Summary of key facts
- •
The lymphatic system is a complex, blind-ended vascular tree distinct and separate from the blood circulatory system.
- •
Lymphatics facilitate tissue fluid homeostasis, nutrient uptake, and immune functions.
- •
Lymphatic vessels and lymphatic endothelial cells have unique features promoting uptake and transport back to the blood circulation.
- •
Lymphatic endothelial cell specification and differentiation are promoted by specific molecular programs.
- •
Heterogeneity and plasticity of lymphatic endothelial cells help create specialized tissue- and organ-specific lymphatic vascular beds.
- •
Lymph nodes are complex structures with unique layers of endothelial cells that function as key immune surveillance centers.
- •
Lymphatic vessels are present in the tumor microenvironment where they are active in tumor metastasis.
- •
Tumor-associated lymphatic vessels have distinct molecular profiles compared with normal lymphatic vessels.
- •
Crosstalk between the lymphatic system and tumors is important for cancer progression and metastasis.
- •
For example, VEGFC and VEGFD are major growth factors attracting VEGFR3-expressing lymphatic endothelial cells and accelerating tumor lymph angiogenesis.
- •
Most of our current knowledge regarding the function of lymphatics in cancers is limited to a few cancer types such as melanoma, breast cancer, and glioblastomas, so there is much still to be explored.
Introduction
The vascular system in our body is essential for supplying nutrients, macromolecules, and cells to tissues and organs but also critical for efficiently removing waste products. The blood and lymphatic vascular systems are responsible for these roles. The lymphatic system is a blind-ended network of vessels that runs parallel to the blood vessels and collects fluid from interstitial spaces to return it back to the bloodstream. , Although the first description of lymph nodes and glands is found in the Hippocratic treatise “On glands” from around 400 BC, , the 17th century was the golden age for the investigation of the lymphatics with several key discoveries: gut lacteals, cloacal bursa, reservoir of the chyle, extraintestinal lymphatic vessels, and hepatic lymph circulation. , In the early 20th century, Florence Sabin and other classical anatomists characterized the lymphatic system in detail using dye injection and histology to characterize fluid uptake. In addition to its important function in tissue and systemic fluid homeostasis, the lymphatic system plays a vital role in immune surveillance by regulating production and transportation of immune cells in response to immunogenic stimuli. Moreover, certain organs contain specialized lymphatic networks that support their function. For example, lacteal lymphatics absorb fatty acids in the intestine and meningeal lymphatics regulate cerebral spinal fluid homeostasis. Therefore lymphatics play numerous important physiologic roles in human health, and lymphatic research is a rapidly emerging field in cardiovascular medicine.
In this chapter, we review structural, molecular, and functional aspects of lymphatic system development and further discuss the importance of understanding this system in oncology.
Overview of the lymphatic system
The lymphatic system consists of an extensive network of vessels that transport lymph (called lymphatic vessels) and nodal centers that filter the lymph (called lymph nodes). Unlike the blood circulation, the lymphatic network is a unidirectional, low-pressure system that drains excess interstitial fluid from tissues and returns it back to the blood circulation ( Fig. 1.1 ). Interstitial fluid is collected by lymphatic capillaries (also called initial lymphatics), composed of a single layer of loosely connected lymphatic endothelial cells (LECs) that lack a continuous basement membrane, a surrounding smooth muscle cell layer, or pericytes. , Unlike endothelial cells in blood vessels joined by continuous junctions creating a tight barrier to passage of fluid and macromolecule, LECs in initial lymphatics are connected by button-like junctions that permit free passage of fluid (see Fig. 1.1 ). In addition, anchoring filaments connect the LECs of initial lymphatics to the surrounding collagenous extracellular matrix and nearby tissues. Tissue swelling from interstitial fluid buildup causes these filaments to stretch and pull on the LECs, increasing the size of the gaps between LECs, promoting increased fluid uptake and decreased interstitial pressure. Lymph contains water, macromolecules (protein and lipids), white blood cells, cell debris, and foreign antigens that are absorbed by initial lymphatics and then transported to lymph nodes via larger caliber collecting lymphatic vessels. Unlike initial lymphatics, collecting lymphatic vessels have elongated LECs that are tightly connected by zipper-like junctions as well as specialized smooth muscle cells, pericytes, and valves that facilitate unidirectional lymph flow. Protein-rich lymph is filtered in lymph nodes to prevent reentry of noxious stimuli into the circulation. Filtered lymph empties into the left (thoracic) duct and right lymphatic ducts, which then empty into subclavian veins where lymph returns to the bloodstream (see Fig. 1.1 ). Returning lymph to the circulation is critical for regulating tissue fluid homeostasis and normal blood volume and pressure. Failure of the lymphatic system to maintain fluid homeostasis results in excessive buildup of fluid in tissues (lymphedema) a disease afflicting up to 200 million individuals globally. In addition to being disfiguring and interfering with limb function and mobility, long-term accumulation of protein-rich lymph leads to further pathologic changes in tissues and skin, making this a significant cause of morbidity. Lymphedema is classified as primary lymphedema (genetic) or secondary lymphedema (acquired). Primary lymphedema is a congenital condition that causes defects and malformation of the lymphatic system. Although the causes of primary lymphedema are not fully understood, it is frequently associated with genetic mutations and developmental anomalies that disrupt lymphangiogenesis or lymphatic valve development and function. , Secondary lymphedema is an acquired disorder resulting primarily from underlying conditions that cause lymphatic obstruction. Although the most common cause of secondary lymphedema worldwide is filariasis (e.g., infection by Wuchereria bancrofti ), in developed countries lymphedema occurs most commonly as sequelae to cancer and cancer treatment. This includes surgical resection of lymph nodes and lymphatic defects caused by chemotherapy and radiotherapy. , Indeed, 1 in 5 women surviving breast cancer develops lymphedema, and over 90% of patients with head and neck cancer experience some form of lymphedema in the first 18 months posttreatment, making lymphedema one of the most common long-term comorbidities of cancer and cancer treatment.
