Delivering Anticancer Drugs to Brain Tumors
Maciej M. Mrugala
Jeffrey G. Supko
Tracy T. Batchelor
There were an estimated 51,140 new primary brain tumors diagnosed in the United States in 2007.1 Malignant gliomas (anaplastic astrocytoma and glioblastoma) are the most common malignant primary brain tumors and represent the most frequent indication for cytotoxic chemotherapy in neuro-oncology. The goal of adjuvant chemotherapy for malignant glioma is eradication of the residual macroscopic and microscopic tumor felt to be the reason for surgical and radiation failure. Temozolomide (TMZ), an orally administered alkylating agent, significantly extends progression-free and overall survival when administered concurrently with radiation in patients with newly diagnosed glioblastoma.2 This positive effect is particularly pronounced in patients with methylated O6-methylguanine-DNA methyltransferase (MGMT) promoter and can be maintained for a prolonged period of time.3,4 In addition, locally delivered chemotherapy in the form of 1,3-bis(2-chloroethyl)-1-nitrosourea (carmustine; BCNU) polymers also extends survival slightly in patients with malignant glioma when applied at the time of the initial debulking procedure.5 A humanized monoclonal antibody against the vascular endothelial growth factor (VEGF) ligand, bevacizumab, extends progression-free survival in recurrent glioblastoma patients relative to historical controls when given alone or in combination with irinotecan.6,7 Bevacizumab was approved as monotherapy for recurrent glioblastoma by the United States Food and Drug Administration in 2009. However, the survival benefit of adjuvant chemotherapy for patients with malignant gliomas is modest as demonstrated by an absolute increase in one-year survival of 6% in one meta-analysis of 12 randomized clinical trials.8
Mechanisms of chemotherapy resistance of brain tumors include factors common to other tumors such as multidrug resistance and increased efficiency of DNA damage repair systems.9 In addition, treating malignant brain tumors represents a unique challenge for oncologists due to the presence of the blood-brain barrier (BBB), a physiologic impediment between the circulatory system of the brain and that of the body. The accessibility of many anticancer drugs to brain tumors is at least partially constrained by the BBB. Therefore, difficulty in achieving adequate and sufficiently sustained levels of the cytotoxic moiety at the tumor site is a significant factor contributing to the failure of systemic chemotherapy for malignant brain tumors.10,11 Accordingly, the development of treatment strategies for brain tumors has emphasized techniques that are intended to overcome this barrier and improve drug delivery to these tumors. In addition, the multiplicity of ancillary agents used in the medical management of brain tumor patients, particularly glucocorticoids and enzyme-inducing antiseizure medications, increases the risk for drug interactions that may impact the efficacy or toxicity of chemotherapy. This chapter reviews the current state of approaches for delivering anticancer drugs to brain tumors, the various techniques that are available for assessing drug distribution to brain tumors, and important pharmacologic interactions that may affect both the accessibility of anticancer drugs to the CNS and the systemic pharmacokinetics of the anticancer agent.
Blood-Brain Barrier
Three main factors influence the extent to which a systemically administered anticancer agent distributes into the brain and brain tumors: (a) the plasma concentration-time profile of the drug; (b) regional blood flow; and (c) transport of the agent through the BBB and blood tumor barrier (BTB). The two former considerations are common to all solid tumors, whereas the latter is specific to brain tumors.10 Erhlich was the first to propose the concept of the BBB at the beginning of this century. On administering the dye Trypan blue to rats by intravenous injection, he observed that all body organs were stained except for the brain and spinal cord.12 The anatomic basis of the BBB was determined three decades ago with the introduction of the electron microscope. It results from a modification of the normal vascular endothelium whereby a sheet of cells is connected by tight junctions on a basement membrane (Fig. 5-1). The area of the exchange surface is 12 m2 and the physiologic role of the BBB is assumed to include maintenance of a constant biochemical content of the interstitial milieu and protection of the brain from foreign and undesirable molecules.13 Low hydraulic conductance, low ionic permeability, and high electrical resistance contribute to the very low permeability for hydrophilic non-electrolytes in the absence of a membrane carrier.14 These properties, together with the lack of intracellular fenestrations and pinocytotic vesicles, and the presence of a thicker basal lamina, create a physiologic barrier that is relatively impermeable to many water-soluble compounds.15,16
Some drugs use specific transport mechanisms present in the endothelial cell to traverse the BBB.17 However, most cytotoxic drugs that gain access to the CNS, as exemplified by the chloroethylnitrosourea alkylating agents, cross the BBB by passive diffusion. Aside from pharmacokinetic properties, the main factors that influence the extent to which these compounds distribute into the CNS include lipid solubility, molecular mass, charge, and plasma protein binding. Specifically, small organic compounds with a molecular weight less than 200 that are lipid soluble, neutral at physiologic pH, and not highly bound to plasma proteins readily cross the BBB.14
A second component of the BBB is the expression of P-glycoprotein (Pgp) on the luminal surface of brain capillary endothelial cells.18 The presence of P-glycoprotein has been implicated in the active efflux of many chemotherapeutic drugs from the brain, including the vinca alkaloids and doxorubicin. Expression of P-glycoprotein has also been reported in malignant gliomas and may serve as another mechanism of chemotherapy resistance.19,20 Multidrug resistance-related protein 1 (MRP1) and P-glycoprotein are differentially expressed in high- and low-grade gliomas. MRP1 overexpression appears to be more common in high-grade gliomas (WHO grade IV) while low-grade tumors (WHO grade II) exhibit greater Pgp expression. These findings may have potential therapeutic implications.21 Agents that reverse the function of P-glycoprotein, such as verapamil, may increase passage of doxorubicin across the BBB.
