Cytokines, Growth Factors, and Immune-Based Interventions
Cytokines are soluble proteins or glycoproteins that exert trophic effects on a variety of targets based on the expression of particular ligand-specific receptors on the target. All of the cytokines have not yet been identified; but at this time, more than 80 different molecules have been defined. The same cytokine can exert different effects on different cells and tissues. However, the biochemical consequences within the cell of ligand binding to its cellular receptor are similar among all the targets. A number of cytokines have been evaluated for their antitumor effects including the interferons, interleukin-1 (IL1), tumor necrosis factor, IL4, IL12, and others. The rationale for testing these agents as antitumor agents is twofold. First, many of these agents stimulate cells of the immune system, an effect that could promote the immunological killing of the tumor cells. Second, many neoplastic cells retain the cytokine receptors of their normal counterparts; thus, direct biological and potentially antitumor effects are theoretically possible.
Currently, only interferon-α (Chapter 13) and IL2 are approved for use as anticancer agents. Most other tested cytokines have either had little or no antitumor effect or were too toxic when administered systemically as a pharmacologic agent. In general, cytokines work physiologically as paracrine signals coordinating cellular responses in a localized area of release. It has been estimated that in the course of trying to develop IL2 as a therapeutic agent, we administered more of the agent to a few hundred patients than had been produced physiologically in the courses of their entire lives by every man and woman who ever lived.
INTERLEUKIN-2
Interleukin-2 (IL2) is a glycoprotein composed of 133 amino acids and has a molecular weight of 15 kD. It is structurally related to IL4, IL15, and granulocyte-macrophage colony-stimulating factor (GM-CSF). It is normally produced by stimulated T cells and NK cells and acts to promote the proliferation of activated T cells. Resting T cells do not express IL2 receptors and do not respond to the cytokine.
The IL2 receptor has three components: an α-chain, a 55-kD component, also known as CD25, that has only 13 amino acids located intracellularly and functions mainly in binding to IL2; a β-chain, a 75 kD component with a large intracellular component involved in signaling; and the common γ-chain, a 64-kD component called “common” because it is also a shared signaling component of receptors for IL4, IL7, IL9, IL15, and IL21. IL2 binds to the three-component high-affinity receptor with a Kd of 10 pmol/l; in the absence of the α-chain, IL2 binding is termed intermediate and is about 100-fold reduced. High-affinity receptors are mainly expressed on activated T cells; intermediate affinity receptors are expressed on monocytes and NK cells.
Biologic activity. IL2 stimulates the proliferation of activated T cells and promotes the secretion of cytokines from monocytes and NK cells. The main biologic consequence of IL2 stimulation is an increase in cytotoxicity in both T cells and NK cells. IL2 also has a negative regulatory effect on T cells to prevent them from overexpanding or attacking self as IL2 knockout mice have lymphadenopathy and autoimmunity.
Pharmacology. The serum half-life of IL2 after intravenous administration has an α-phase of about 13 min and a more prolonged β-phase of about 90 min. Peak serum levels vary with the dose; 6 × 106 IU/m2 by IV bolus produces serum levels near 2000 IU/ml. IL2 has been conjugated to polyethylene glycol to prolong its half-life (α 3 h; β 12½ h), but this form is not FDA approved. It is mainly excreted as an inactive metabolite in the urine. When 6 × 106 IU/m2 IL2 is administered by continuous infusion, it reaches steady-state levels within 2 h at 123 IU/ml and levels fall rapidly after the infusion is stopped. When 6 × 106 IU/m2 IL2 is administered subcutaneously, peak serum levels of 32–42 IU/ml are reached within 2–6 h.
Method of administration. Chiron IL2 (aldesleukin) is the only form of IL2 currently FDA approved. It is administered in one of three ways. High-dose IL2 is 600,000 or 720,000 IU/kg administered by IV bolus every 8 h until dose-limiting toxicity is reached or a maximum of 15 doses. Low-dose IL2 is 60,000 or 72,000 IU/kg administered by IV bolus every 8 h for 15 doses. A third regimen is for more chronic administration: 250,000 IU/kg subcutaneously daily for 5 days, then 125,000 IU/kg daily for 6 weeks. Considerable data exist on high-dose and low-dose schedules. Much less information is available on the activity of the subcutaneous regimen. Treatment is generally repeated at least once in responding patients.
