Abstract
Bleeding symptoms presenting in the neonatal period usually present a diagnostic and therapeutic challenge for treating physicians. Bleeding disorders may be due to either congenital or acquired coagulation disorders, and may be related to mortality or long term morbidity when not appropriately and timely diagnosed. While severe congenital coagulation defects usually present in the first hours to days of life with distinct symptoms in otherwise well newborns, acquired coagulation disorders usually present in sick newborns with a variety of presentations and distinct etiologies that differ from older children and adults. In newborns, the diagnosis of coagulation abnormalities based upon plasma concentrations of components of the hemostatic system requires age-appropriate reference ranges because plasma concentrations of several procoagulant and inhibitor proteins are physiologically decreased at birth. The aim of this chapter is to discuss clinical presentation, diagnosis, and management of the most common congenital and acquired bleeding disorders in newborns, excluding platelet disorders.
Introduction
Bleeding symptoms presenting in the neonatal period usually present a diagnostic and therapeutic challenge for treating physicians. Bleeding disorders may be due to either congenital or acquired coagulation disorders, and may be related to mortality or long-term morbidity when not appropriately and timely diagnosed. While severe congenital coagulation defects usually present in the first hours to days of life with distinct symptoms in otherwise well newborns, acquired coagulation disorders usually present in sick newborns with a variety of presentations and distinct etiologies that differ from older children and adults. In newborns, the diagnosis of coagulation abnormalities based upon plasma concentrations of components of the hemostatic system requires age-appropriate reference ranges because plasma concentrations of several procoagulant and inhibitor proteins are physiologically decreased at birth. The aim of this chapter is to discuss clinical presentation, diagnosis, and management of the most common congenital and acquired bleeding disorders in newborns, excluding platelet disorders.
General Information
Developmental Hemostasis
Components of the hemostatic system are already synthetized by the fetus starting at 10 weeks’ gestational age. At birth, all factors of the coagulation and fibrinolytic system are present and measurable. However, the concentration of several factors differs significantly from older children and adults. In the coagulation system, plasma concentrations of the vitamin K-dependent factors (F), contact factors, and the capacity to generate thrombin are decreased in newborns as compared to adults, while other factors such as fibrinogen, FV, FVIII, and FXIII are similar or increased at birth [1–5]. Plasma concentrations of the inhibitors antithrombin, heparin cofactor II, protein C, and protein S are decreased at birth up to 50% of older children and adult values. By contrast, the plasma concentration of α2-macroglobulin in newborns is increased approximately twice compared to adult values. In the fibrinolytic system, plasma concentrations of plasminogen, tissue-plasminogen activator, α2-antiplasmin (α2-AP) and plasminogen activator inhibitor-1 (PAI-1) are also significantly decreased in newborns compared to adults [1–3]. All these specific age-dependent features of the hemostatic system are considered physiological and do not predispose newborns to an increased risk of hemorrhage. However, these differences may hamper the diagnosis of a bleeding disorder in newborns or even lead to misclassification of coagulation defects when reference ranges of coagulation established for adults are used to interpret laboratory results of newborns with bleeding disorders. For this reason, accurate interpretation of coagulation tests in newborns can only be performed using age-dependent reference ranges, keeping in mind that these reference ranges may vary depending on the reagent and analyzer used in the laboratory [4, 6].
Clinical Presentation
Unexplained bleeding symptoms in otherwise healthy full-term newborns should be carefully investigated because it may reflect the presence of a severe congenital factor deficiency. Bleeding manifestations include persistent oozing from the umbilical stump, bleeding into the scalp resulting in large cephalohematomas, subdural hemorrhage, bruising, soft-tissue hemorrhage, bleeding following circumcision, persistent bleeding from puncture sites or, more rarely, gastrointestinal bleeding. Bleeding into joints, which is typical for older infants with severe hemophilia A or B, rarely occurs in newborns.
