysis patients is frequently lower than 30 kcal/kg/d and patients with chronic renal failure are often energy-deficient as manifested by weight loss, diminished adipose tissue, and loss of muscle mass. Because these patients also tend to be volume-expanded, their muscle mass and fat stores may be even lower than their weight might suggest.
Dietary instructions for renal patients must be complete, given by professionals, and reinforced regularly. Achieving an adequate caloric intake is a major problem in the management of patients with chronic renal failure. In some cases, compliance with other dietary restrictions must be sacrificed to attain an adequate caloric intake. The vigorous pursuit of perfect metabolic balance may result in a diet that is totally unpalatable and a patient with an inadequate calorie intake.
Because they are frequently anorectic and have difficulty in maintaining an adequate calorie intake, patients with chronic renal failure may require nutritional supplements that supply energy. Many commercial nutritional supplements are now available that are especially appropriate for patients with renal failure. In some supplements, the calories are all carbohydrate (e.g., G2 Gatorade) (Table 10-17). Other supplements are more specific to renal failure; they contain a mixture of fat and carbohydrate and are designed to be low in protein, phosphorus, sodium, and potassium for readily apparent reasons (e.g., Nepro). Table 10-4 lists the supplements specifically intended for patients with renal disease, and Table 10-5 provides the detailed nutritional content of some of them.
Water Intake
Water requirements are not usually a major problem for patients with chronic renal failure who are not receiving dialysis. Fluid intake should equal insensible loss (water lost from skin and lungs, which is usually 400 to 600 mL per day) plus urine volume. Most conservatively managed patients do well on 1.5 to 3.0 L per day. The goal of fluid (and salt) management in the patient who are receiving dialysis is to limit the rate of weight gain between dialysis treatments to 1 lb per day. This can be achieved with a diet containing 750 to 1,000 mg of sodium plus a water intake in the range of 750 to 1,000 mL daily (Table 13-5). A daily fluid allotment of 1,000 mL includes 500 mL for insensible losses and 500 mL for a 1-lb weight gain. Fluid in food must be subtracted from the fluid allotment. Any food that is liquid at room temperature (e.g., gelatin, ice cream) is counted as a fluid. Fruits and vegetables are 85% to 90% water, cooked cereals are 70% to 85% water, and meat is 45% to 60% water. It should be readily apparent that 1,000 mL per day is a severe fluid restriction; compliance is difficult for many patients.
In patients undergoing long-term peritoneal dialysis and in those on hemodialysis who have greater urinary losses, water and sodium intakes may be increased. Thus, in the absence of anuria, urinary salt and water losses can be added to the restrictive allowances above. Stringent salt restrictions may improve metabolic control but make the diet unpalatable. Nonetheless, patients with hypertension and congestive heart failure may have to reduce their salt intake.
Sodium Intake
Recommendations for dietary sodium intake are outlined in Table 13-5, and sodium-restricted diets are readily available. One must consider modest sodium restriction when possible because of the growing concern about the potentially detrimental consequences of severe sodium restriction as discussed earlier in this chapter. Little sodium is excreted in the feces, and as chronic renal disease progresses, the ability of the kidneys to respond to variations in sodium intake diminishes. The normal kidney responds to a low-sodium diet by reabsorbing virtually all the filtered sodium and responds to a high-sodium diet by reabsorbing less. The failing kidney progressively filters and reabsorbs less sodium and cannot adapt to changes in sodium intake. When this happens, edema, hypertension, and congestive heart failure may ensue. On the other hand, too little dietary sodium intake can result in dehydration, a reduced GFR, and acceleration of the deterioration in renal function. The goal is to have the daily sodium intake equal the fixed daily loss. Recently, Smyth et al. (132) systematically reviewed the data on sodium intake and renal outcomes in individuals with and without kidney disease. They concluded that high sodium intakes (more than 4.6 g per day) are likely detrimental, a conclusion supported by all of the data collected by others about adverse CVD outcomes in people without renal disease (110–114). Even so, the data in patients with renal disease are limited. On the other hand, Smyth et al. (132) found that the association of detrimental renal outcomes with low sodium intakes is uncertain due to inconsistent findings in available published reports. Again, this finding is consistent with other recent data on low dietary sodium intakes and adverse clinical outcomes (110–114).