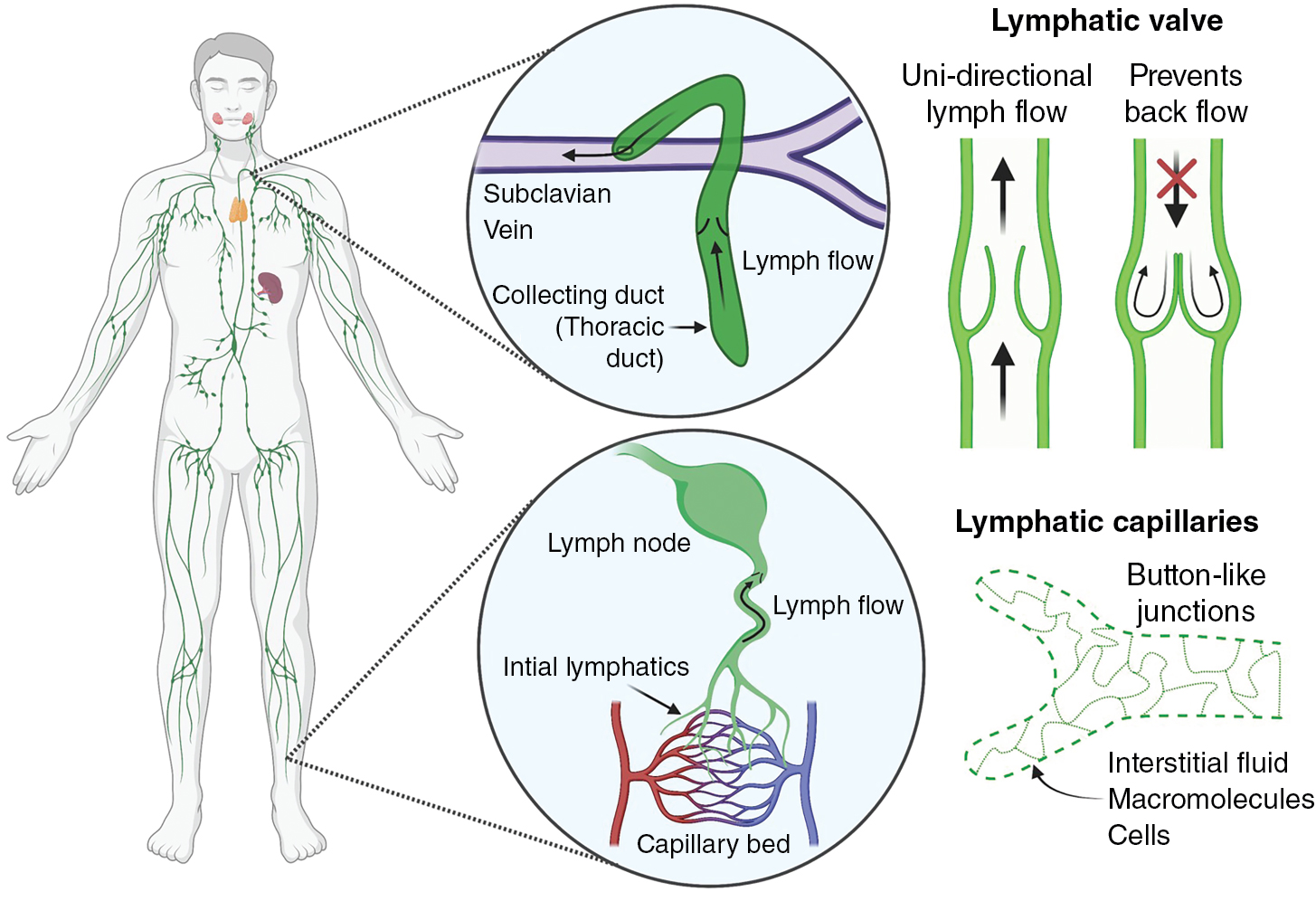
Embryonic development of the lymphatic system
Lymphatic vessels begin to develop during midgestation, shortly after blood circulation is established. Studies using in vivo imaging in zebrafish and lineage tracing in mice have confirmed that the majority of initial lymphatics originate from the cardinal vein and other primitive veins, as originally proposed by Florence Sabin over a century ago. LECs also emerge from alternative nonvenous sources via organ-specific mechanisms that appear to be conserved in mammals. Hemogenic endothelium contributes to mesenteric lymphatics, nonvenous cells derived from blood capillaries supplement dorsal skin lymphatics, , hematopoietic cell and second heart field-derived cells give rise to cardiac lymphatics, bone marrow–derived cells play a role in forming corneal lymphatics, and inflammation-associated macrophages incorporate into renal lymphatics. Lymphatic endothelial progenitors coalesce and expand to form a primitive lymphatic plexus that further develops and elaborates to form a defined, tree-like network of capillaries and collecting lymphatic vessels. , , Extensive postnatal lymphangiogenesis also occurs through sprouting of preexisting lymphatic vessels and by proliferation and migration of fully differentiated lymphatic ECs, as shown in both mice and zebrafish. , ,
Several important molecular pathways critical for lymphatic vessel development have been identified. The transcription factor, prospero homeobox 1 (PROX1), is required for lymphatic commitment and maintenance. Additional transcription factors including SRY-box transcription factor 18 (SOX18), Chicken ovalbumin upstream promoter transcription factor II (NR2F2 (also known as COUP-TFII)), and GATA binding protein 2 (GATA2) act upstream of and promote expression of PROX1. Prox1-positive progenitor cells bud from the endothelium of the cardinal vein and other primitive veins and begin to express vascular endothelial growth factor receptor 3 (FLT4 (also known as VEGFR3)), which allows the lymphatic progenitors to respond to vascular endothelial growth factor C (VEGFC) signals to migrate and form the primitive lymph sacs that gives rise to the lymphatic network. Several different cell types such as fibroblasts, neurons, endothelial cells, smooth muscle cells, and macrophages express VEGFC and can attract LECs and help guide their migration. , Loss of Vegfc or Vegfr3 results in lymphatic malformation, edema, and lethality, confirming