A small but important proportion of newborns present with an intracranial hemorrhage (ICH) as the first manifestation of their bleeding tendency, and a major cause of morbidity such as neurological impairment and developmental delay [7]. Intracranial hemorrhage can occur in both full-term and premature newborns and in a variety of locations. Intracranial hemorrhage in full-term newborns is rare and usually occurs either spontaneously or secondary to a variety of insults such as birth asphyxia, trauma, vitamin K (VK) deficiency, disseminated intravascular coagulation (DIC), and several congenital factor deficiencies [8–9]. The reported incidence of ICH in newborns with severe hemophilia A or B ranges between 3% and 4%. This incidence is significantly increased compared to the one reported in non-hemophiliac newborns ranging from 0.03% after elective caesarean to 0.1% after vacuum extraction [10–12]. Other rarer inherited factor deficiencies possibly causing ICH include FV, FVII, FX, FXI, and FXIII. Non-hematological causes such as arteriovenous malformations also need to be considered in neonates who have spontaneous ICH.
The characteristic form of ICH in premature newborns is an intraventricular hemorrhage (IVH) which is characterized by bleeding from the fragile microvasculature of the subependymal germinal matrix that may extend into the lateral ventricles and/or brain parenchyma [13–17]. Approximately 20% to 40% of premature infants born before 32 weeks’ gestational age or with a birth weight less than 1,500 grams develop an ICH [14]. Most IVHs occur in the first 24 hours, with almost all developing by 72 hours of life [14, 18]. The pathophysiologic mechanism of IVH in premature infants is incompletely understood and likely multifactorial [19]. Abnormalities of cerebral blood flow resulting in ischemia, and subsequent reperfusion of brain tissue are the most likely causes. Other potential contributing mechanisms include the fragility of the germinal matrix capillaries, oxidative damage to the endothelium, and concurrent impairment of the coagulation system including decreased plasma concentrations of some coagulant proteins, thrombocytopenia, and enhanced local fibrinolytic activity [20–21]. Factors such as vaginal delivery, labor, intrapartum asphyxia, respiratory distress syndrome, increased mean diastolic and systolic blood pressure, and decreased superior vena cava flow due to an immature myocardium have been associated with IVH in premature newborns [22–23]. The role of VK deficiency in the development of IVH has been assessed in three randomized controlled trials evaluating the role of VK administration to mothers during pregnancy in preventing IVH in newborns. Two demonstrated benefit and one did not [24–26].
Laboratory Investigations
Bleeding symptoms in newborns require prompt and accurate laboratory investigations to ensure appropriate treatment and possibly avoid long-term morbidity. A stepwise approach including coagulation screening tests (prothrombin time (PT), activated partial thromboplastin time (aPTT), and fibrinogen) followed by specific coagulation protein assays as indicated by the screening test results is recommended. Depending on the clinical circumstances, if screening tests are normal, factor XIII deficiency must still be excluded. This approach of stepwise evaluation of otherwise healthy newborns with bleeding symptoms and abnormal coagulation screening tests is depicted in Table 18.1.
Table 18.1 Evaluation of otherwise healthy newborns with bleeding symptoms and abnormal coagulation screening tests
Type or location of bleeding | Abnormal tests | No abnormal tests | |||
---|---|---|---|---|---|
PT | aPTT | PT + aPTT | PT + aPTT + fibrinogen | ||
Umbilical stump | FVIII, FIX, (vWF) | FV, FX | Fibrinogen | FXIII, α2-AP | |
After circumcision | FVIII, FIX, FXI | Fibrinogen | |||
Bruising | VK deficiency | FX | |||
Soft-tissue | (vWF) | FV | Fibrinogen | FXIII | |
Gastrointestinal | VK deficiency | FVIII, FIX | FII, FV, FX | ||
Puncture sites | VK deficiency | FVII, FIX, (vWF) | FX | Fibrinogen | |
Cephalhematoma | FVIII, FIX | ||||
Intracranial | FVII, VK deficiency | FVIII, FIX, FXI, (vWF) | FII, FV, FX | FXIII |
In newborns, the diagnosis of many congenital factor deficiencies based upon plasma concentrations can be difficult because plasma concentrations of several coagulation proteins are physiologically decreased at birth (see Table 18.2) [1–5]. Mild to moderate congenital deficiencies of prothrombin, FV, FVIII, FIX, FX, and FXI result in plasma concentrations that may overlap with neonatal physiological values. By contrast, plasma concentrations resulting from either mild to moderate FVII deficiency or severe deficiency of FV, FVII, FVIII, FIX, FX, and FXIII can be easily distinguished from physiologic values (see Table 18.2).
