(1)
Mayo Clinic, Jacksonville, Florida, USA
5.1 Introduction
Drugs that inhibit vascular endothelial growth factor (VEGF) are effective for center-involving diabetic macular edema (DME) since most eyes respond well to regimens that include repeated intravitreal injections. A significant minority of patients (approximately 40%), however, exhibit an inadequate response and require additional or alternate therapy. In the phase III anti-VEGF registration trials, laser photocoagulation served as secondary therapy for patients with inadequate responses after 3–6 months of monthly anti-VEGF injections [52, 66]. Supplemental laser usually resolves persistent edema slowly, but it has not been shown to incrementally improve VA in most cases [26, 62].
Glucocorticoids were the first drug class shown to improve DME in randomized clinical trials [34–36]. Triamcinolone acetonide, the first drug to be tested (Kenalog®; Bristol-Myers Squibb, New York, NY), was not originally formulated for intraocular use, and it was associated with a high risk of cataract and elevated intraocular pressure. Topical and periocular corticosteroids [24] do not significantly improve DME because they do not penetrate the sclera sufficiently to produce therapeutic concentrations within the retina. Intravitreally injected corticosteroids resolve macular edema and improve visual acuity in both treatment-naïve eyes and those previously treated with laser and/or anti-VEGF therapy. As a result, significant interest in treating DME with intravitreal corticosteroids has developed, and this chapter reviews the current state-of-the art corticosteroid use in the management of patients with DME.
5.2 Characteristics of Corticosteroids
The primary structure of VEGF – a long amino acid sequence – prevents it from crossing the cell membrane’s lipid bilayer, whereas the lipophilic ring structures of corticosteroids enable them to pass easily. Corticosteroids bind to heat shock proteins and several soluble steroid receptors within the cytoplasm [20] and the resultant complex migrates to the cell nucleus (Fig. 5.1). The heat shock protein dissociates, leaving the corticosteroid-receptor complex to bind to the promoter or repressor regions of several genes [11, 29]. The corticosteroid-receptor complex can also produce signal transduction within the cytoplasm, stimulating the release of molecules such as annexin-1 [77], a modulator of leukocyte migration [23].


Fig. 5.1
This drawing shows the mechanisms through which corticosteroids exert their effects. Corticosteroids dissociate from serum transport proteins, enter target cells by crossing cell membranes, and bind to cytoplasmic receptors that include heat shock proteins (hsp90). The corticosteroid-receptor complexes enter cell nuclei, interact with DNA receptor sites, and suppress nuclear factor (NF)-κB. This increases the expression of many species of mRNA and suppresses others; both mechanisms affect the synthesis of thousands of proteins. Corticosteroids also act as survival factors for neuroglia and directly promote vasoconstriction
Corticosteroids improve macular edema via several mechanisms. They bind to the promoter region of the VEGF gene and downregulate VEGF synthesis [33, 74]. The resultant free-VEGF levels drop significantly, though they remain 100-fold higher than after the intravitreal injection of anti-VEGF drugs. Steroids decrease exudation by favorably altering the Starling’s law equilibrium through vasoconstriction. Steroids decrease average capillary lumen pressure, decrease the mean transcapillary exudative pressure, and reduce the hydrostatic pressure gradient.
Inflammation contributes significantly to the pathogenesis of diabetic retinopathy, and corticosteroids are potent anti-inflammatory molecules. Corticosteroids repress several key pro-inflammatory transcription markers such as nuclear factor-kappa B (NFĸB) and activator protein 1, thereby disrupting the pro-inflammatory feedback loop that is critical for the development of DME [80, 89]. Corticosteroids inhibit phospholipase A2 [79], which is upregulated in the retinas of streptozotocin-induced diabetic rates [40, 57] and has been associated with increased ICAM-1, VEGF, and TNF-α expression, and the formation of DME [57, 67]. Corticosteroids are known to reduce tissue edema and downregulate the release of prostaglandins and histamines [87]. Corticosteroids inhibit the synthesis of endothelial nitric oxide synthase (eNOS), a potent vasodilator [56]. Each of these factors causes vasodilation and/or edema and leads to choroidal thickening. Corticosteroids decrease the synthesis of several chemokines, intercellular adhesion molecules, and growth factors that inhibit the migration and margination of polymorphonuclear leukocytes [55]. These are capable of disrupting the VEGF feedback loop and downregulating several VEGF-mediated processes. Corticosteroids also upregulate the expression of anti-inflammatory proteins that downregulate the inflammatory response: interleukin (IL)-10, adenosine, and IĸBα (the natural inhibitor of NFĸB).