The normal physiologic structure of the BBB is disrupted in vasculature within and adjacent to brain tumors. The barrier is usually more permeable in the center of a malignant tumor as opposed to the well vascularized and infiltrating edge that exhibits variable degrees of BBB integrity.22 Figure 5-2 contrasts the normal BBB with the disrupted barrier found in a brain tumor. Vick and colleagues identified junctional clefts in the endothelial cells of capillaries adjacent to brain tumors.23 These clefts correlated with the density of infiltrating tumor cells and were present in brain capillaries not in direct contact with the tumor. Evidence also exists that the microvasculature of these tumors lacks the properties of a normal BBB and has greater permeability as a result. Morphologic studies have demonstrated that the BTB differs anatomically from the normal BBB, with open tight junctions, gap junctions, fenestrations, and numerous intracellular vesicles.10,24 The increased permeability of these blood vessels forms the basis of contrast enhancement of brain tumors on CT and MRI scans. Iodinated, water-soluble
contrast agents do not penetrate areas of the brain with an intact BBB but are able to penetrate brain tumors.25 These alterations in permeability are highly variable between tumors and within the same tumor.26 For example, low-grade gliomas and proliferating edges of malignant gliomas seem to have a normally functioning, selective BBB and, consequently, do not typically show contrast enhancement on CT and MRI studies. A large variation in the enhancement patterns of malignant brain tumors on CT scans is common.27,28 Approximately 30% of patients with anaplastic astrocytoma, a type of malignant glioma, are reported to have nonenhancing lesions.27 A novel MRI technology for assessment of cerebral blood flow, cerebral blood volume and blood-brain-barrier leakage was recently developed and refined. Both dynamic susceptability contrast MRI (DSC-MRI) and T1-weighted dynamic contrast-enhanced MRI (DCE-MRI) allow for precise measurement of these paramenters. In additon, DCE-MRI can estimate the degree of BBB leakage.29 Finally, positron emission tomography (PET) studies have shown that alterations in permeability usually occur in the central part of large tumors, whereas the periphery is intact.30 The presence of a selective, normal BBB near the proliferating edge of a brain tumor may result in variable delivery of water-soluble drugs in this region and may contribute significantly to the high local failure rate of conventional anticancer drugs.
contrast agents do not penetrate areas of the brain with an intact BBB but are able to penetrate brain tumors.25 These alterations in permeability are highly variable between tumors and within the same tumor.26 For example, low-grade gliomas and proliferating edges of malignant gliomas seem to have a normally functioning, selective BBB and, consequently, do not typically show contrast enhancement on CT and MRI studies. A large variation in the enhancement patterns of malignant brain tumors on CT scans is common.27,28 Approximately 30% of patients with anaplastic astrocytoma, a type of malignant glioma, are reported to have nonenhancing lesions.27 A novel MRI technology for assessment of cerebral blood flow, cerebral blood volume and blood-brain-barrier leakage was recently developed and refined. Both dynamic susceptability contrast MRI (DSC-MRI) and T1-weighted dynamic contrast-enhanced MRI (DCE-MRI) allow for precise measurement of these paramenters. In additon, DCE-MRI can estimate the degree of BBB leakage.29 Finally, positron emission tomography (PET) studies have shown that alterations in permeability usually occur in the central part of large tumors, whereas the periphery is intact.30 The presence of a selective, normal BBB near the proliferating edge of a brain tumor may result in variable delivery of water-soluble drugs in this region and may contribute significantly to the high local failure rate of conventional anticancer drugs.
The central role hypothesized for the BBB in the resistance of brain tumors to chemotherapy has been questioned by some authorities. It has been suggested that because the BBB adjacent to tumors and the BTB lack the normal properties of an intact BBB, drug delivery to these areas should not be compromised.23 Indirect support for this argument comes from the observation that brain tumors occasionally respond to cytotoxic drugs that would not be expected to cross the BBB due to their physicochemical properties. A more consistent observation, however, has been that the most effective classes of antineoplastic drugs against malignant brain tumors are lipid-soluble molecules that can easily penetrate an intact BBB. Moreover, in addition to the existence of normal BBB at the proliferating edge of brain tumors, PET studies have demonstrated that, whereas the BBB and BTB may be abnormal at the time of diagnosis, these structures may become normalized on subsequent treatment.31 These latter observations support a pivotal role for the BBB and BTB in the resistance of brain tumors to systemically administered chemotherapy. Novel anti-VEGF (vascular endothelial growth factor) agents were recently introduced to glioma therapy.32,33 Through their mechanism of action these agents influence both BBB and BTB and can produce striking imaging results, frequently leading to either partial or complete disappearance of the contrast-enhancing tumors from MRI scans (Fig. 5-3).