Table 18.2 Plasma concentrations of coagulation proteins in full-term healthy newborns and in congenital factor deficiencies
Factor | Plasma concentration (U/ml) | ||
---|---|---|---|
At birth | By factor deficiency | ||
Severe | Mild–moderate | ||
II | 0.27–0.64 | – | <0.01–0.20 |
V | 0.34–1.45 | <0.01 | 0.02–0.20 |
VII | 0.28–1.43 | <0.01 | 0.01–0.15 |
VIII | 0.50–1.78 | <0.01 | 0.01–0.50 |
IX | 0.15–0.91 | <0.01 | 0.01–0.50 |
X | 0.12–0.87 | <0.01 | 0.01–0.15 |
XI | 0.10–0.87 | – | <0.01–0.70 |
XIII | 0.11–0.93 | <0.01 | – |
Abbreviations: units per milliliter (U/ml)
Prenatal diagnosis of most congenital factor deficiencies is performed by amniocentesis or chorionic villus biopsy. The prenatal diagnosis of congenital deficiencies of specific coagulation proteins is largely confined to severe hemophilia A and B although deficiencies of FV, FVII, FXIII, and vWF (von Willebrand factor) have also been diagnosed prenatally [27]. Prenatal diagnosis of vWF deficiency is only indicated for type 3 or severe von Willebrand disease (vWD) [28–29]. Recently, droplet digital PCR and targeted massively parallel sequencing for maternal plasma DNA analysis was developed to noninvasively determine fetal mutational status in pregnancies at risk for hemophilia A and B [30]. Early diagnosis permits either termination of the pregnancy or early intervention when indicated.
Congenital Hemorrhagic Disorders
Hemostatic Proteins
For congenital deficiencies of components of the hemostatic system, both a severe and a milder form occur. Severe congenital deficiencies of prothrombin, factor (F)V, FVII, FVIII, FIX, FX, FXI, fibrinogen, FXIII, and α2AP can all present with bleeding in the first days of life [31–33]. Mild congenital deficiencies of these proteins usually do not cause bleeding at birth in otherwise healthy full-term newborns. Although von Willebrand disease (vWD) is the most common bleeding disorder during childhood, only rare severe forms of vWF deficiency present with bleeding in newborns. Deficiencies of FXII, high molecular weight kininogen, and prekallikrein do not cause bleeding and are not discussed further.
Inheritance
Congenital deficiencies of prothrombin, FV, FVII, FX, FXI, FXIII, fibrinogen, and α2AP are inherited as autosomal recessive traits with consanguinity present in many families [34]. Deficiencies of FVIII (hemophilia A) and FIX (hemophilia B), the most common congenital bleeding disorders to present in newborns, are inherited as sex-linked recessive traits. Although vWF deficiency is predominantly inherited as an autosomal dominant pattern, cases of autosomal recessive inheritance have been reported [35–36]. Rarely, combined deficiencies of prothrombin, FVII, FIX, and FX, or FV and FVIII present in newborns [37–38].
Congenital Factor Deficiencies
The following provides specific information on the presentation and management of congenital factor deficiencies with an emphasis on severe forms.
Factor VIII Deficiency
Factor VIII is a heterodimer with a molecular weight of 330,000 consisting of a COOH-terminal-derived light-chain in a metal ion-dependent association with an NH2-terminal heavy-chain fragment. The gene for FVIII is located on the long arm of the human X chromosome in the most distal band Xq28 (see Table 18.3). Following activation by FXa or thrombin, FVIIIa acts as a cofactor for the FIXa-mediated activation of FX, which subsequently converts prothrombin to thrombin in the presence of FVa, negatively charged phospholipids, and calcium.