the critical role of this ligand–receptor pair in lymphatic development. , , Collagen and calcium binding EGF domains 1 (CCBE1) and a disintegrin and metalloproteinase with thrombospondin motifs 3 (ADAMTS3) are required for proteolytic activation of VEGFC, and their loss also causes lymphatic defects. Chemokine signals such as the CXC motif chemokine receptor 4 (CXCR4) – CXC motif chemokine ligand 12 (CXCL12) axis provide critical guidance cues for lymphatic network assembly and patterning ( Fig. 1.2 ). , Notably, mutations in VEGFR3, VEGFC, CCBE1, and ADAMTS3 are found in human congenital lymphatic diseases including congenital lymphedema, Milroy disease, and Hennekam syndrome, reinforcing the importance of understanding more about the molecular mechanisms of lymphangiogenesis.
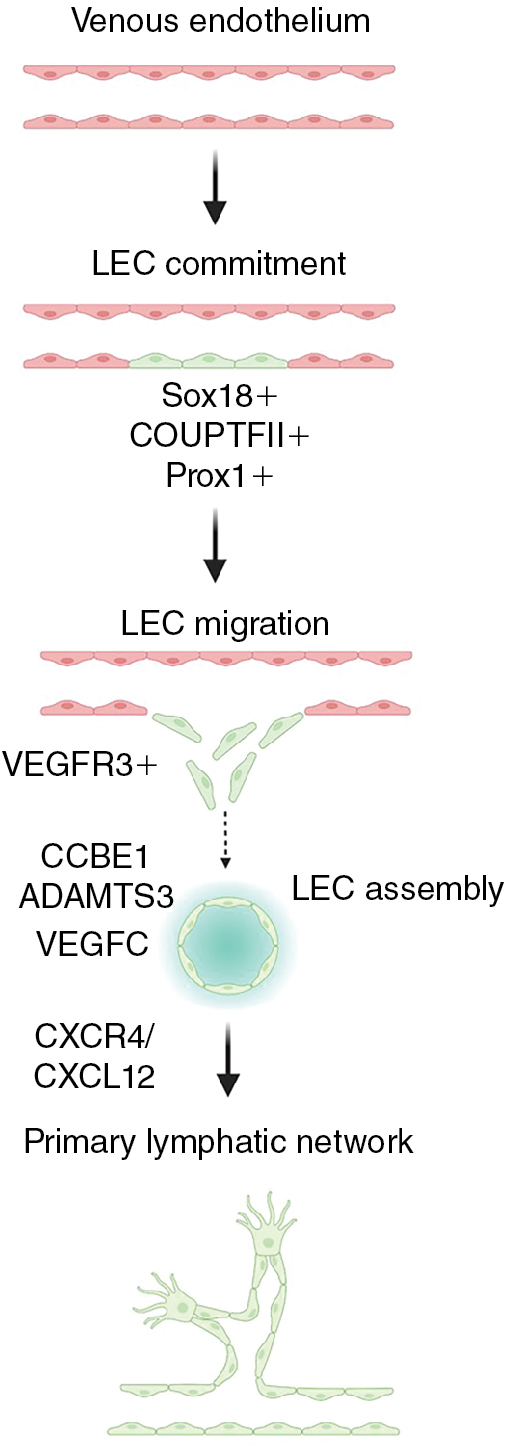
Animal models have been critical to our understanding of the development of the lymphatic system. The lymphatic vasculature is highly conserved throughout the vertebrates, including in aquatic animals. The zebrafish is a superb genetically and experimentally accessible vascular model organism that has already yielded many important discoveries regarding lymphatic vessel development. , , , , , , Studies in the fish have shown that a subset of venous endothelial cells bud from the posterior cardinal vein and form secondary sprouts that give rise to lymphatic progenitor cells on the horizontal myoseptum region, called parachordal lines or parachordal lymphangioblasts . , , This subset could be angioblasts from the ventral side of the posterior cardinal vein that migrates toward the dorsal side of the vein and gives rise to lymphatic sprouts. The venous cells committed to lymphatic fate acquire Vegfc-promoted Prox1 expression that regulates asymmetric cell division and helps direct their differentiation and migration to become lymphatic progenitors. As in mammals, Vegfc/Vegfr3 and cxcr4/cxcl12 signaling pathways are critical to guide migration and patterning of these progenitor cells in fish, , and zebrafish ccbe1 and adamts3 genes are essential for cleavage of precursor Vegfc, and their loss impairs lymphatic vessel development. , The extensive conservation of mechanisms of lymphatic development among the vertebrates permits genetically and experimentally accessible animal models such as the zebrafish to be used to experimentally probe molecular mechanisms and biologic processes required for lymphatic development and their links to human lymphatic diseases.