Vascular Normalization
The vascular network formed by high-grade brain tumors is abnormal and is characterized by dilated vessels, increased permeabity, and abnormally thickened basement membranes. These morphological changes are associated with the formation of vasogenic brain edema and contribute to the hypoxic tumor microenvironment and increased interstitial fluid pressure (IFP).34 Vasogenic brain
edema is a cause of significant neurological morbidity in the brain tumor patient population and may decrease efficacy of cytotoxic therapies. Corticosteroids, also known to downregulate VEGF,35 have been used as the primary therapy for brain edema for over 50 years. However, these agents cause significant toxicity in brain tumor patients. Anti-VEGF agents, by reducing vessel permeability and “normalizing” the brain tumor vascular network, may represent a new class of agents with antiedema properties. According to the vascular normalization hypothesis, the morphological and physiological effects of anti-VEGF agents on the tumor vasculature may also improve the efficacy of cytotoxic therapies by reducing the hypoxic fraction within the tumor and by improving delivery of therapeutic agents into the tumor mass36 (Fig. 5-4). Preclinical and clinical observations have demonstrated that reduction in tumor hypoxia, improved delivery of therapeutics, and enhanced efficacy, all features consistent with the vascular normalization hypothesis, can be achieved with anti-VEGF therapy.37, 38, 39
edema is a cause of significant neurological morbidity in the brain tumor patient population and may decrease efficacy of cytotoxic therapies. Corticosteroids, also known to downregulate VEGF,35 have been used as the primary therapy for brain edema for over 50 years. However, these agents cause significant toxicity in brain tumor patients. Anti-VEGF agents, by reducing vessel permeability and “normalizing” the brain tumor vascular network, may represent a new class of agents with antiedema properties. According to the vascular normalization hypothesis, the morphological and physiological effects of anti-VEGF agents on the tumor vasculature may also improve the efficacy of cytotoxic therapies by reducing the hypoxic fraction within the tumor and by improving delivery of therapeutic agents into the tumor mass36 (Fig. 5-4). Preclinical and clinical observations have demonstrated that reduction in tumor hypoxia, improved delivery of therapeutics, and enhanced efficacy, all features consistent with the vascular normalization hypothesis, can be achieved with anti-VEGF therapy.37, 38, 39
![]() FIGURE 5-4 Schematic diagram to illustrate the process of vascular normalization. A. In healthy organs, there is a balance of proangiogenic and antiangiogenic molecules, which maintains an organized and efficient vascular supply. B. Tumors produce proangiogenic factors (various shades of green) that induce an abnormal, inefficient vascular network. C. Judiciously administered anti-VEGF therapy can restore the balance of proangiogenic and antiangiogenic signals and normalize the vascular network, potentially improving drug delivery and efficacy. D. However, if anti-VEGF therapy is continued, it can destroy the network totally, impeding delivery of oxygen and nutrients, and ultimately depriving the tumor of essential molecules for growth. In preclinical models, panel C usually progresses toward panel D (single arrow) with currently approved anti-VEGF agents. However, in human tumors, panel C commonly reverts to panel B after a “window of normalization” (double arrow). Anti, antiangiogenic molecules; IFP, interstitial fluid pressure; pO2, tissue oxygen level; Pro, proangiogenic molecules. (From Jain RK, et al. Angiogenesis in brain tumours. Nat Rev Neurosci 2007;8(8):610-622, with permission.) (Ref. 194.) |
Drug Delivery Methods
Following the administration of an anticancer drug by the intravenous or oral routes, the BBB can effectively impede the distribution of drug molecules from systemic circulation into the CNS. Consequently, considerable effort has been expended to develop drug delivery strategies that either entirely circumvent the BBB or modulate the permeability of the barrier to enhance the extent drug distribution into the brain from systemic circulation. These techniques include intra-arterial administration, BBB disruption with hyperosmolar solutions40 or biomolecules, high-dose chemotherapy (HDCT), intrathecal (IT) injection, and local delivery by direct intratumoral injection of free drug or implantation of drug embedded in a controlled-release biodegradable delivery system. Even when drugs have crossed the BBB, however, their migration to tumor cells may be hindered by increased intercapillary distances, greater interstitial pressure, lower microvascular pressure, and the sink effect exerted by normal brain tissue.41
Intra-arterial Administration
The theoretical advantage for delivering anticancer drugs by the intra-arterial route is related to the ratio of systemic to regional blood flow. In comparison to intravenous injection, a considerably higher local drug concentration can be achieved with intra-arterial injection, thereby increasing the amount of the agent driven across the BBB. This has been confirmed experimentally, there was a two- to threefold increase in tumor concentration of cisplatin and a chloroethylnitrosourea, respectively, in the brains of animals after intra-arterial administration as compared to intravenous administration.42 With this technique, sufficient local drug concentrations can be achieved with smaller than conventional doses so that systemic side effects are minimized.43 The pharmacokinetic advantages of intra-arterial administration occur only during the first passage
through the CNS, because the drug then enters venous circulation and the plasma profile is indistinguishable from that afforded by intravenous administration.