Factor | Chromosome | Molecular weight | Plasma concentration (μg/ml) | Half-life (hours) | Minimal hemostatic level |
---|---|---|---|---|---|
I | 4q26-q28 | 330,000 | 3,000 | 120 | 0.5–1.0 g/L |
II | 11p11.2 | 72,000 | 100 | 72 | 0.15–0.40 U/ml |
V | 1q21-q25 | 330,000 | 10 | 12–36 | 0.10–0.25 U/ml |
VII | 13q34 | 50,000 | 0.5 | 4–6 | 0.05–0.10 U/ml |
VIII | Xq28 | 330,000 | 0.1 | 12–15 | 0.30–0.50 U/ml |
IX | Xq27 | 56,000 | 5 | 18–30 | 0.20–0.50 U/ml |
X | 13q34 | 58,800 | 10 | 65 | 0.10–0.20 U/ml |
XI | 4q32-q35 | 160,000 | 5 | 65 | 0.10–0.30 U/ml |
XIII |
| 320,000 | 60 | 72–240 | 0.10–0.50 U/ml |
vWF | 12pter-p12 | 309,000 | 5–10 | 12 | 0.30–0.50 U/ml |
Abbreviations: micrograms per milliliter (μg/ml); grams per liter (g/L); units per milliliter (U/ml); factor (F); a subunit of FXIII (a); b subunit of FXIII (b); von Willebrand factor (vWF)
Severe FVIII deficiency (FVIII <1%) is the most common congenital coagulation disorder to present in newborns. A small percentage of newborns with moderate (FVIII 1%–5%) or mild (FVIII 5%–50%) FVIII deficiency may also present following an acquired hemostatic challenge [39]. Based upon recent cohort studies, 40% to 60% of children with severe hemophilia A are clinically symptomatic as newborns. A further 40% present by 1 year of age, and 50% have had a major bleed by 1.5 years [40–42]. Common presentations are ICH, muscle and mouth bleeding, cephalic hematoma, bleeding from the umbilical stump, and following circumcision [11, 43–46]. Rarer reported clinical presentations include bleeding in the adrenal gland, hematoma of the liver, splenic rupture, and radial artery pseudoaneurysm following radial artery puncture [47–49]. Although rare, severe hemophilia A may occur in females and present at birth. The plasma concentrations of FVIII in these female newborns is, in general, less than <0.01 U/ml.
Treatment
Currently, FVIII concentrates (plasma derived or recombinant) are the treatment of choice [50]. Recently, data from a randomized trial have shown that previous untreated children receiving plasma-derived factor VIII had a lower incidence of inhibitors than those treated with recombinant factor VIII [51]. From these data, newborns with hemophilia A requiring FVIII substitution should preferably receive plasma-derived factor VIII as first-line therapy. No data are available so far on the incidence of inhibitors in previous untreated patients treated with the new half-life extended FVIII concentrates. Alternative therapy to FVIII replacement has been used in newborns undergoing circumcision. In one study of 10 patients with severe hemophilia A, local fibrin glue was used instead of infusion of FVIII concentrate. Only two of three patients who bled postoperatively required FVIII concentrate [52]. Insufficient data are available to support the benefit of prophylactic doses of FVIII intrauterine or shortly after birth in newborns suspected or confirmed to have hemophilia in order to offset the trauma of labor and reduce the risk of ICH. The role of the new non-FVIII treatment options (Emicizumab, Fitusiran, Concizumab) as first line therapy in newborns with hemophilia A is still under debate.
Factor IX Deficiency
Human FIX is a vitamin K (VK)-dependent single-chain glycoprotein with a molecular weight of 56,000. The gene for FIX is located on the tip of the long arm of the X chromosome at position Xq27 (see Table 18.3). Following activation by FXIa or FVIIa complexed with tissue factor, FIXa complexed with FVIIIa on membrane surfaces activates FX, which subsequently converts prothrombin to thrombin.