Because of its life-threatening toxicities (see below), patients must be carefully screened before embarking on a course of IL2 treatment. Patients should undergo cardiac stress testing, pulmonary function tests, brain MRI, and a thorough physical examination and laboratory testing before treatment. They should have a good performance status (0.1 on ECOG scale), no active infections, and normal renal, hepatic, and thyroid function.
Clinical effects. IL2 was approved for use in metastatic renal cell cancer in 1992 and in metastatic melanoma in 1998 (1, 2). High-dose IL2 produces an overall response rate of about 19% in patients with renal cell cancer; however, 8% of patients get complete responses. Both complete and partial responses appear to be quite durable with median response durations of 8–9 years. Thus, median survival is not affected appreciably, but a subset of patients receives substantial benefit from the therapy. Unfortunately, it is not possible to distinguish in advance patients more likely to respond.
High-dose IL2 produces an overall response rate of 16% in metastatic melanoma and 6% of patients achieve complete responses, many of which are long lasting. Median response duration is about 5 years.
The role of high-dose therapy versus low-dose therapy is controversial. Many argue that response rates are the same with the two regimens. However, response durations do not seem to be as durable when low-dose IL2 is used, at least in some studies. Other groups have not seen dramatic differences in efficacy between high- and low-dose regimens, but all groups have noted dramatic differences in toxicities. The mechanism of action of IL2 against these cancers is undefined.
A novel use for IL2 has been developed as more information has emerged about T-cell subsets and their function. A subset of CD4+ T cells known as regulatory T cells (Tregs) are CD25+ and express the FoxoP3 transcription factor. These cells function to suppress T-cell mediated immune responses. Daily administration of IL2 at a dose of 106 IU/m2 is effective in some autoimmune diseases such as chronic graft-vs-host disease and hepatitis C-induced vasculitis (3).
Toxicities. The toxicities from IL2 are life-threatening and are dominated by the capillary leak syndrome (4). Intravascular fluid leaks into the extravascular space, tissues, and alveoli of the lungs. As a consequence, patients develop hypotension, edema, respiratory difficulties, confusion, tachycardia, oliguric renal failure, and electrolyte abnormalities including hypokalemia, hypomagnesemia, hypocalcemia, and hypophosphatemia. Patients may also experience nausea and vomiting, fever, chills, malaise, and thrombocytopenia. Diarrhea, abnormal liver functions, and neutropenia may occur. Patients often develop a pruritic skin rash over most of the body. Hypothyroidism may also occur. Arrhythmias are a rare complication.
Despite the severity and widespread distribution of the toxic effects of IL2, nearly all the toxicities are reversible within 24–48 h of stopping the drug.
DENILEUKIN DIFTITOX (IL2-DIPHTHERIA HYBRID TOXIN)
Mechanism of action. The fusion protein delivers a potent cellular toxin (diphtheria) to CD25-expressing malignant cells inhibiting cellular protein synthesis and leading to cell death.
Pharmacology. Following the first dose, the agent has a distribution phase half-life of 2–5 minutes and a terminal phase half-life of 70–80 minutes. The development of neutralizing antibodies enhances clearance with subsequent courses.
Administration. Because of infusion reactions, patients are usually premedicated with an antihistamine and acetaminophen before infusion. The drug is given at a dose of 9 or 18 μg/kg/day by IV infusion over 30–60 minutes on 5 consecutive days every 21 days for a total of 8 cycles. The drug is not given if serum albumin levels are less than 3 g/dl.