Steroids maintain and restore the blood-retinal barrier by preventing phosphorylation of tight junction proteins [39, 86]. Corticosteroids improve tight junction integrity by upregulating junctional protein expression and facilitating their translocation to the cellular borders [3] and by protecting against oxidative stress-induced phosphorylation of tight junction proteins in RPE cells [63]. Corticosteroids increase fluid movement through the retina by stabilizing Müller cells and improving aquaporin-4 (AQP-4) and potassium channels [70, 90].
The neuroprotective capabilities of a constant infusion of low-dose fluocinolone were demonstrated in transgenic S334ter-4 and Royal College of Surgeons rats. Fluocinolone preserves outer nuclear layer cell morphology, stabilizes a- and b-wave electroretinography amplitudes, and reduces neuroinflammation [37, 38].
Triamcinolone, dexamethasone, and fluocinolone are all fluorinated glucocorticoids that lack mineralocorticoid activity. They differ according to their glucocorticoid-receptor binding affinities (dexamethasone > triamcinolone > fluocinolone) and their lipophilicity (triamcinolone > fluocinolone > dexamethasone). These characteristics may be partially responsible for their relative potencies (triamcinolone = 5, dexamethasone = 25, fluocinolone = 25, compared to cortisol = 1) (Table 5.1).
Table 5.1
This table lists the important characteristics of the corticosteroids used for the treatment of diabetic macular edema
Characteristics of corticosteroids that are used to treat diabetic retinopathy | |||
---|---|---|---|
Characteristic | Dexamethasone | Fluocinolone | Triamcinolone |
Molecular weight (kDa) | 0.392 | 0.452 | 0.394 |
Binding affinity (nmol) | 5.4 | 2.0 | 1.5 |
Intravitreal half-life | 5.5 h | Unknown | 18 days (crystalline) |
Relative potencies(cortisol = 1) | 25 | 25 | 5 |
Corticosteroids differ according to the proteins that they regulate. Triamcinolone, dexamethasone, and fluocinolone each upregulate over 6000 proteins in each of two types of retinal pigment epithelial cells, but only 15–25% of these proteins are upregulated by all three corticosteroids. Within trabecular meshwork (TM 86) cells, dexamethasone upregulates transcripts associated with RNA posttranscriptional modifications, fluocinolone affects lipid metabolism, and triamcinolone affects cell morphology; within TM 93 cells dexamethasone affects histone methylation, fluocinolone affects the cell cycle, and triamcinolone affects cell adhesion [65].
Solubilized fractions of the 3 corticosteroids have brief intravitreal half-lives of only 2–5 h [29]. Crystalline deposits of triamcinolone acetonide are minimally soluble in aqueous, and they slowly release steroid with a half-life of approximately 18 days [75]. Since triamcinolone and dexamethasone are less lipophilic than fluocinolone, some authors speculate that fluocinolone may accumulate less in the anterior chamber and cause fewer steroid-related intraocular pressure rises [69].
Corticosteroid administration by several routes (topical, periocular, intraocular, systemic) frequently increases intraocular pressure and causes posterior subcapsular cataracts [5, 6, 43]. Cataract formation and IOP elevation may be due to activation of a corticosteroid receptor found in both the lens and trabecular meshwork [34].
5.3 Corticosteroid Delivery to the Eye
The timeline in Fig. 5.2 shows important milestones in the use of intraocular corticosteroids for the treatment of DME.