The primitive lymphatic vascular network further matures and remodels to develop a functional system. A hierarchical structure consisting of capillaries and precollecting and collecting lymphatics forms to ensure efficient unidirectional lymph transport. Blind-ended lymphatic capillaries are composed of oak-shaped LECs that overlap and connect via discontinuous button-like junctions. This unique shape and structure allow interstitial fluid to easily drain from the interstitial spaces of tissues into the lymphatic vessels and inhibits backflow out of lymphatic capillaries ( Fig. 1.3 A). , The downstream collecting lymphatics are more complex vessels that consist of continuous zipper-like junctions that prevent fluid leakage, and they are covered with basement membrane and a contractile smooth muscle layer that promotes transport of fluid toward the lymph node and eventually back to blood circulation. The presence of valves is an important additional feature of collecting lymphatics that helps prevent backflow (see Fig. 1.3 B). Strong expression of the forkhead box C2 (FOXC2) and nuclear factor of activated T cells 1 (NFATC1) transcription factors in a subset of LECs helps regulate lymphatic maturation and valve formation. , Animals with defective FOXC2 or NFATC1 display abnormal lymphatic vessel patterning, failed remodeling of lymph sacs, and deficient valve formation. , Lymph flow causing oscillatory shear stress is one of the factors that regulates FOXC2, whereas as discussed later, microRNA-204 (miR-204) posttranscriptionally regulates nfatc1. Interestingly, suppression of either FOXC2 or NFATC1 leads to lymphatic hyperplasia, suggesting that these factors also regulate LEC proliferation. , Another transcription factor important for lymphatic development is GATA2. Knockdown of Gata2 leads to overgrowth of lymphatics and defective lymphatic valve in mice and zebrafish. , Gata2 promotes Vegfr3 expression required for LEC migration and vessel patterning. LECs contributing to collecting lymphatic vessels also express secreted signals such as platelet derived growth factor subunit B (PDGFB) and reelin (RELN) that recruit smooth muscle cells to support these vessels. , Smooth muscle coverage of collecting lymphatic vessels is critical for lymphatic contractility. ,
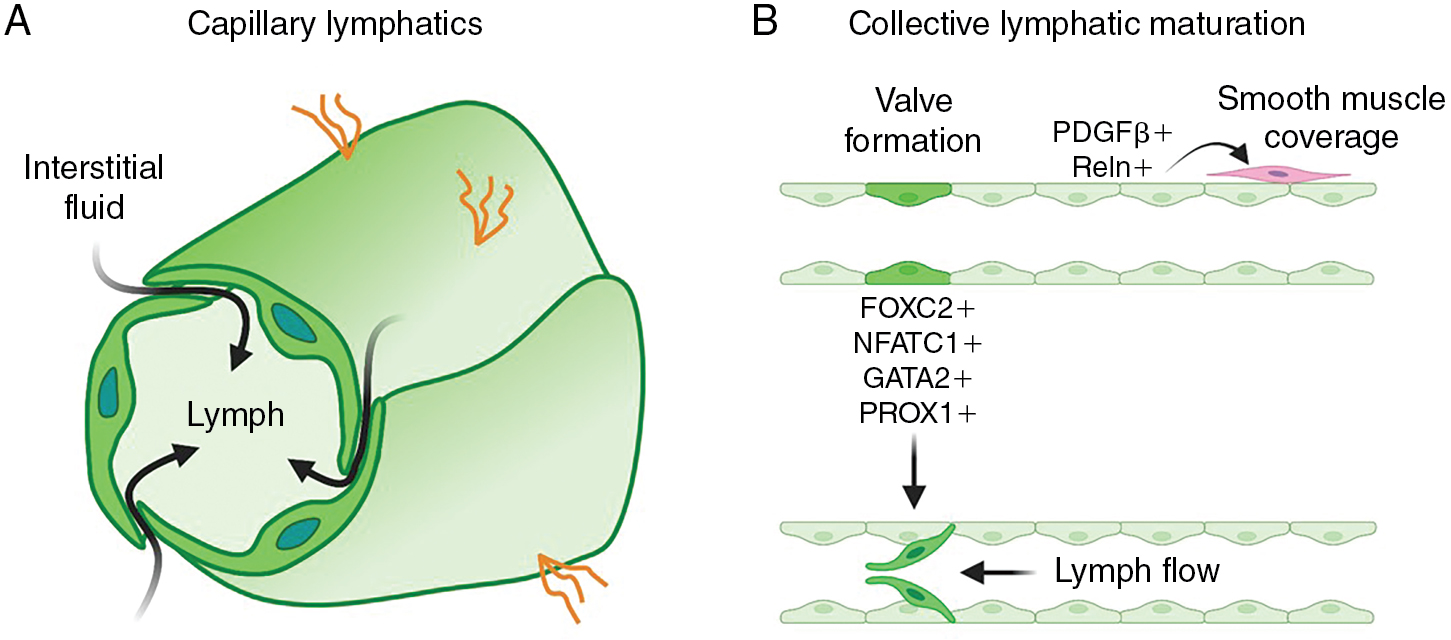
Research on the role of microRNAs in lymphatic biology has begun to uncover important functions for these factors in regulating lymphatic vessel development. Small RNA profiling of human blood and LECs led to identification of lymphatic microRNAs. , The expression of miR-204 is highly enriched in human and zebrafish LECs compared with blood vessel endothelial cells (BECs) and the miR-204-deficient animals fail to form lymphatic progenitors and the lymphatic vessel network. miR-204 targets NFATC1, and the lymphatic defects in miR-204-deficient animals can be reversed by manipulating nfatc1, suggesting that this regulatory axis is important for developmental lymphangiogenesis. In primary cultured rat mesenteric LECs, tumor necrosis factor (TNF) treatment induces miR-9 to regulate Nuclear factor kappa-light-chain-enhancer of activated B cells (NF-κB) level and suppress inflammation. MicroRNAs miR-181a and miR-31 bind to the 3′ untranslated region of PROX1 and negatively regulate LEC genes such as VEGFR3 and FOXC2 in blood endothelial cells to maintain their BEC identity. , Expression of some of these microRNAs is regulated by bone morphogenic protein (BMP) activity to help direct the lymphatic fate decision. MicroRNA-466 has also been shown to suppress Prox1, inhibiting lymphangiogenesis in a rat corneal model. The pan-endothelial miR-126 targets cxcl12a and vegfr3 signaling and directs LEC sprouting and extension. ,