Toxicity. Hypersensitivity reactions (although most are controllable/preventable by slowing the rate or temporarily interrupting the infusion and treating with antihistamines, acetaminophen, and possibly glucocorticoids, they can be severe or life-threatening); respiratory (dyspnea); gastrointestinal; constitutional (flulike); vascular leak syndrome; rash; elevations of hepatic enzymes (not usually accompanied by other liver abnormalities); renal insufficiency; anemia; thrombocytopenia; hemolysis; proteinuria; and increased risk of infections. Most patients develop antibodies against the toxin/IL2, and these may impact on clearance rates that tend to be two to three times more rapid by the third course. Patients may also lose visual acuity and color vision; thus, these should be monitored during treatment.
Clinical effectiveness. Approved for treatment of persistent or recurrent cutaneous T-cell lymphomas (CTCL) expressing the CD25 antigen (5).
COLONY-STIMULATING FACTORS
The relatively disappointing antitumor efficacy of cytokines has been counterbalanced by the more effective use of a group of cytokines in supportive care of the cancer patient. The lesson learned from these development efforts is that cytokines are more effectively applied to people when they are used to influence their known physiologic targets. Thus, colony-stimulating factors are capable of increasing the production of the cells they normally regulate. However, here, too, we have learned the physiologic limitations of the hematopoietic system. Generally, when we make a patient anemic or granulocytopenic or thrombocytopenic with chemotherapy or radiation therapy, the problem is not that the physiologic response to the cytopenia is limited by poor production of the relevant colony-stimulating factor. Instead the limitation is the number of surviving marrow precursors and the obligate time period for their differentiation into end-stage cells. Thus, even when a cytokine is used to perform its physiologically relevant task, it does not act as a cure-all that erases the prior damage of disease and therapy. Nevertheless, colony-stimulating factors have made a modest contribution to more rapid recovery of blood counts after treatment.
Unfortunately, the magnitude of the effect of colony-stimulating factors has not been sufficient to influence the maximally tolerated doses of myelotoxic agents, a result that was hoped for when these agents were first introduced. However, clinical experience has defined settings in which their use can be beneficial, and guidelines for clinical use have been developed.
GRANULOCYTE-COLONY-STIMULATING FACTOR
Granulocyte-colony-stimulating factor (G-CSF) is a 174-amino acid glycoprotein (MW 19,600) encoded by a gene on chromosome 17q11–12 that acts late in myeloid cell differentiation to promote the development of granulocytes. Not only is granulocyte production increased by G-CSF, but the generation of reactive oxygen species by granulocytes is also augmented. Over time additional functions have been uncovered, and its use is now being evaluated in cardiac disease and stroke. It may have a role in suppressing immune reactions.
G-CSF production is usually induced by inflammatory cytokines, and it is produced by fibroblasts, macrophages, and endothelial cells. The receptor for G-CSF is in the cytokine type I receptor family and signals through Janus-like kinase (JAK)/signal transducer and activator of transcription (STAT) pathways.
Biologic activity. When added to bone marrow cell cultures, G-CSF mainly stimulates the development of neutrophils, in contrast to GM-CSF, which induces neutrophil, eosinophil, basophil, monocyte, and dendritic cell development. In addition to increasing neutrophils in the marrow, G-CSF promotes the early release of these cells into the peripheral blood and promotes their ability to phagocytose and kill bacteria. Through the release of metalloproteinases, they also promote the mobilization of hematopoietic stem cells into the peripheral blood.
Pharmacology. Intravenous administration of G-CSF (filgrastim) shows an α-phase half-life of about 8 min and a β-phase half-life of about 2 h. When given subcutaneously, the half-life is 2.5–5.8 h. To prolong the half-life, a 20-kD polyethylene glycol molecule was covalently attached to the N-terminal methionine of filgrastim to produce pegfilgrastim. The half-life of subcutaneously administered pegfilgrastim is 27–47 h.