Bleeding due to severe FIX deficiency (FIX <1%) in newborns occurs at the same sites as for FVIII deficiency [11, 41–46]. The diagnosis of mild FIX deficiency (FIX 5%–40%) in newborns may be difficult because of physiologically decreased FIX levels as low as 0.15 U/ml and the potential for concurrent VK deficiency in these patients (see Table 18.1).
Treatment
Plasma-derived and recombinant FIX concentrates are the replacement products of choice and preferable to prothrombin complex concentrates (PCC). No data are available so far on the incidence of inhibitors in previous untreated patients treated with the new half-life extended FIX concentrates.
Afibrinogenemia and Hypofibrinogenemia
The fibrinogen molecule is a dimer with a molecular weight of 330,000 consisting of three pairs of polypeptide chains named Aα-, Bβ, and γ-chain. These three chains are encoded by three separate genes located on chromosome 4 (see Table 18.3). Following enzymatic cleavage of fibrinopeptides by thrombin, fibrinogen is converted into insoluble fibrin inducing clot formation.
Fibrinogen deficiency is rare. Bleeding due to afibrinogenemia has been reported in newborns following circumcision, or as umbilical stump bleeding and soft tissue hemorrhage, and bleeding from puncture sites [52–56].
Treatment
Although fresh frozen plasma (FFP) can be used as initial therapy, cryoprecipitate or fibrinogen concentrates are preferable. Fresh frozen plasma at a dose of 10–20 ml/kg or cryoprecipitate at a dose of 1 U/5 kg of body weight will usually raise the plasma fibrinogen level 50 to 100 mg/dl and last for 4–5 days, reflecting the long plasma half-life of fibrinogen (see Table 18.3). One fibrinogen concentrate is available in Europe (Haemocomplettan HS, CSL Behring) and North America (Riastap, CSL Behring). The dose required for children depends on the type of bleeding and on the weight of the patient [52–56].
Prothrombin Deficiency
Prothrombin is a VK-dependent plasma glycoprotein with a molecular weight of 72,000. The gene for prothrombin is located on chromosome 11 at position 11p11.2 (see Table 18.3). Prothrombin is converted to thrombin by FXa in the presence of FVa, phospholipids and calcium.
Deficiency of prothrombin is very rare. Knockout mouse models demonstrated that life expectancy in unchallenged adults genetically depleted of prothrombin was about 5 to 7 days. The loss of viability was associated with the development of severe hemorrhagic events within multiple tissues, particularly in the heart and brain. These models suggested that the conditional loss of prothrombin is uniformly not compatible with maintenance of hemostasis or long-term survival [57]. Patients with prothrombin levels below 30%–40% may present with prolonged postinjury bleeding, mucosal bleeding, hematomas, and hemarthroses [58]. Bleeding complications due to prothrombin deficiency are reported in two newborns and consisted of gastrointestinal bleeding and ICH [59–61].
Treatment
Bleeding secondary to prothombin deficiency can be treated with FFP at a loading dose of 10–20 ml/kg followed by 3 ml/kg every 12–24 hours. Although PCC contains significant amount of prothrombin, administration of PCC should be carefully monitored because the presence of other VK-dependent factors in PCC may activate the coagulation cascade leading to thrombotic complications [34, 62].
Factor V Deficiency
Factor V is a single-chain glycoprotein with a molecular weight of 330,000. The gene for FV is located on chromosome 1 at position 1q21-25 (see Table 18.3). Following activation by thrombin or FXa, FVa acts as a cofactor for the FXa-mediated activation of prothrombin.
Bleeding due to severe FV deficiency has been reported in newborns. The clinical presentations include ICH, subdural hematoma, bleeding from the umbilical stump, gastric hemorrhage, and soft tissue hemorrhage [63–64]. Antenatal intraventricular hemorrhage (IVH) was reported in three newborns [65–67].
Treatment
Currently, only FFP is available as a replacement source for FV. When bleeding occurs infusions of FFP at an initial dose of 10–20 ml/kg, followed by infusions of 3 to 6 ml/kg can correct the bleeding defect (see Table 18.3). Platelet transfusion may provide an alternative source of FV and a valuable treatment in concomitant to FFP [34, 68]. While the administration of recombinant FVIIa (rFVIIa) (NovoNordisk) has been successfully used in patients with FV deficiency not responding to FFP, there have been no reports on the use of rFVIIa in neonatal hemorrhage so far.