Heterogeneity of lymphatic endothelial cells and organ-specific lymphatic vessels
Although LECs in diverse lymphatic vessels share many similarities, they are remarkably heterogeneous and plastic in different organs and in different physiologic and pathologic states. Although lymphatic capillaries and collecting lymphatics have distinct morphologic and functional characteristics (discussed in Embryonic development of the lymphatic system ), LECs on both vessels express PROX1, the master transcription factor of lymphatic differentiation and identity. They also both express other important lymphatic regulators such as podoplanin (PDPN), VEGFR3, , and neuropilin 2 (NRP2). , Although the organization of their cell–cell junctions varies, all lymphatic vessels also express similar sets of junctional proteins like VE-cadherin, claudin-5, and platelet and endothelial cell adhesion molecule 1 (PECAM-1). Although the LECs of lymphatic capillaries and collecting vessels have many similarities, they are also clearly distinguishable by molecular profiling. Notably, lymphatic capillaries express high levels of the lymphatic vessel endothelial hyaluronan receptor 1 (LYVE1) and the chemokine, C-C motif chemokine ligand 21 (CCL21). LYVE1 is used as a common lymphatic marker, although its molecular function is not well understood. LYVE1 is expressed in LEC progenitors and in primitive blood vessels during embryonic development, , , but mice deficient in Lyve1 are viable and develop macroscopically normal lymphatic vessels. , However, lymphatic capillaries with limited distensibility in organs such as the liver and intestine undergo morphologic changes that alter fluid uptake. , Lyve1 also plays a critical role in docking dentric cells to the basolateral surface of lymphatic vessels. CCL21 is a strong chemoattractant for C-C motif chemokine receptor 7 (CCR7)-positive immune cells, guiding their migration toward lymphatic vessels. , , Unlike lymphatic capillaries, collecting lymphatics express low levels of LYVE1 and CCL21 and, as a result, migration of immune cells to and through these vessels is thought to be passive. In contrast to capillary vessels that are specialized for fluid uptake and immune cell recruitment, collecting vessels are designed for transport (see Fig. 1.3 ). Collecting vessels also have luminal valves that are critical for unidirectional flow (see Fig. 1.1 ). These valves express high levels of transcription factors FOXC2, NFATC1, GATA2, and integrin subunit alpha 9 (ITGA9), which are required for their formation (see Fig. 1.3 B). , , ,
LECs respond to inflammatory stimuli and show remarkable plasticity in pathologic conditions. Inflamed peripheral lymphatics suppress migration of leukocytes by atypical chemokine receptor 2 (ACKR2) and inhibit dendritic cell maturation via altered expression of intercellular adhesion molecule 1 (ICAM1). Interestingly, LECs respond differently to some inflammatory stimuli and can influence dendritic cell migration in CCL21-, ICAM1-, and CCR7-independent fashion, suggesting that LECs are highly plastic and respond in a stimulus-specific manner as shown in the case of skin inflammation. The molecular profile changes observed in tumor-associated lymphatics further support the idea that LECs are highly plastic. These new findings add layers of complexity to understanding the plasticity of LECs in health and disease state including infection, inflammation, and cancer. Lymphatic vessels also acquire unique features and contribute to specialized functions according to the functional demands of each organ, guided by organ-specific cues. , We discuss a few well-characterized organ-specific lymphatic vascular beds later.