through the CNS, because the drug then enters venous circulation and the plasma profile is indistinguishable from that afforded by intravenous administration.
This approach has been clinically evaluated in various settings, including neoadjuvant and adjuvant therapy and recurrent malignant glioma.44,45 Thus far, clinical trials of intra-arterial chemotherapy for malignant gliomas have not demonstrated improvement in survival over conventional intravenous therapy. A phase III study involving 315 patients with malignant gliomas failed to show any advantages of adjuvant intra-arterial BCNU over intravenous infusion of the same drug.46 Moreover, subjects in the intra-arterial BCNU arm of this trial experienced significant treatment-related toxicities. 10% developed leukoencephalopathy and 15% developing ipsilateral blindness. Two randomized clinical trials compared intra-arterial and intravenous ACNU (nimustine). There was no significant difference in the progression-free survival and overall survival in each treatment arm. However, toxicity associated with intra-arterial ACNU was modest. No cases of leukoencephalopathy and only one case of transient visual impairment were reported.47,48
Potential disadvantages of the intra-arterial route include local complications related to catheterization (thrombosis, bleeding, infection) and neurological sequela (orbital and cranial pain, retinal toxicity, leukoencephalopathy, or cortical necrosis).44,46,49 In addition, prodrugs requiring hepatic activation, such as cyclophosphamide, procarbazine and irinotecan, are not suitable for use by the intra-arterial route. One factor that partly explains the unique toxicities associated with intra-arterial administration is the “streaming” effect.50 Infusion of drug into the high pressure, rapidly moving arterial bloodstream results in incomplete mixing of the drug and plasma and great variability in the amount of drug reaching different regions of the vascular territory. Depending on the characteristics of the distribution pattern, higher concentrations of drug might be achieved in normal brain, whereas lower amounts reach the tumor. Different strategies have been attempted to minimize the streaming effect, including rapid infusion,50 superselective cannulation of the feeding artery,49,51 diastole-phased pulsatile infusion,52,53 and local blood flow adjusted dosage.51 All of these techniques were combined in a phase I trial involving 21 brain tumor patients treated with intra-arterial carboplatin. The neurologic side effects were minor, and a two-fold escalation of the dose beyond the conventional intra-arterial dose was achieved with promising results.51 Despite its serious limitations, there have been a few reports of promising results in patients receiving intra-arterial therapy for primary brain tumors. Intra-arterial carboplatin and etoposide were demonstrated to be safe and useful in the treatment of progressive optic-hypothalamic gliomas in children.54 Safety and efficacy of intra-arterial chemotherapy with multiple agents in conjunction with osmotic disruption of the BBB were established.55,56 It seems that intra-arterial chemotherapy, with all its current limitations, is slowly moving forward and may achieve wider applications. Perfection of the delivery techniques and development of newer less toxic compounds should increase its efficacy and safety.