Fig. 5.2
This figure details the timeline for the intraocular use of corticosteroids. Many of the most important milestones in the history of corticosteroid use and delivery system creation are listed
Since corticosteroids are small and lipophilic, they easily cross cell membranes and the blood-retinal barrier to reach glucocorticoid receptors in target tissues. Parenterally administered corticosteroids have large volumes of distribution, so high doses would need to be given to improve retinal vascular conditions and significant side effects would be frequent. Corticosteroid drops and periocular depot injections would be unlikely to cause systemic adverse events, but they must penetrate several tissue layers to reach the retina in therapeutic concentrations [44].
The composition of tears together with their rapid elimination through the lacrimal drainage system via the blink mechanism constitutes the major barriers to topically administered drug penetration into the eye. Drug that manages to penetrate the corneal and conjunctival epithelium may be rapidly eliminated by conjunctival lymphatics and uveal blood flow. Unfortunately, topically administered drugs generally have intravitreal bioavailability of less than 0.001% [60].
Topical corticosteroids are effective against several chorioretinal vascular conditions in rats because the diffusion distance from the cornea to the retina is very short. The larger anteroposterior diameter of the human eye (approximately 24 mm), however, makes it nearly impossible to achieve clinically active concentrations within the retina. Corticosteroid formulations that may enhance ocular penetration and facilitate diffusion to the macula will be discussed in Chap. 8.
Sub-Tenon’s injections of triamcinolone acetonide treat uveitis and postoperative cystoid macular edema but are ineffective against DME [24]. Drug must penetrate several anatomic barriers (episclera, sclera, choroid, and retinal pigment epithelium) to reach the retinal vasculature. Furthermore, flow within the conjunctival lymphatics and vitreous moves drug away from the retina [50]. Together, these diffusion barriers decrease intravitreal bioavailability after a sub-Tenon’s injection to 0.01–0.1% [30]. Drug injections directly into the vitreous bypass the outer blood-retinal barrier and produce maximum bioavailability [30].
Several sustained-release reservoirs and extended duration strategies have been developed to prolong the retention of steroids within the vitreous. Triamcinolone sustained-release systems (Kenalog®; Trivaris®, Allergan, Irvine, CA, USA; Triesence®, Alcon, Fort Worth, TX, USA) are currently available for periocular and intraocular use (Fig. 5.3). Attempts to create sustained-release triamcinolone devices have thus far been unsuccessful, and further development has been halted. Biodegradable nanocarrier systems (liposomes, nanoparticles, nanocrystals, and nanosystems) are in various stages of development and may ultimately be used to treat posterior-segment disease [48]. Three sustained-release corticosteroid devices have been successfully developed and approved by the US Food and Drug Administration (US FDA) – the dexamethasone phosphate insert (DEX, Ozurdex®, Allergan, Irvine, CA, USA) (Fig. 5.4), the fluocinolone acetonide insert (Iluvien®, Alimera Sciences, Alpharetta, GA, USA), and the fluocinolone acetonide implant (Retisert®, Bausch & Lomb, Rochester, NY, USA). These will be discussed later in this chapter.



Fig. 5.3
This fundus photograph was taken 1 day after intravitreal injection of triamcinolone acetonide. Drug suspended in the posterior vitreous can be seen over the macula

Fig. 5.4
This ultra-widefield fundus photograph shows a dexamethasone insert in the inferior vitreous (black arrow)
5.4 Triamcinolone
Elevated aqueous levels of interleukin (IL)-8, interferon-induced protein-10 (IP-10), monocyte chemoattractant protein-1 (MCP-1), and VEGF were found in 22 eyes of 11 patients with DME compared to 6 control eyes [76]. In the 11 patients with DME, one eye was injected with 4 mg of triamcinolone acetonide (TA), and the other was injected with 1.25 mg of bevacizumab. Four weeks later, steroid-injected eyes showed a reduction in IP-10, MCP-1, VEGF, IL-6, and platelet-derived growth factor-AA (PDGF-AA), whereas only VEGF was reduced in bevacizumab-injected eyes. Significant clinical trials with intravitreal triamcinolone are detailed in Table 5.2.