Method of administration. Filgrastim is generally administered at a dose of 5 μg/kg subcutaneously daily. When given to promote granulocyte recovery, the daily dose is continued until the neutrophil count has increased above 10,000/μl. Pegfilgrastim is usually administered only once at a dose of 100 μg/kg or a total dose of 6 mg subcutaneously. A single dose of pegfilgrastim appears comparable in efficacy to a 10–14 day course of filgrastim. For mobilization of stem cells, the usual dose of filgrastim is 10 μg/kg/day or 5–8 μg/kg twice daily.
Clinical effect. Based on expert opinion and analysis of the world’s literature on G-CSF use (6, 7), guidelines have been developed to aid in decision making on who should and who should not receive G-CSF during chemotherapy (Table 14-1). In general, G-CSF is overused in clinical practice. The guidelines suggest that it be used with regimens that have a greater than 20% likelihood of inducing febrile neutropenia. Only a small fraction of frequently used regimens are in this category. Risk of developing febrile neutropenia is reduced by about 50%. In the setting of febrile neutropenia, G-CSF may speed neutrophil recovery by 2 or 3 days. However, its use has not permitted dose escalation of hemotherapy. G-CSF is extremely effective in mobilizing hematopoietic stem cells into the peripheral blood. It is so effective that bone marrow harvest has become unnecessary in the vast majority of stem cell donors. Not only are peripheral blood stem cells easier to collect from the donor, but G-CSF-mobilized cells are also more efficient at reestablishing normal hematopoiesis than bone marrow-derived cells and are associated with shorter periods of neutropenia and thrombocytopenia.
Toxicities. The acute toxicity associated with G-CSF use is minor. A few patients may experience bone pain. In normal individuals receiving G-CSF to mobilize hematopoietic stem cells, rapid splenic enlargement is possible and rare splenic rupture has occurred. Thus, these patients need to be monitored for abdominal or shoulder pain.
More serious concerns are emerging about long-term effects. First, animal studies have shown that the amount of damage to hematopoietic stem cells by cyclic chemotherapy is increased with the use of colony-stimulating factor support to hasten recovery (8). In addition, at least three studies have reported an increase in the incidence of acute leukemia and myelodysplasia when cancer therapy was supported with G-CSF use compared to the incidence with chemotherapy alone (9–11). The precise mechanism of the G-CSF effect is unclear. Possibly, through its antiapoptotic effects, it keeps damaged cells alive that would normally die. Regardless of mechanism, the twofold increased leukemia/myelodysplasia risk is sufficient to motivate clinicians to use the agent more sparingly and only when indicated, especially when cure is the goal.
GRANULOCYTE-MACROPHAGE COLONY-STIMULATING FACTOR
Granulocyte-macrophage colony-stimulating factor (GM-CSF) is a 127-amino acid glycoprotein (MW 22 kD) encoded by a gene on chromosome 5q31 that acts early and late in myeloid cell development. The GM-CSF receptor has a unique α-chain called CSF2R and shares a β-chain with the IL3 and IL5 receptors. It stimulates the common myeloid progenitor to differentiate toward the granulocyte/monocyte progenitor rather than the erthroid/megakaryocyte progenitor, and it stimulates an increase in all the progeny of the granulocte/monocyte progenitor. It also activates granulocytes, monocytes, and macrophages and promotes the antigen-presenting function of dendritic cells. Like G-CSF, it is produced by macrophages, fibroblasts, and endothelial cells, but unlike G-CSF, GM-CSF is also produced by T cells.