Intestinal lymphatic vessels play vital roles in lipid absorption, preservation of gut immunity and intestinal homeostasis. The intestinal lymphatics are composed of two unique vascular networks that connect to form the largest lymphatic bed in the human body. Lacteals are blind-ended lymphatic capillaries that drain excess fluid from the vascular bed within the intestinal villi. These lacteal lymphatic capillaries link back to the submucosal lymphatics and the muscular lymphatic network that drains the smooth muscle layer of the intestine. Both vascular networks connect to larger collecting vessels in the mesentery to transport fluid to mesenteric lymph nodes. Lacteals have distinct specialized functions in absorption of dietary fats (chylomicrons) and fat-soluble vitamins from the villi to be delivered directly to the circulation via the cisterna chyli and thoracic duct. Nevertheless, it is unclear exactly how chylomicron uptake occurs; it may involve paracellular transport across LEC junctions as well as transcellular transport in vesicles. Like dermal lymphatic capillaries, lacteals have high expression of LYVE1 and CCL21, and they provide an entry point for dendritic cells in the villi to access draining mesenteric lymph nodes. This serves as an essential mechanism of gut immunosurveillance and oral tolerance. , However, unlike most lymphatic capillaries, lacteals have a mixture of both discontinuous button-like and continuous zipper-like cell–cell junctions. In addition, lacteal LECs continuously proliferate at a slow rate under homeostasis. Postnatal deletion of VEGFC resulted in lacteal regression and diminished lipid uptake with no effects on the integrity of quiescent adult dermal lymphatics. This regenerative mechanism may be important in lacteals to manage the constant cellular stress from exposure to dietary products. Although lacteals play a primary role in uptake of dietary fat, lymphatic vessels outside the gastrointestinal tract can also affect fat metabolism. This is evident in patients suffering from chronic lymphedema, who frequently present with accumulation of adipose tissue in affected body parts. Dysfunction in lymph transport can also result in chylous effusions in the abdominal cavity (ascites) and thoracic cavity (chylothorax) and may contribute to cholesterol-associated conditions like atherosclerosis. ,
In the brain, fluid homeostasis and clearance of waste and cellular debris are maintained by the glymphatic system named after its dependence on glial cells and comparable draining functions to lymphatics. , , The lymphatic vascular network in the dural meningeal layers surrounding the brain has led to a new understanding of central nervous system (CNS) lymphatics. , , , Meningeal lymphatics function as drainage channels for clearance of cerebrospinal and interstitial fluid macromolecules. They also provide paths for lymphocytes and dendritic cell trafficking from the cerebrospinal fluid (CSF), through deep cervical lymph nodes, to the peripheral lymphatic system. Ligation of the meningeal lymphatic vessels results in accumulation of immune cells within the meninges. , Phenotypically, meningeal lymphatic vessels express classic LEC markers like PROX1, VEGFR3, LYVE1, and CCL21. Unlike lymphatic vessels in other organs, meningeal lymphatic vessels develop postnatally in response to VEGFC signaling in the blood vasculature. In mice, inhibition of VEGFC signaling hinders meningeal lymphatic vessel development and overexpression of VEGFC promotes excess meningeal lymphangiogenesis, highlighting the remarkable plasticity and regenerative potential of the meningeal lymphatics. Meningeal lymphatic vessels (mLVs) consist of dorsal mLVs and basal mLVs. Interestingly, although dorsal mLVs have similar features as capillary LVs, dorsal mLVs have continuously sealed zipper-like junctions, whereas basal mLVs have discontinuous sealed loss button-like junctions. Interestingly, basal mLVs display a precollecting vessel phenotype characterized by lymphatic valves but no smooth muscle cells (SMC). These distinct features promote CSF and interstitial fluid (ISF) uptake and maintenance of unidirectional lymph flow. , , In addition, the close anatomic proximity of the basal mLVs to the subarachnoid space suggests that basal mLVs are hotspots for fluid drainage into the lymphatic system. Meningeal lymphatics are highly conserved including in lower vertebrates such as the zebrafish, where high-resolution optical imaging of lymphatics in living animals can be used to understand its function in brain homeostasis. A better understanding of mechanisms regulating the meningeal lymphatic system may have clinical implications in neurodegenerative diseases (e.g., Alzheimer disease), autoimmune neuroinflammatory conditions (e.g., multiple sclerosis), and cancer.
There are also some hybrid vascular beds that share lymphatic and blood vessel properties. The Schlemm canal (SC) is an endothelium-lined channel that creates a vascular route for the drainage of the aqueous humor within the anterior eye chamber. , This structure is regarded as an intermediate vessel type between lymphatic and blood vessels (hybrid) but has functional and molecular similarities closer to those of lymph vessels. , SC endothelial cells express typical lymphatic markers like PROX1, VEGFR3, CCL21 and are responsive to VEGFC-induced angiogenesis, although they lack expression of LYVE1 and podoplanin. In addition, they express TEK receptor tyrosine kinase (also known as TIE2) and endomucin, which are generally blood endothelial cell markers. , , During postnatal development, the SC sprouts from the choroidal veins in a manner analogous to the formation of primitive embryonic lymph sacs from Prox1+ endothelial cells in the cardinal veins. The VEGFC/VEGFR3 and angiopoietin/TIE2 signaling pathways have also been reported to be vital in the formation and differentiation of SCs and maintenance of SC integrity. , SC dysfunction decreases drainage of aqueous humor, which leads to increased intraocular pressure, ultimately resulting in glaucoma. Genetic deletion of angiopoietin 1 and 2 (TIE2 ligands) during late embryogenesis in mice resulted in loss of SC and development of glaucoma in early life. Loss of function mutations in TIE2 and angiopoietins have also been observed in human patients with congenital glaucoma. , The ascending vasa recta (AVR) of the kidney provides another example of a hybrid vascular bed. The AVR is a vascular network that drains large volumes of interstitial fluid from the renal medulla. AVR endothelial cells express blood endothelial cell markers (endomucin, CD34, CD31) and some LEC markers (PROX1 and VEGFR3) but not LYVE1 and podoplanin. High expression of PROX1 and VEGFR3 and abundant fenestration in the AVR may help facilitate its functional roles in fluid re-uptake in the kidney.
Taken together, LEC plasticity and heterogeneity are likely to play in the specialized functions of lymphatic and lymphatic-like vessels in different tissues and organs.