Table 5.2
This table lists the important diabetic macular edema trials with triamcinolone acetonide and details their key findings
Important diabetic macular edema trials with triamcinolone | ||
---|---|---|
Trial and phase | Cohorts | Key findings |
DRCR.net Protocol B | Treatment arms IVT 1 mg IVT 4 mg Laser | At 2 years 1. Improvements in mean BCVA were −3, −2, and +1 letters 2. Elevated intraocular pressures seen in 16%, 33%, and 13% 3. Cataracts developed in 23%, 61%, and 13% |
Gillies et al. (2006) | Treatment arms IVT Laser | At 2 years 1. Eyes treated with IVTA were twice as likely to achieve 10 letter improvement in BCVA 2. 44% of IVT eyes required intraocular pressure lowering medications |
Kriechbaum et al. (2014) | Treatment arms IVT (8 mg) Bevacizumab (2.5 mg) | Comparable improvements in BCVA and macular thickness at 3 months At 12 months, VA stable in bevacizumab arm but decreased in IVT arm due to cataracts |
DRCR.net Protocol I 854 patients | Treatment arms RAN + laser RAN + def laser IVT + laser Laser | At 12 months 1. Eyes receiving IVT + laser achieved 5 fewer letters of visual improvement compared to RAN groups 2. Pseudophakic eyes receiving IVT + laser had comparable BCVA improvements as RAN groups |
Pilot studies showed that intravitreal injections of triamcinolone acetonide (IVTA) effectively reduce DME [4, 59]. In the prospective, randomized DRCR.net Protocol B trial, 1 and 4 mg intravitreal TA every 4 months were compared to laser photocoagulation. At 4 months, patients receiving 4 mg of TA were more likely to achieve +10-letter improvements in BCVA compared to laser (27% vs. 17%) and had greater mean decreases in central retinal thickness (CRT) (−98 μm vs. −39 μm). At the 2-year primary endpoint, however, the mean BCVA improvement in the laser group was +1 ± 17 letters compared to −3 ± 22 letters and −2 ± 18 letters in the 1 and 4 mg TA groups, respectively. Elevated IOP was seen in 33% of eyes receiving 4 mg triamcinolone, 16% of those receiving 1% triamcinolone, and 13% of those treated with laser. At 2 years, more patients receiving triamcinolone required glaucoma medications (4 mg, 13%; 1 mg, 6%; laser, 3%) and more developed cataracts (4 mg, 61%; 1 mg, 23%, laser, 13%) [25].
In a 2-year randomized clinical trial, IVTA was administered as often as every 6 months [36]. Eyes receiving IVTA were twice as likely as those treated with laser monotherapy to achieve +10-letter improvements in BCVA. The majority of eyes developed IOP problems: >5 mmHg rise in IOP (68%), need for glaucoma medication (44%), and need for cataract surgery (54%).
The effect of triamcinolone and bevacizumab on subfoveal choroidal thickness (SFCT) was investigated in a prospective, randomized study of 51 eyes with DME [78]. Compared to baseline, the mean SFCT in eyes receiving IVTA was significantly reduced at 24 h (−5.2%) and 12 weeks (−8.2%) (P < 0.01 for both). Eyes receiving single injections of bevacizumab experienced significant decreases in SFCT from 24 h to 4 weeks, but not at 8 or 12 weeks. The authors suggest that the presence of inflammatory factors other than VEGF may be responsible for the differences between IVTA and bevacizumab observed after 4 weeks. A more likely explanation is that the shorter intraocular half-life of bevacizumab produces a duration of action of less than 8 weeks.
A 12-month randomized, prospective trial compared 3 monthly injections of bevacizumab (2.5 mg) with a single injection of IVTA (8 mg) followed by monthly and q4month PRN injections, respectively [53]. Eyes in both the bevacizumab and triamcinolone arms experienced improved BCVA (0.30–0.23 logMAR for bevacizumab vs. 0.32–0.26 logMAR for triamcinolone) and reduced macular thickness (505–358 μm for bevacizumab vs. 490–308 μm for triamcinolone) at 3 months. Visual acuity continued to improve through 12 months in the bevacizumab group (0.18 LogMAR) but had decreased in the triamcinolone group (0.36 LogMAR) because of cataracts.