Most patients with renal disease with GFR greater than 5 mL per minute will tolerate dietary sodium intakes in the range of 1 to 3 g per day (40 to 130 millimol per day), although the actual intake must be individualized to the patient. Further deterioration of renal function requires restriction and control over sodium intake to a level approximately half that immediately above. Following initiation of dialysis, the restriction may be liberalized somewhat because sodium output into the dialysate can be increased and managed to some degree. Patients with renal disease are often placed on diets in which the sodium restrictions are excessively stringent. A rising BUN may reflect dehydration instead of excessive protein intake. Further, not all forms of chronic renal failure affect sodium homeostasis in the same way. Pyelonephritis and polycystic kidney disease tend to be salt-wasting conditions requiring increased dietary sodium, and glomerulonephritis is associated with hypertension that might require a lower salt intake.
In any case, dietary sodium requirements change with nonurinary salt losses, primarily those in sweat. Sodium restrictions must be liberalized in warm climates for patients whose homes are not air-conditioned. Some patients require sodium bicarbonate for the treatment of acidosis, a management option that is now becoming more frequent because of demonstrated benefits (97,98). In such cases, dietary sodium must be reduced to compensate for the sodium in the sodium bicarbonate if that is the alkalinizing agent used. Two grams of sodium bicarbonate contain the same amount of sodium as 1.5 g of salt.
Potassium Intake
For the reasons discussed above, individuals with chronic kidney disease do not require significant dietary potassium restriction until GFR and urine volume fall to very low levels, generally below about 15 to 20 mL per minute. Foods high in protein are usually also high in potassium, and, if patients with very low GFR, hyperkalemia may develop. The standard American diet contains 50 to 100 mEq of potassium per day. Patients with a GFR of less than 15 mL per minute usually require potassium restriction to 40 to 70 mEq per day. Hyperkalemia is less likely in those with a urine output of 1,000 mL or more per day. A 40-g protein diet (0.6 gm protein/kg/d for a 70-kg person) provides 50 to 60 mEq of potassium per day. Excessive dietary potassium can lead to hyperkalemia and the danger of cardiac arrhythmias. Patients with renal failure should not use salt substitutes that contain large amounts of potassium (Table 7-16). Similarly, they should not be given potassium supplements when treated with thiazides or furosemide. Hyperkalemia is exacerbated by acidosis and catabolic stress with muscle protein breakdown. These conditions should be prevented by proper medical management and corrected expeditiously when they occur. In some patients, potassium restriction may not be necessary, since, despite nearly normal serum potassium levels, the total body potassium is frequently low in chronic renal failure, since most potassium is located intracellularly. Thus, dietary potassium should not be appreciably restricted unless hyperkalemia is a problem and the urine output is below about 1,000 mL per day. Table 13-5 provides some general guidelines. The National Kidney Foundation provides guidelines on choosing foods to help regulate dietary potassium intake at http://www.kidney.org/atoz/content/potassium.