Factor VII Deficiency
Factor VII is a VK-dependent single-chain zymogen with a molecular weight of 50,000. The gene for FVII is located on the long arm of chromosome 13 at position 13q34 (see Table 18.3). Factor VII is a component of the extrinsic pathway of blood coagulation. Following activation by binding to its cofactor, tissue factor, FVIIa catalyzes activation of additional FVII and activates both FX and FIX.
Severe FVII deficiency (FVII <1%) usually causes significant bleeding equivalent to that seen in patients with severe hemophilia, that manifests within the first 6 months of life. Patients with FVII levels of greater than 5% generally have mild hemorrhagic episodes. The most common reported bleeding complication in newborns with congenital FVII deficiency is ICH [69–71]. In a review of 75 patients with FVII deficiency, ICH was observed in 12 (16%) patients. In 5 (41%) out of these 12 patients, ICH occurred in the first week of life with fatal outcome [72]. Recently, a newborn with a severe ICH due to congenital FVII deficiency having a novel homozygous mutation of NM_000131.4 c.272_277 delins CCTCTC on 13th chromosome was reported [73]. Congenital FVII deficiency may occur in infants with Dubin–Johnson syndrome or Gilbert syndrome [74–76].
Treatment
Bleeding episodes due to FVII deficiency can be treated with FFP, PCC or FVII concentrates, either plasma-derived factor VII or recombinant factor VIIa depending upon availability. For severe hemorrhage, FFP should be administered at an initial dose of 20 ml/kg followed by a dose of 3 to 6 ml/kg repeated every 8 to 12 hours until healing occurs. Although FFP or PCC can be used as initial therapy, FVII concentrate has become the replacement product of choice. However, careful monitoring is required because of the risk of thromboembolisms (TEs) [34, 69, 77]. A recovery study of rFVIIa has been reported for a newborn with severe FVII deficiency and ICH. The drug was administered intravenously every 4 hours at doses of 15, 22, and 30 μg/kg. Factor VII:C was >100% between 30 and 180 minutes after each infusion with mean trough levels over 25% for all three doses. Infusions were well tolerated and maintained effective hemostasis with a good clinical outcome [79]. The successful treatment of intracranial hemorrhage in newborns with severe FVII deficiency using rFVIIa at doses ranging from 20 to 150 μg/kg every 4 to 6 hours has been described in several case reports [80–82]. In 1988, Daffos et al. used fetal blood sampling to diagnose FVII deficiency at 24 weeks’ gestational age and the fetus was transfused in utero at 37 weeks’ gestational age with 200 U of FVIIa concentrate. Subsequently the baby was born without hemorrhagic complications and did not bleed as a newborn [83].
von Willebrand Factor Deficiency
The von Willebrand factor is an adhesive multimeric glycoprotein with a molecular weight of 309,000. The gene for vWF is located on the short arm of chromosome 12 at position 12pter-p12 (see Table 18.3). Following simultaneous binding of vWF to the glycoprotein Ib receptor on platelet surfaces and to collagen in the subendothelium, vWF mediates the adhesion of platelets to the injured vascular wall. Platelet adhesion is mediated by the highest molecular weight multimers of vWF. In addition, vWF acts as a plasma carrier and stabilizing protein for FVIII.
von Willebrand disease includes partial or complete deficiency of vWF (type 1, and 3), qualitative variants with decreased platelet-dependent function (type 2A, and 2 M), qualitative variants with increased affinity for platelet glycoprotein Ib (type 2B), and qualitative variants with markedly decreased affinity for FVIII (type 2 N). Although vWD is the most common congenital bleeding disorder, patients rarely present as newborns because plasma concentrations of vWF are increased at birth with an increased proportion of high molecular weight multimers [84–85]. Bleeding due to vWF deficiency are rarely reported in newborns. Two infants with type 2B vWD presented with bleeding at blood sampling and soft tissue sites. Repeated platelet counts were less than 50 × 109/L, and the bleeding time and aPTT were prolonged. The vWF multimeric structure was characterized by the absence of the high molecular weight forms, and the patient’s platelets aggregated at much lower ristocetin concentrations than did controls [86]. One infant with type 2 A vWD presented with bleeding from the umbilical stump and later with life threatening epistaxis [87]. Two newborns with type 3 vWD presented at the age of 5 and 7 days respectively, with ICH and subdural hemorrhage [88–89].