The efficacy of IVTA was evaluated in 20 eyes that had been unresponsive to at least 3 monthly intravitreal bevacizumab injections [46]. At least 2 months after the last intravitreal bevacizumab, an aqueous humor sample was obtained, and vascular endothelial growth factor, IL-2, IL-6, IL-8, tumor necrosis factor-α, and transforming growth factor-β2 concentrations were measured with a multiplex cytokine array. Triamcinolone was injected intravitreally, and BCVA and central subfield thickness were evaluated through 3 months. The mean BCVA was 47.1 ± 18.9 letters at baseline, 53.3 ± 19.7 letters at 1 month (P = 0.002), and 52.4 ± 19.1 letters at 2 months (P = 0.041), but the acuity gains were not sustained at 3 months (50.9 ± 18.6 letters; P = 0.204). A mean decrease in central subfield thickness (−11%) was seen in 12 eyes at 1 month. Multivariate analysis showed that the intraocular concentration of IL-8 was an independent factor for anatomic response at 1 month (P = 0.006). The authors concluded that intravitreal triamcinolone may be an attractive treatment option for patients who have poor short-term responses to bevacizumab.
Diabetic macular edema may develop or worsen after panretinal photocoagulation (PRP) for PDR. In a prospective study of eyes with severe diabetic retinopathy, 91 eyes (46 eyes with DME; 45 eyes without DME) of 76 patients underwent PRP with IVTA (30 eyes), PRP with intravitreous bevacizumab (31 eyes), or PRP alone (30 eyes) [19]. The primary outcome measures included changes in BCVA and central macular thickness (CMT) at 1 and 3 months. Secondary outcome measures were the proportions of eyes with BCVA gain or loss, and decreased or increased CMT. In eyes with DME, there was significant worsening in BCVA (P = 0.031) in the PRP group but significant improvement in BCVA (P = 0.012) in the IVTA group. In eyes without CSME, those receiving PRP alone experienced significant worsening in BCVA from 0.18 to 0.26 at 1 month (P = 0.008) and to 0.27 at 3 months (P = 0.005). In eyes without DME, there was significant increase in CMT from 209.75 to 259.00 μm at 1 month (P = 0.023) and to 276.14 μm at 3 months (P = 0.011) in the PRP group; in eyes with DME, the proportion of eyes with improved BCVA and decreased CMT was significantly higher in the IVTA group (75% and 100%, respectively) than in the IVB group (37.5% and 62.5%, respectively). The authors concluded that IVTA and bevacizumab may be effective adjunctive treatments to PRP by minimizing the risk of PRP-induced macular edema and visual loss.
The DRCR.Net Protocol I trial randomized 854 patients to receive ranibizumab + prompt laser, ranibizumab + deferred laser, IVT + prompt laser or prompt laser + sham. At 1 year, the median improvements in BCVA in each of the four treatment arms were +9, +9, +4, and +3 letters, respectively [26]. Patients receiving triamcinolone experienced BCVA improvements during the first 3 months that were similar to patients receiving ranibizumab, but BCVA then worsened through 12 months because steroid-related cataracts developed. One-year BCVA improvements in the triamcinolone subgroup that was pseudophakic at baseline were comparable to those in the ranibizumab groups.
Eyes randomized to prompt laser + triamcinolone were eligible to receive ranibizumab as early as week 74 for persistent DME with no improvement in BCVA. At the 5-year concluding visit, mean improvements in BCVA in the four arms were +10, +8, +7, and +5 letters [31]. During the first 2 years of the trial, pseudophakic eyes that were randomized to IVT + laser had better improvements in BCVA compared to the entire group receiving triamcinolone. By the 5-year visit, however, the BCVA gains in the pseudophakic eyes receiving triamcinolone resembled those in the entire triamcinolone arm.