Phosphorus, Calcium, and Vitamin D Intakes
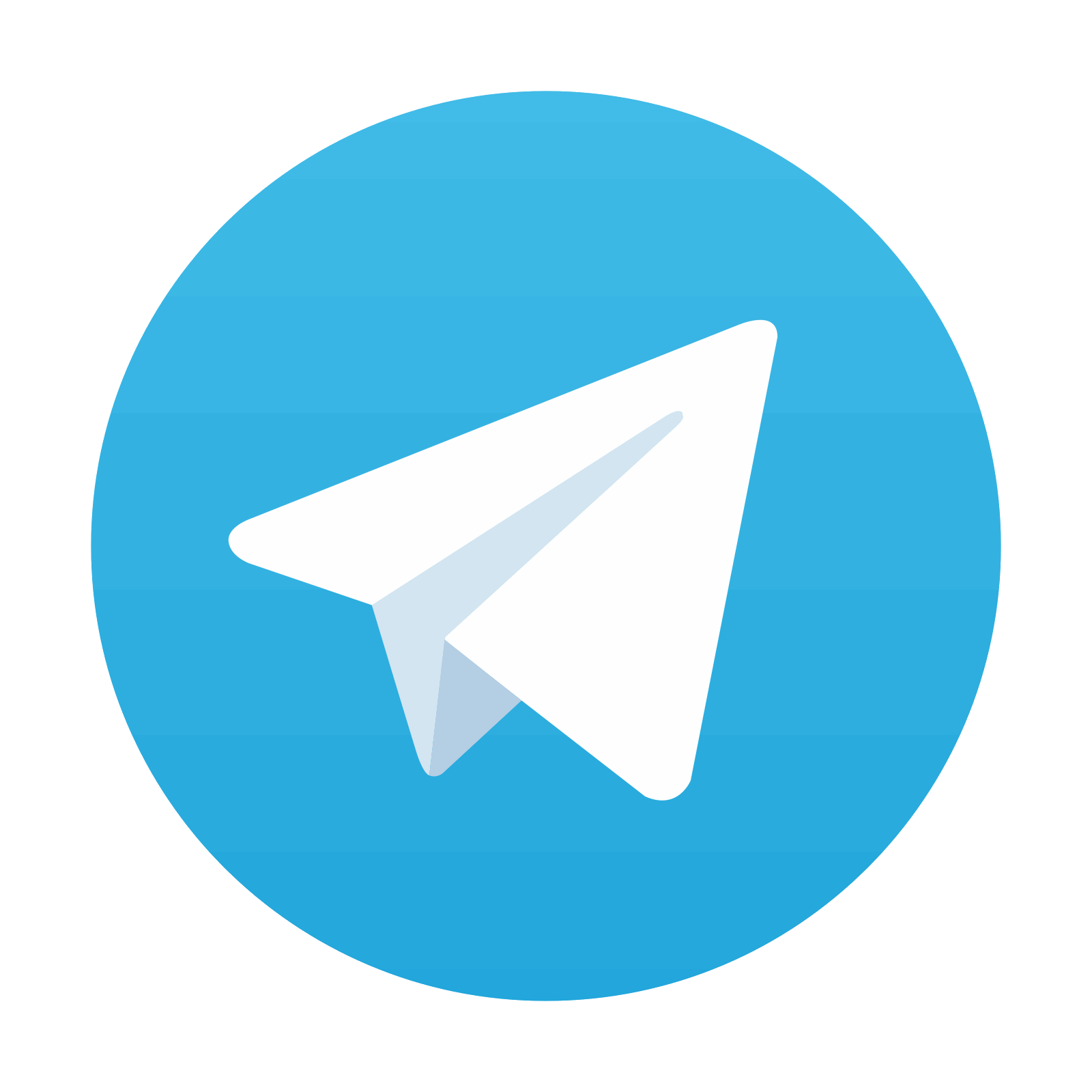
Stay updated, free articles. Join our Telegram channel

Full access? Get Clinical Tree
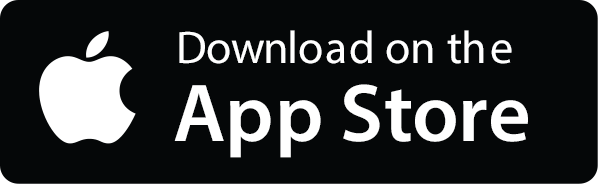
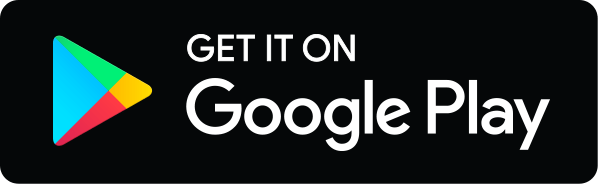