Blood-Brain Barrier Disruption
BBB disruption involves the use of hyperosmolar solutions or biomolecules to increase the permeability of the BBB and improve drug delivery to brain tumors. The specific mechanisms underlying osmotic opening of the BBB and BTB are not entirely understood. Preliminary explanations suggested that a hyperosmotic environment caused endothelial cell shrinkage with resultant separation of tight junctions.57 In addition to cellular shrinkage, osmotic stress releases biologically active molecules from endothelial cells, including serine proteases, that could potentially degrade the collagen matrix of the endothelial basement membrane. Finally, cellular shrinkage may also trigger second messenger signals and calcium influx, which could affect the integrity of tight junctions.13
Methods for disrupting the BBB involving both intravenous and intra-arterial administration have been developed for use in brain tumor patients.57 The results of nonrandomized studies have been encouraging for certain brain tumor subtypes, especially primary CNS lymphoma.58 Potential advantages of this method include increased tumor delivery of drug and lack of systemic toxicity from cytotoxic chemotherapy. Another possible advantage of this technique is avoiding the sink effect seen with other procedures used for delivering chemotherapy to brain tumors. The sink effect refers to the selective achievement of higher concentrations of a drug in areas of disrupted BBB in the tumor than to the rest of the brain. As a result of this concentration gradient, the drug rapidly diffuses out of the tumor into the surrounding brain and compromises tumor exposure time. Because BBB disruption theoretically affects the endothelium of both normal brain and brain tumor, a nonselective increase in drug delivery into both areas occurs, and no concentration gradient is established. Despite these potential advantages, the technique of BBB disruption is complex and requires transfemoral angiography and general anesthesia. Moreover, an attendant risk of stroke and a high frequency of seizures are associated with this method. These factors have limited the application of this technique.57,59
Tumor location and vascular supply determine the arterial circulation that is catheterized and infused. Most commonly, one major artery (left carotid, right carotid, left or right vertebral) is cannulated and treated. Some have advocated that documentation of BBB disruption with iodinated contrast agents be obtained before chemotherapy administration. Given the technical requirements of this procedure, it has not been widely adopted, and no definitive conclusions about the efficacy of the technique can be derived from the results of clinical trials that have been published to date. Other, less invasive techniques of BBB disruption are being investigated. It was recently shown that focused ultrasound exposure, when applied in the presence of preformed gas bubbles, can cause MRI-proven reversible opening of BBB in rabbits.60,61 There is also interest in evaluating the use of biological agents to increase permeability of the BBB. Experimental data have demonstrated the effectivness of vasoactive compounds, including histamine, leukotriene C4, β-interferon, tumor necrosis factor-α, and bradykinin. The bradykinin analog RMP-7 (Cereport) selectively increases delivery of radiolabeled carboplatin to brain tumor in animal xenograft models and improves survival. However, a recent randomized double-blind, placebo controlled phase II study showed that RMP-7 does not improve efficacy of carboplatin in patients with recurrent malignant glioma.62
High-Dose Chemotherapy
Considering that the BBB is a major factor in brain tumor resistance to chemotherapy and that diffusion across this barrier depends on the concentration-time profile of the free fraction of drug
(i.e., drug that is not bound to plasma proteins), the assumption has been that increasing the administered dose would drive more drug across the BBB.10 The rationale for HDCT was derived from the relatively linear in vitro dose-response curve exhibited by the classic alkylating agents and the assumption that intrinsic cellular resistance could be overcome by increasing the dose. In the context of treating brain tumors, HDCT could overcome the previously mentioned sink effect and provide higher drug concentrations in the tumor for sustained periods. A number of phase I and II studies have been undertaken to evaluate this approach.63 Despite the theoretical advantages, HDCT for recurrent malignant glioma has not made a significant impact on patient survival, although anecdotal cases of long-term survival have been observed. Among patients with newly diagnosed tumors, the median survival achieved using HDCT with bone marrow or stem cell rescue is comparable to that with conventional-dose chemotherapy (12 to 26 months versus 10 to 12 months, respectively). Treatment-related morbidity and mortality have been high, however, with a mortality rate as great as 27% in one early study. More recently, HDCT with autologous stem cell rescue was used with apparent success in treatment of medulloblastoma and malignant astrocytic tumors in children.64 Adult patients with recurrent medulloblastoma do not seem to benefit from HDCT.65 Safety and efficacy of HDCT were also addressed by investigators in the treatment of recurrent CNS germinomas and malignant astrocytomas in adults.66,67 HDCT in conjunction with stem cell rescue achieved prolonged tumor control in the treatment of newly diagnosed anaplastic oligodendroglioma.68 The strategy of HDCT followed by stem cell rescue may become an effective treatment strategy for potentially chemosensitive brain tumors such as anaplastic oligodendroglioma and primary CNS lymphoma (PCNSL). The best overall survival data achieved for patients with PCNSL have been reported after HDCT.68, 69, 70, 71, 72, 73
(i.e., drug that is not bound to plasma proteins), the assumption has been that increasing the administered dose would drive more drug across the BBB.10 The rationale for HDCT was derived from the relatively linear in vitro dose-response curve exhibited by the classic alkylating agents and the assumption that intrinsic cellular resistance could be overcome by increasing the dose. In the context of treating brain tumors, HDCT could overcome the previously mentioned sink effect and provide higher drug concentrations in the tumor for sustained periods. A number of phase I and II studies have been undertaken to evaluate this approach.63 Despite the theoretical advantages, HDCT for recurrent malignant glioma has not made a significant impact on patient survival, although anecdotal cases of long-term survival have been observed. Among patients with newly diagnosed tumors, the median survival achieved using HDCT with bone marrow or stem cell rescue is comparable to that with conventional-dose chemotherapy (12 to 26 months versus 10 to 12 months, respectively). Treatment-related morbidity and mortality have been high, however, with a mortality rate as great as 27% in one early study. More recently, HDCT with autologous stem cell rescue was used with apparent success in treatment of medulloblastoma and malignant astrocytic tumors in children.64 Adult patients with recurrent medulloblastoma do not seem to benefit from HDCT.65 Safety and efficacy of HDCT were also addressed by investigators in the treatment of recurrent CNS germinomas and malignant astrocytomas in adults.66,67 HDCT in conjunction with stem cell rescue achieved prolonged tumor control in the treatment of newly diagnosed anaplastic oligodendroglioma.68 The strategy of HDCT followed by stem cell rescue may become an effective treatment strategy for potentially chemosensitive brain tumors such as anaplastic oligodendroglioma and primary CNS lymphoma (PCNSL). The best overall survival data achieved for patients with PCNSL have been reported after HDCT.68, 69, 70, 71, 72, 73