5.5 Dexamethasone
The dexamethasone posterior-segment drug delivery system (DEX, Ozurdex®, Allergan Inc., Irvine, CA, USA) is a biodegradable, sustained-release reservoir. The DEX contains 0.7 mg of micronized, preservative-free dexamethasone in a biodegradable cylinder (6 mm long by 0.46 mm diameter) made of a copolymer of poly (lactic-co-glycolic) acid that slowly breaks down into glycolic acid and water while simultaneously releasing dexamethasone over the course of 3 months. The cylinder is preloaded into a single-use applicator and then injected across the pars plana into the anterior vitreous through a 22-gauge needle. The needle is advanced through the sclera in a tunneled fashion, and the tract self-seals after removal. The procedure is performed in the clinic under aseptic conditions after the instillation of topical and subconjunctival anesthesia.
A prospective, comparative study showed that pain after dexamethasone injection under topical anesthesia was comparable to that after anti-VEGF injections that were delivered with much smaller needles [64]. The insert should not be injected into aphakic and pseudophakic eyes that lack an intact barrier between the vitreous and anterior chamber because it can migrate into the anterior chamber and damage the corneal endothelium [49, 51, 58]. After injection, the insert usually settles into the inferior vitreous base without being noticed by patients. After approximately 3 months, the insert dissolves completely, and intraocular dexamethasone concentrations fall to near zero. In addition to rare cases of endophthalmitis and vitreous hemorrhage, injections have been complicated by inadvertent insert placement into the crystalline lens [7, 21, 32] or fragmentation of the insert [1, 27, 71, 72].
Drug release from the insert follows a biphasic pattern with intraocular concentrations peaking at 2–6 weeks (vitreous, 100–1000 μg/mL; retina, 100–1000 μg/gm), followed by a sharp drop during the third month, a lower plateau for 3–4 months (vitreous, 0.1–1 ng/mL; retina, 0.1–1 ng/gm), and non-detectable levels by months 7–8 [17]. The intraocular dexamethasone concentrations are higher and steadier during the initial plateau than can be achieved with topical [83], subconjunctival [81], periocular [84], and oral administration [82].
Previous vitrectomy accelerates clearance of drugs from the eye, but rabbit studies show that time-related intravitreal dexamethasone concentrations after the injection of the insert were the same in vitrectomized and non-vitrectomized eyes [18]. The CHAMPLAIN study was a prospective, open-label, 26-week trial of DEX in 55 eyes with DME that had previously undergone vitrectomy. At 8 weeks after injection, the mean BCVA improved by +6.0 letters, with 30.4% improving by >10 letters, and mean CRT changed by −156 μm [9]. These improvements were similar to those achieved in non-vitrectomized eyes in the phase III MEAD trial [10], suggesting that DEX functions well following vitrectomy.
Plasma dexamethasone concentrations after intravitreal injection of the DEX are below the lower limit of quantification (50 pg/mL), so the likelihood of patients developing systemic corticosteroid-related effects is very low [28].
In the ORVO study [14], protein arrays were performed on aqueous samples taken before and 4 weeks after a dexamethasone insert. In many patients, aqueous hepatocyte growth factor and EG-VEGF reductions at 4 weeks mirrored the reduction in macular edema.
Important trials that featured dexamethasone inserts are detailed in Table 5.3.