Lymph node microscopic structure and function
Lymph nodes are soft, lumpy specialized structures that are closely integrated with the lymphatics, serving as filters to prevent reentry of harmful stimuli into circulation and as key immune surveillance centers. These highly organized structures have different cellular compartments that play crucial roles for mounting effective immune responses , ( Fig. 1.4 A). The lymph node contains multiple lymphoid lobules surrounded by a sinus system filled with lymph enclosed in a collagenous fibrous capsule. The lobules are covered in a dense reticular meshwork that provides a three-dimensional (3D) scaffold with gaps for cellular interactions. The lymph node is composed mainly of leukocytes that account for approximately 95% of cells. Other stromal cell types within the lymph node include BECs, LECs, and fibroblastic reticular cells, which all contribute to adequate organ function. , The number and structural organization of lymph nodes vary between species. Generally, lymph nodes become more abundant and complex as species size increases. For example, mice have approximately 22 lymph nodes arranged in simple chains, whereas humans have about 450 lymph nodes organized in more complex chains. Larger species also have greater anastomoses of afferent lymphatics compared with smaller species. ,
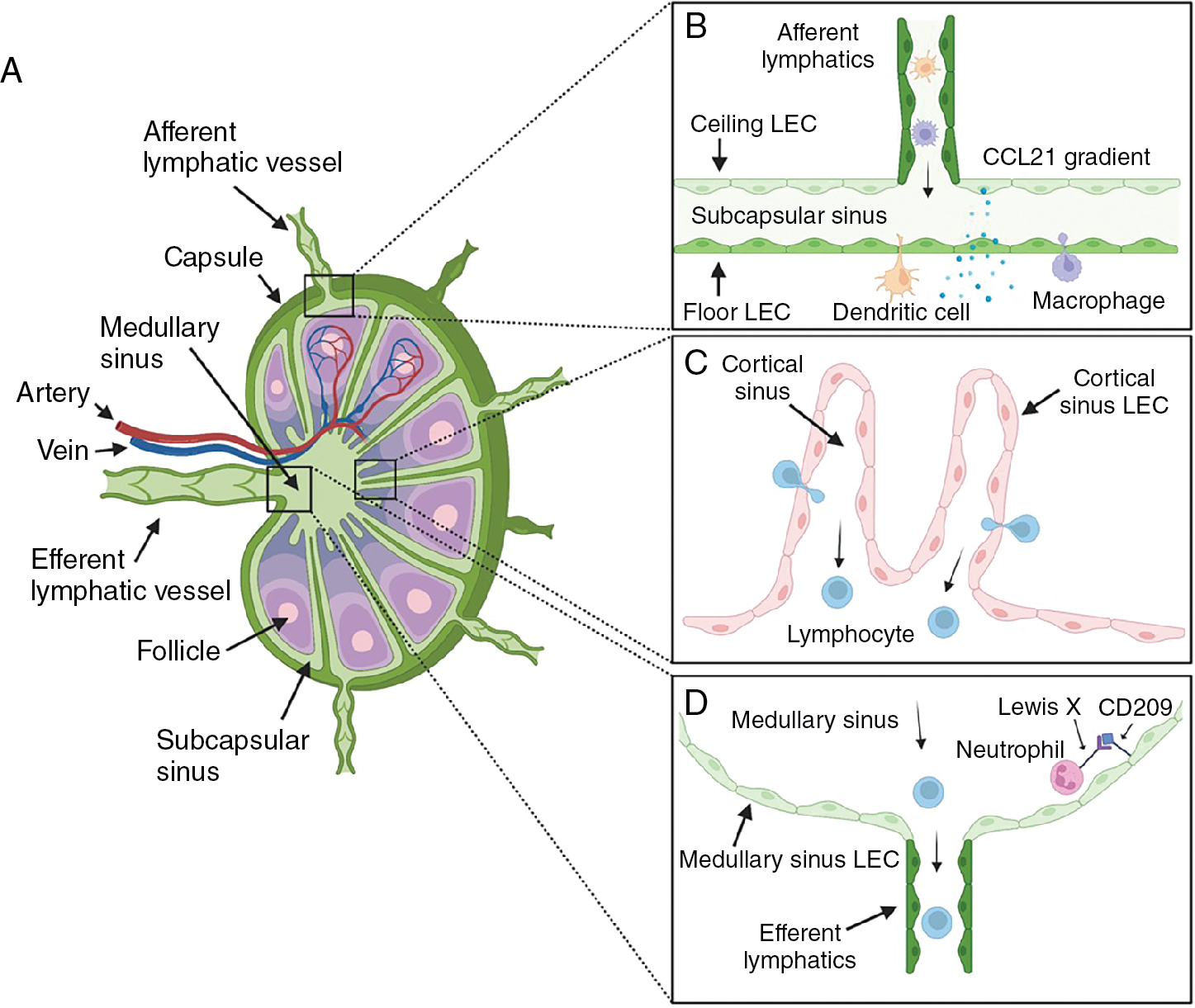
Lymph nodes receive blood supply through high endothelial venules that facilitate entry of blood antigens, naïve cells, and memory cells. Lymph carrying antigen-presenting cells (APCs), tissue-derived antigens, and immune cells enters the node through the afferent lymphatic vessels (see Fig. 1.4 B). By providing an avenue for antigens, APCs, and lymphocytes to converge, the lymph node facilitates effective immune surveillance and activation of adaptive immunity. Upon entering the lymph node, APCs and lymphocytes migrate to distinct subcompartments within the parenchyma. T lymphocytes and dendritic cells traffic to paracortical T-cell areas, whereas B lymphocytes home to the follicles in the cortex (see Fig. 1.4 C). This is modulated by stromal cell networks and stromal cell–derived chemokines (CCL21, CCL19, and CXCL13), which play crucial roles in directing immune cells to their respective compartments. Within the reticular meshwork, APCs present antigens to lymphocytes, which become activated and undergo clonal expansion to generate new antigen-specific lymphocytes and plasma cells to initiate an immune response.