Intrathecal Administration
IT chemotherapy involves the direct injection of drug into the CSF and is an obvious way to bypass the BBB. It is accomplished by injecting drug into the lumbar subarachnoid space, the cerebral ventricles, or the basal cisterns, with or without the use of catheters, pumps, or ventricular reservoirs.74 The rationale is that the cells lining the fluid spaces of the brain are permeable, which results in a free exchange of molecules from extracellular fluid (ECF) to CSF and vice versa. Relatively small doses of a drug given by IT injection can achieve high local concentrations, due to the low volume of CSF (˜150 mL), which thereby minimizes systemic toxicity. Furthermore, because of the intrinsically low levels of enzymes in the CSF, agents that are subject to rapid metabolism in blood, such as cytosine arabinoside (ara-C), remain in the active form in CNS for a longer periods of time. The three drugs administered most commonly in this manner are methotrexate sodium, ara-C, and thiotepa (thiotriethylene phosphoramide). These agents have been used mainly for the treatment of leptomeningeal metastases from systemic cancer.75 Other agents that have been tested in the clinical setting via IT route include topotecan,76,77 trastuzumab,78, 79, 80 and rituximab.81,82
IT drug administration has disadvantages, including the necessity to establish access to the CSF compartment. The drug can be administered via lumbar route by performing a satndard lumbar puncture or through a ventricular catheter (Fig. 5-5). A ventricular access device is usually implanted in the frontal horn of the lateral ventricle to facilitate the administration of drug and minimize patient discomfort. This entails a small surgical risk of hemorrhage and infection. Moreover, the catheter may malfunction over time and require replacement.83 IT drug administration also has numerous pharmacokinetic limitations. Among these, the drug must overcome bulk flow of CSF to penetrate the cisterns and ventricles (Fig. 5-6). In addition, the flow of interstitial fluid produced by brain cells and microvessels from ECF to CSF counteracts the diffusion of drug from CSF to ECF. Estimates are that CSF is completely renewed every 6 to 8 hours; thus, the concentration of a drug injected into the CSF is diluted continuously as a consequence of this process. This dilution can only be overcome by a continuous infusion or sustained-release system to maintain a clinically relevant concentration. Development of a liposomal form of ara-C allows sustained release of this drug into CSF and increases the effective half-life of the agent in the CSF by almost 50-fold.84
![]() FIGURE 5-5 Different approaches to IT delivery of drugs. (From National Cancer Institute and Universtiatsklinikum Bonn; www.meb.uni-bonn.de, with permission.) (Please see Color Insert.) |
Another pharmacologic disadvantage of the IT route is the fact that production of CSF by the choroid plexus and its elimination into the venous circulation may be altered by the tumor itself, which disturbs bulk flow and modifies drug distribution and diffusion. For example, the clearance of methotrexate from CSF is decreased in the presence of leukemic meningitis.85,86 Moreover, diffusion in the ventricular space is heterogeneous87 and may result in uncertain and potentially toxic local concentrations in CSF, even if continuous-infusion devices are used.74 Finally, and most important,
brain tumors are often in locations not adjacent to the ventricular system and may require diffusion of drug from CSF to tumor over a distance of several centimeters, which impairs the ability to achieve cytotoxic concentrations of the drug at the site of the tumor. In the case of methotrexate, the concentration of drug has been calculated to be no more than 0.1% of the CSF concentration 1 cm from the ependymal edge 48 hours after IT administration.88 However, the relationship is quite complex. Whereas compounds with greater lipophilicity will access the ECF more effectively, they will also be subject to a higher rate of removal by the vascular and cellular compartments, thereby limiting the extent of drug penetration into brain tissue.89 Therefore, at this point in time, IT chemotherapy is not feasible for brain tumors and is restricted to the treatment and prevention of leptomeningeal metastases.90
brain tumors are often in locations not adjacent to the ventricular system and may require diffusion of drug from CSF to tumor over a distance of several centimeters, which impairs the ability to achieve cytotoxic concentrations of the drug at the site of the tumor. In the case of methotrexate, the concentration of drug has been calculated to be no more than 0.1% of the CSF concentration 1 cm from the ependymal edge 48 hours after IT administration.88 However, the relationship is quite complex. Whereas compounds with greater lipophilicity will access the ECF more effectively, they will also be subject to a higher rate of removal by the vascular and cellular compartments, thereby limiting the extent of drug penetration into brain tissue.89 Therefore, at this point in time, IT chemotherapy is not feasible for brain tumors and is restricted to the treatment and prevention of leptomeningeal metastases.90
Intratumoral Administration
The simplest and most direct way to guarantee that a cytotoxic drug reaches its target is to deliver it directly into the tumor or into the cavity left after tumor resection. As with IT injection, this bypasses most of the previously mentioned obstacles pertaining to systemic drug administration for treating brain tumors. Systemic toxicity may also be reduced because substantially lower doses of drug may be given, and only a relatively small amount of drug distributes from the CNS to the bloodstream. A particularly attractive advantage of this strategy is that anticancer drugs (hydrophilic, high molecular weight) that are normally impeded by the BBB may be used. Conceptually, the low permeability of the BBB to such compounds should promote their retention within the CNS by inhibiting distribution into the bloodstream. Due to the high local drug concentrations that can be achieved, better distribution of drug may be provided within the tumor by diffusion and convection driven by the hydrostatic pressure of the tumor. The two techniques that have been most commonly used for directly introducing chemotherapeutic agents into brain tumors are parenteral delivery as either a bolus injection or continuous infusion through a cannula and implantation of drug embedded in a slow-release carrier system.