Table 5.3
This table lists the important diabetic macular edema trials with the dexamethasone insert and details their key findings
Important diabetic macular edema trials with the dexamethasone insert | ||
---|---|---|
Trial and phase | Cohorts | Key findings |
Macular edema trial 171 patients | Treatment arms DEX 700 μg DEX 350 μg Observation | At 90 days 1. Proportions of patients improving by 10 letters were 33%, 21%, and 12% (P = 0.007) 2. Greater improvements in central macular thickness in DEX 700 μg compared to laser (P = 0.03) At 6 months 1. Proportions of patients improving by 10 letters were 30%, 19%, and 23% |
PLACID trial Phase II | Treatment arms DEX + laser Laser | More patients receiving DEX + laser improved by 10 letters at 1 month (P < 0.001) and 9 months (31.7% vs. 17.3%; P = 0.0076) but not at 12 months Area under the curve analysis showed greater BCVA improvements in DEX + laser group |
CHAMPLAIN trial open-label 55 patients | Single-arm DEX in patients with previous vitrectomy | Mean change in central retinal thickness was −156 μm at 8 weeks (P < 0.001) but only −39 μm at 26 weeks (P = 0.004) Mean change in BCVA was +6 letters at 8 weeks (P < 0.001) but only +3 letters at 26 weeks (P = 0.046) |
MEAD trials Phase III 253 patients | Treatment arms DEX 700 μg DEX 350 μg Sham | At 3 years 1. More patients receiving the DEX improved by at least 15 letters (22.2%, 18.4%, 12.0%; P < 0.018) 2. Mean improvement in BCVA in pseudophakic eyes receiving DEX was +7 letters 3. 29.7% of DEX group had IOP >25 mmHg, but only one patient required incisional glaucoma surgery 4. 66% of DEX group developed cataracts and 61% underwent surgery |
In an initial 6-month trial, single applications of 0.35 and 0.7 mg dexamethasone inserts were performed in eyes with macular edema due to diabetic retinopathy, retinal vein occlusions, posterior uveitis, and the Irvine-Gass syndrome [54]. A subset analysis showed that patients with uveitis and Irvine-Gass responded equally well to the insert compared to eyes with the other diagnoses [85].
Patients in this trial with DME (n = 171) were fully reported in a separate analysis [42]. Enrollment criteria for this subgroup included patients with DME and baseline BCVA measurements from 20/40 to 20/200. Key exclusion criteria included a history of vitrectomy, moderate or severe glaucoma, poorly controlled systemic arterial hypertension (systolic blood pressure >160 mmHg and/or diastolic blood pressure >90 mmHg), and poorly controlled diabetes mellitus (HbA1c >13%). Patients were randomized 1:1:1 to receive the high-dose insert (700 μg), the low-dose insert (350 μg), or observation. The insert (20-gauge) was inserted through the pars plana with suturing of the incision sites. Eyes that lost 15 letters of BCVA were eligible for whatever treatment was proposed by the investigator. The primary outcome measure for these groups was a +10-letter improvement in BCVA at day 90. Additional outcome measures included the proportion of eyes improving by at least +15 letters, changes in dye leakage on fluorescein angiography, reduction in CRT, and safety measures.
At day 90, more patients in the group receiving the 700 μg insert than the 350 μg insert or observation groups experienced 10-letter improvements in BCVA (33.3% vs. 21.1% vs. 12.3%) (P = 0.007, 700 μg group vs. laser). At day 180, the corresponding +10-letter BCVA improvements were 30%, 19%, and 23% (P >0.4 for treated vs. observed eyes). At day 60, more patients receiving the 700 μg insert than observation achieved +15-letter improvements (P = 0.01). There were significantly greater improvements in CRT for treated vs. observed eyes (P = 0.03 at day 90). A dose-response trend was noted at all time points, but there were no significant differences between the 700 and 350 μg DEX arms.
Compared to the observation group at day 90, patients receiving the 700 μg DEX had significantly greater improvements in CRT (+30.2 μm vs. −132.3 μm) and fluorescein dye leakage. Four patients in the observation group and two in the 350 μg DEX group were treated with rescue laser or triamcinolone, but none in the 700 μg DEX group.