Biologic activity
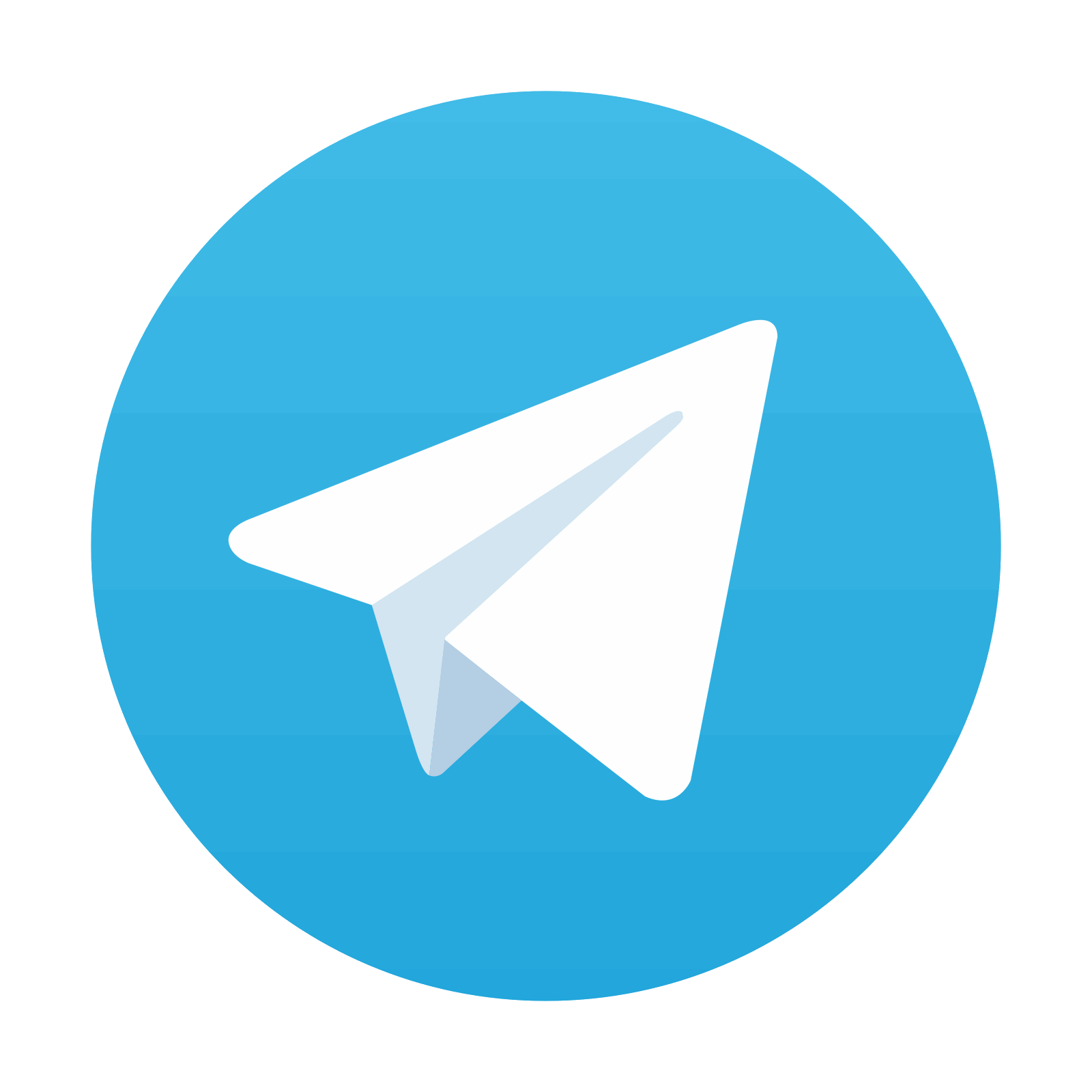
Stay updated, free articles. Join our Telegram channel

Full access? Get Clinical Tree
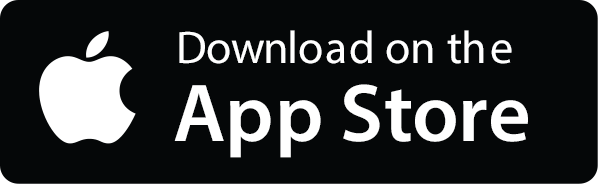
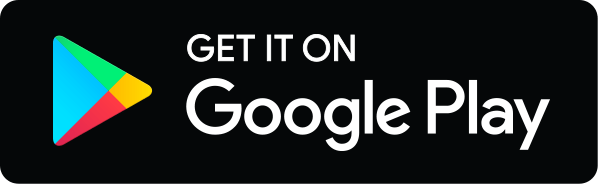