The feasibility of intratumoral infusion has been demonstrated in a number of clinical trials involving approximately ten different anticancer agents.91 These studies, however, have not shown a clear survival advantage or direct evidence of increased drug delivery within the tumor. Furthermore, toxicity has been observed with this technique, including nervous system injury and infection.91
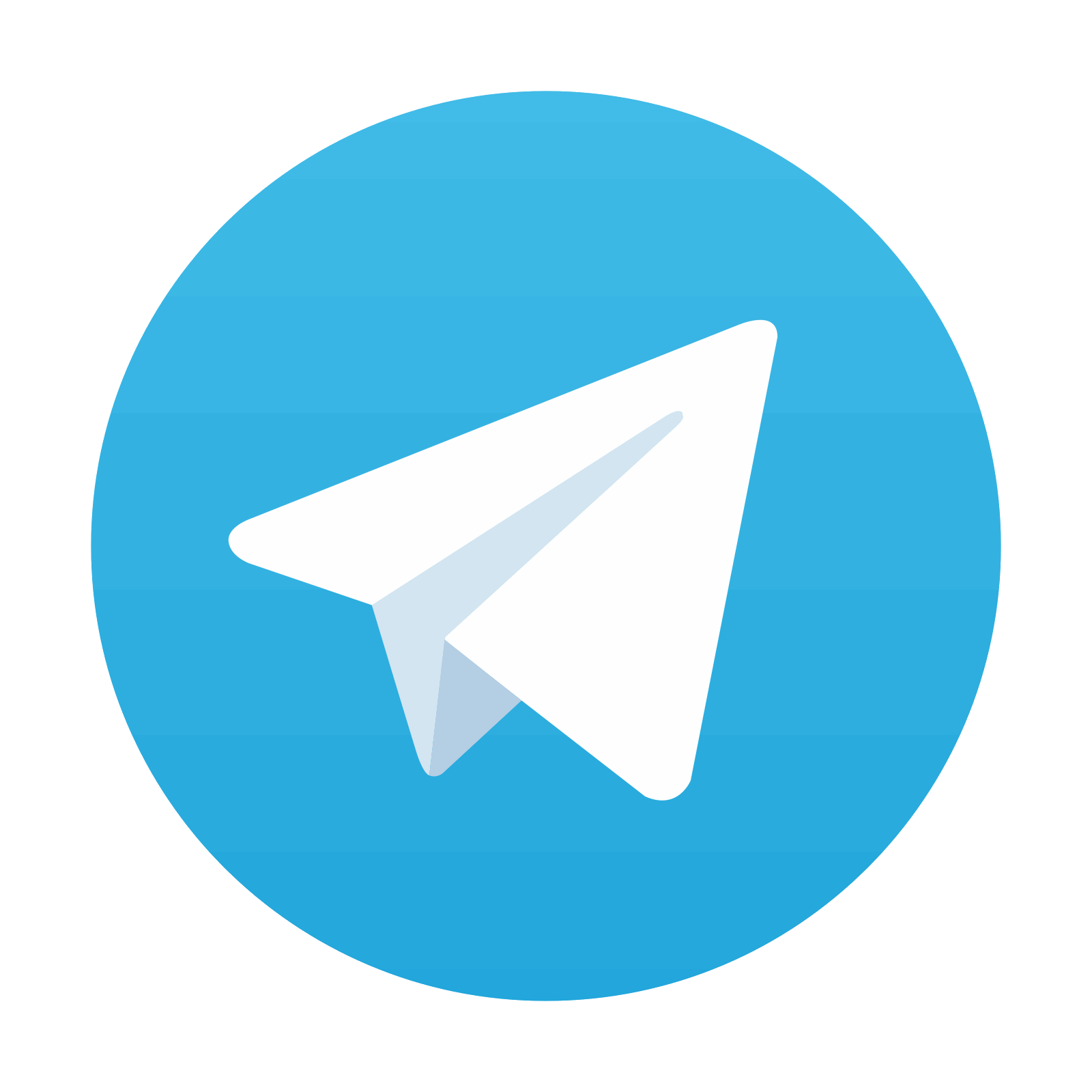
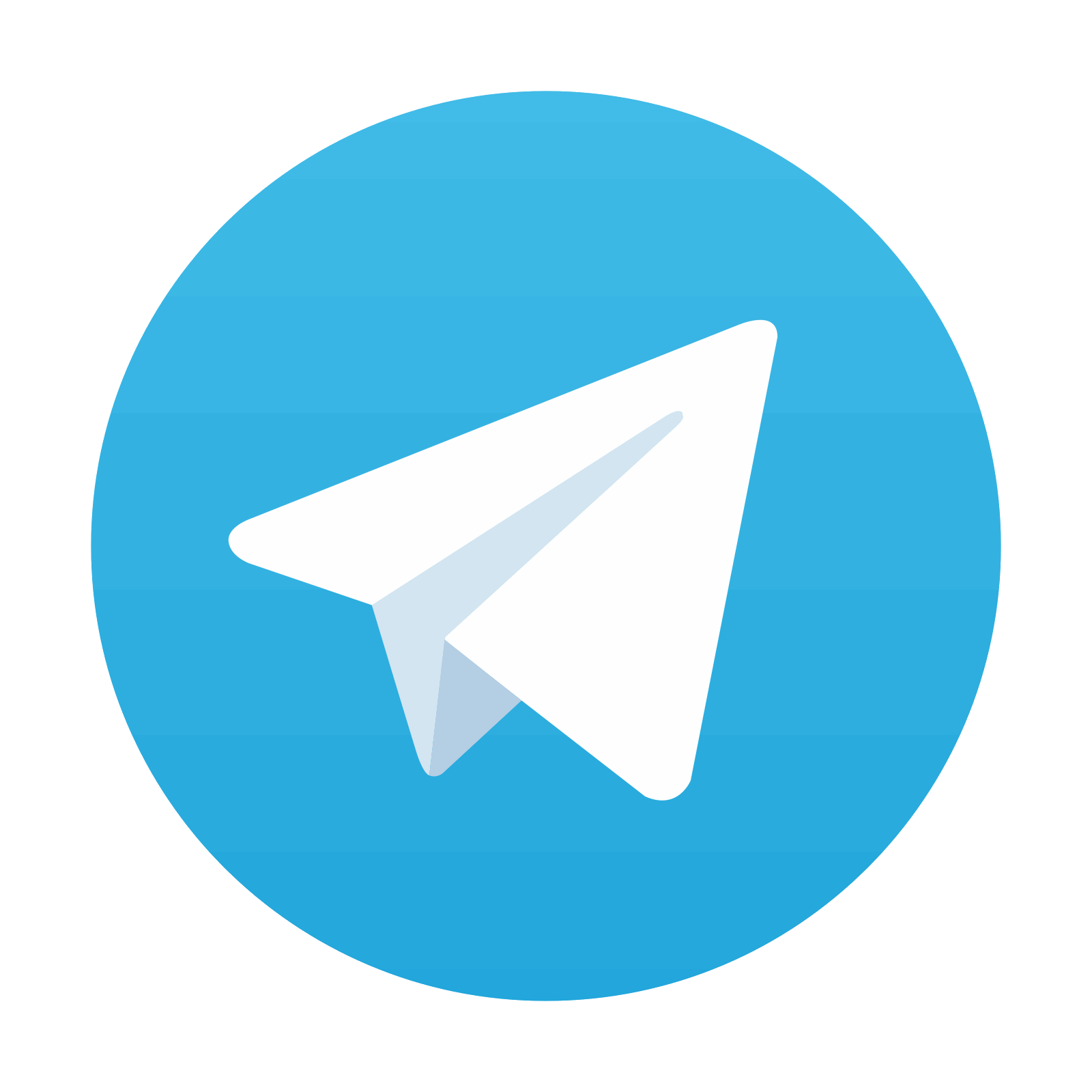
Stay updated, free articles. Join our Telegram channel

Full access? Get Clinical Tree
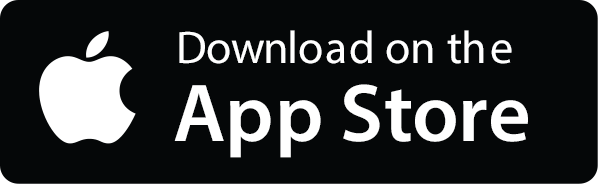
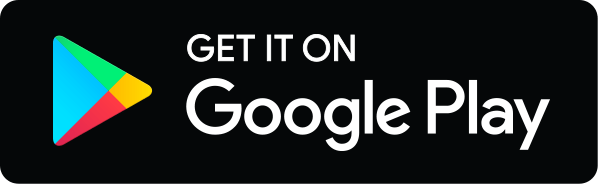