After entering the lymph node, afferent lymphatic vessels form branched sinus systems. The basic structure of the lymph node sinus system is conserved across mammalian species. , Much of our current knowledge about the sinus system was acquired from studies in rodent models. However, human lymph node sinuses differ significantly in structure as a result of the presence of trabeculae and trabecular lymphatic sinuses that are absent in rodents. In general, the afferent lymphatics penetrate the lymph node capsule to open into a subcapsular sinus (SCS) with multiple afferent vessels entering at different positions (see Fig. 1.4 A). The SCS is lined by LECs on both sides that cover the entire cortex. LECs facing the capsule are termed ceiling LECs and those that border the lymph node parenchyma are known as floor LECs (see Fig. 1.4 B). Within the sinus lumen, there are both migratory leukocytes of the afferent lymph and tissue-resident leukocytes. SCS macrophages and sinus-resident dendritic cells are associated with floor LECs and have cellular projections that maintain surveillance of the sinus lumen. The SCS macrophages trap large-sized particles, molecules, and microbes in the lymph and present antigens to naïve/memory B and T cells migrating to the follicles and T-cell areas, respectively. , During infection or inflammation, SCS macrophages can reactivate memory B cells to differentiate into plasma cells in the subcapsular proliferative foci (SPF)—a site of rapid plasma cell differentiation. Small molecules (<70 kDa), however, can penetrate directly through lymph node conduits to reach the B-cell follicles and T-cell areas.
The SCS connects directly to the medullary sinus at the lymph node margin. Although, there is no clear demarcation point between both major sinuses, the ceiling LECs in the medulla have a distinct phenotype. In all mammals, the medullary sinuses converge into a single efferent lymphatic vessel that transports lymph out of the lymph node (see Fig. 1.4 D). Medullary sinuses contain slow-flowing lymphocytes emerging from the lymph node and native intralumenal medullary sinus macrophages. Other sinus systems include the transverse and cortical sinuses.
Transverse sinuses are deep radial invaginations of SCS situated in the lymph node fibrous septae. They are present in humans, rats, and other large species but not in mice. Transverse sinuses form shortcut conduits from the SCS to the medullary sinus to enhance subcompartmentalization of the lymph node. Cortical sinuses, on the other hand, are blind-ended sacs that appear at the paracortical zone and drain into the medullary sinuses. They form a compact network of lymphatics through which egressing lymphocytes exit the lymph node parenchyma.
Lymph node organogenesis occurs with a massive LEC remodeling, which starts at embryonic day 12 (E12) in mice as a cup-like projection around the anlage (the future lymph node area). Embryonic LECs serve as a primary lymphoid tissue organizer (LTo) and attract hematopoietic lymphoid tissue-inducer (LTi) to the anlage. At E15.6–E16.5, LECs from collective lymphatics underneath the lymph node anlage remodel and form a disc-like structure, which is subsequently engulfed by a cup-like LEC sheet at E20.5. Around E16.5, the outer and inner layers of LEC sheets and sinusoidal cords form. At this stage, the outer layer (ceiling LECs) and inner layer (floor LEC) express unique gene expression in adult lymph nodes, suggesting the LEC heterogeneity is already established during embryonic development. Lymph node LECs also secrete growth factors like PDGFβ to recruit SMCs, which is required for capsule formation.
Several studies have suggested the presence of heterogenous populations of LECs in the lymph node. , , In mice, LECs lining the SCS express macrophage scavenger receptor 1 (MSR1), whereas cortical sinus LECs express endomucin. Ceiling LECs have also been reported to differentially express atypical chemokine receptor CCRL1, which maintains CCL21 chemokine gradient in the murine SCS. Human LECs, however, lack expression of the general lymphatic marker LYVE1 in the SCS. A single-cell survey characterizing LEC heterogeneity identified six transcriptionally distinct LEC subtypes located in distinct anatomic locations in the human lymph node. , Floor LECs in the SCS and medullary sinus constitutively express neutrophil chemoattractants. Interestingly, selective expression of C-type lectin CD209 on medullary sinus LECs facilitates neutrophil adhesion via CD209-Lewis X binding, thus mediating neutrophil recruitment into the medullary sinuses. Overall, LEC heterogeneity in the lymph node creates a unique chemokine gradient and provides important niche for immunologic events.
The lymphatic system and cancer
Tumor vasculature consists of leaky vessels and the network is formed in nonhierarchical fashion where cancer cells invade into the bloodstream ( Fig. 1.5 A). In addition to leaky blood vessels, lymphatic vessels are present in the tumor microenvironment, and they play an active role in tumor progression and metastatic spread (see Fig. 1.5 A). , , The dissemination of tumor cells from primary tumors to distant organs via circulation or lymphatics is a key characteristic of malignant cancer progression. Several studies have shown that increased lymphatic vessel invasion increases the risk of lymph node and distant metastasis and is associated with poor patient prognosis. Peritumoral lymphatic vessels take up cells and macromolecules from tumors and transport them to sentinel lymph nodes. As a result, tumor-associated lymphatic vessels serve as an important route for metastatic cancer cells to spread from the primary tumor to migrate to regional lymph nodes (see Fig. 1.5 B).