Treatment
The goal of the therapy for vWD is to normalize platelet adhesion and increase plasma concentrations of FVIII. Although partially purified FVIII concentrates contain large amounts of vWF, not all of them are able to normalize platelet adhesion because they only contain low- and mid-molecular weight multimers [91]. Several partially purified FVIII concentrates containing some of the high-molecular weight vWF multimers that have been successfully used to treat bleeding in patients with vWD are available (Bayer, CSL Behring, Octapharma, Takeda). Both type 2 and type 3 vWD can present in newborns and may require treatment with FVIII-vW concentrates at a dose varying from 5 to 40 U/ml initially administered every 12 to 24 hours.
Factor X Deficiency
Factor X is a VK-dependent two-polypeptide-chain molecule with a molecular weight of 58,800. The gene for FX is located on the long arm of chromosome 13 at position 13q34 (see Table 18.3). Following activation by the FVIIa-tissue factor complex or the FIXa-FVIIIa complex, FXa converts prothrombin to thrombin in the presence of FVa, phospholipids, and calcium.
Bleeding complications due to severe FX deficiency have been reported in newborns. The most common clinical presentation was ICH, but other sites of bleeding were also reported including bleeding from the umbilical stump or from heel prick sites, gastrointestinal bleeding, scrotal hemorrhage, and spontaneous bruising [92–94]. Subdural hemorrhage was diagnosed antenatally in one newborn [95].
Treatment
Uncomplicated bleeding episodes can be treated with FFP at an initial dose of 20 ml/kg followed by a dose of 5–10 ml/kg every 24 hours until hemostasis occurs. For severe bleeding episodes, PCC can also be used at doses which depend upon the concentration of FX contained in the concentrate. However, repeated administration of PCC should be avoided because of the risk of TEs and disseminated intravascular coagulation (DIC). For similar reasons, high doses of PCC that increase FX levels by more than 50% of normal should also be avoided. Successful primary prophylaxis with PCC in four newborns presenting with bleeding within 24–72 hours of birth has been described by McMahon et al [96]. In all children, early prophylaxis prevented development of arthropathy and improved quality of life [34, 97]. A new human plasma-derived FX concentrate (Coagadex; BioProducts Laboratory, Elstree, UK) for the treatment of hereditary FX deficiency was approved in 2016 in the United States and Europe. Clinical studies have shown that administration of this concentrate at a dose of 25 IU/kg is safe and efficacious for on-demand treatment and short-term prophylaxis in patients with moderate-to-severe hereditary FX deficiency [98].
Factor XI Deficiency
Factor XI is a homodimer composed of two identical chains bound together by disulfide bonds, and with a molecular weight of 160,000. The gene for FXI is located on chromosome 4 at position 4q32-35 (see Table 18.3). Following activation by FXIIa, FXIa mediates the activation of FIX in the presence of calcium.
Deficiency of FXI is rare, and is different from other coagulation protein deficiencies in that bleeding symptoms do not necessarily correlate with plasma concentrations of FXI nor do all patients bleed [99]. Bleeding complications due to severe FXI deficiency are reported in two newborns. One newborn bled after circumcision at the age of 3 days, while another newborn was diagnosed prenatally with bilateral subdural hemorrhage [100–101].
Factor XIII Deficiency
Factor XIII is a tetramer consisting of two A subunits and two B subunits with a molecular weight of 320,000. The gene for the A subunit of FXIII is located on chromosome 6 at 6p24-25, while the gene for the B subunit is located on chromosome 1 at position 1q31-32 (see Table 18.3). The catalytic activity of FXIII resides in the A subunit. After binding to calcium, FXIII is converted to its activated form by thrombin during fibrin formation. Activated FXIII catalyzes the formation of peptide bonds between adjacent molecules of fibrin monomers, leading to a mechanical and chemical stability of the fibrin clot.