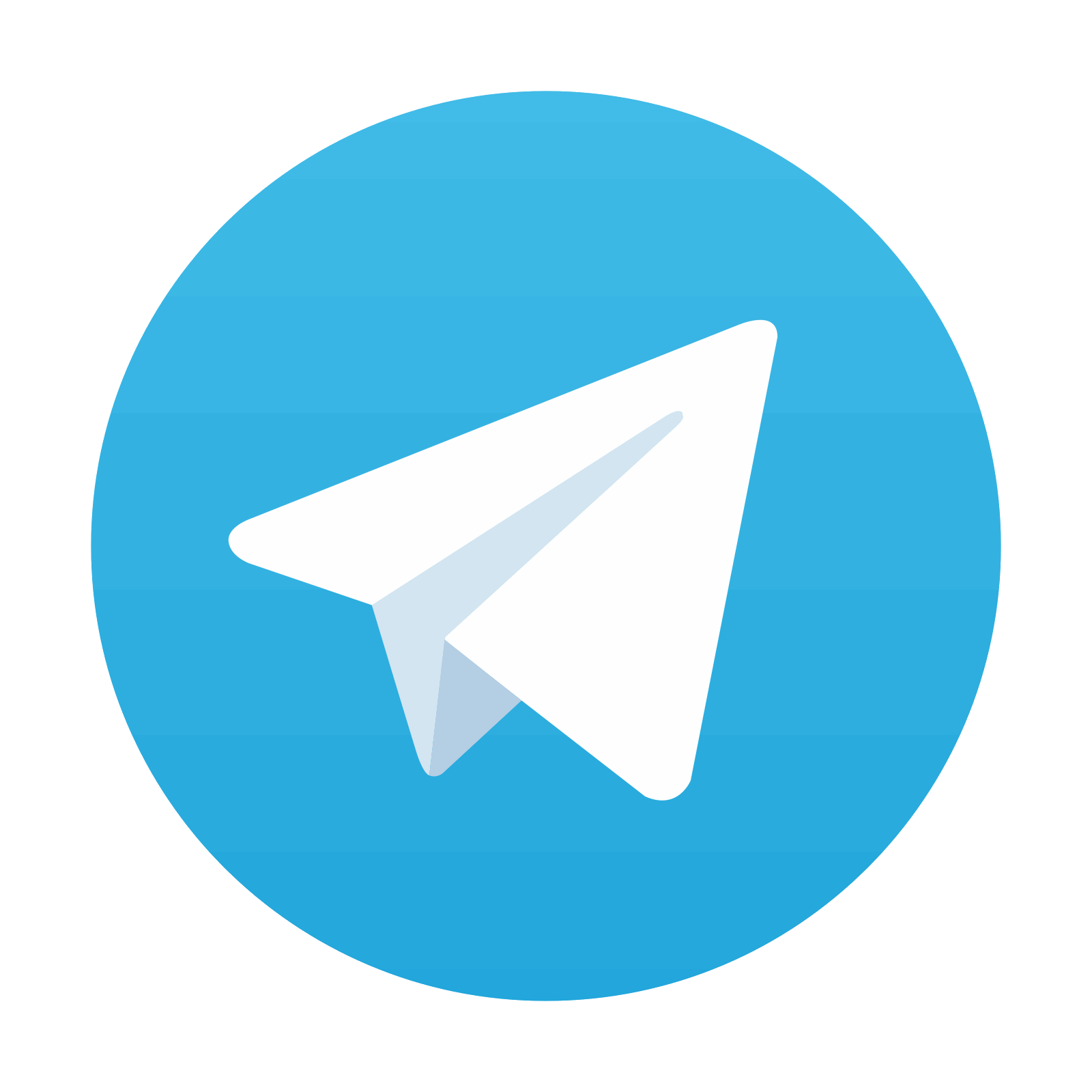
Stay updated, free articles. Join our Telegram channel

Full access? Get Clinical Tree
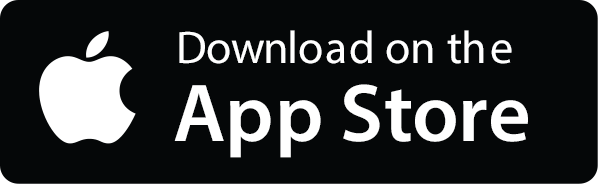
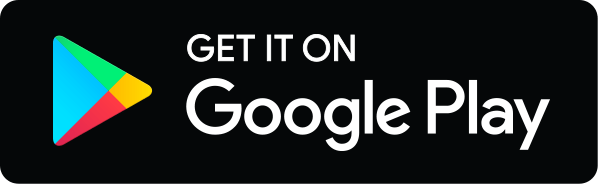