Severe FXIII deficiency typically manifests at birth with bleeding from the umbilical stump or ICH [102–105]. Other clinical presentations of homozygous FXIII deficiency include delayed wound healing, abnormal scar formation, and recurrent soft tissue hemorrhage with tendency to form hemorrhagic cysts. Intracranial hemorrhage occurs even in the absence of a trauma in approximately one-third of all affected patients [106]. Heterozygous newborns are not clinically affected.
Treatment
Fresh frozen plasma, cryoprecipitate as well as plasma derived (CSL Behring) or recombinant (NovoNordisk) FXIII concentrates can be used for the treatment of FXIII deficient patients. Newborns with FXIII deficiency should be placed on a prophylactic regimen of FXIII because of the high incidence of ICH. Plasma concentrations of FXIII over 1% are effective and the very long half-life of FXIII permits once a month therapy. Therefore, prophylactic replacement therapy is achieved either with little doses of FFP (2–3 ml/kg) administered every 4 to 6 weeks, cryoprecipitate at a dose of 1 bag/10–20 kg every 3 to 6 weeks or, preferably, with plasma-derived FXIII concentrate at a dose of 10 to 20 U/kg every 4 to 6 weeks depending on the clinical situation and the pre-infusion plasma concentration of FXIII [34, 106–107]. In the phase 3 trial evaluating the recombinant FXIII in patients older than 6 years with congenital FXIII-A subunit deficiency, a dose of 35 U/kg of rFXIII was safe and effective in preventing bleeding episodes [108].
Familial Multiple Factor Deficiencies
Congenital deficiencies of two or more coagulation proteins have been reported for 16 different combinations of coagulation factors [109–110]. Bleeding in the neonatal period has only been reported for two infants with combined factor deficiencies. One infant with FII, FVII, FIX, and FX deficiency presented with spontaneous bruising and bleeding from the umbilical stump which persisted until 3 months of age when the infant was treated with FFP [111]. A second infant with FV and FVIII deficiency presented with serious bleeding (undescribed) in the first week of life [112]. Multiple factor deficiencies, including combined FXI and FXII, FXI and FVIII, and FVIII, FXI, FXII and FXIII deficiencies, have been observed in patients with Noonan’s syndrome [113].
Treatment
Initial therapy is usually with FFP. Subsequent treatment will vary depending upon the specific factors affected.
Alpha2-Antiplasmin Deficiency
Alpha2-antiplasmin is a single-chain glycoprotein with a molecular weight of 63,000 and a plasma half-life of approximately 60 hours. The gene for α2-AP is located on chromosome 18 at position p11.1-q11.2. Alpha2-antiplasmin is a major inhibitor of the fibrinolytic cascade by forming an irreversible inactive complex with plasmin.
Alpha2-antiplasmin deficiency is a rare congenital disorder that presents with normal coagulation screening tests. A common presentation is bleeding from the umbilical stump [114]. Reports of older children and adults describe bleeding in the central nervous system, epistaxis, hemarthrosis, spontaneous intramedullary hematoma, and excessive bleeding during surgery or following minor trauma [115–117].
Treatment
Successful administration of antifibrinolytic agents including tranexamic acid and epsilon-aminocaproic acid have been reported to treat bleeding episodes or as prophylaxis during surgical procedures in adult patients with α2-AP deficiency [118]. Tranexamic acid was administered by continuous infusion or either hourly infusion or orally during 14 days by procedures [119]. No data on dose requirements of antifibrinolytic agents in newborns and children with α2-AP deficiency are available.
Acquired Hemorrhagic Disorders
The most common causes of acquired hemorrhagic disorders in newborns are VK deficiency, DIC, and liver disease.
Vitamin K Deficiency
Mechanism of Action of Vitamin K
Vitamin K is a cofactor essential for the γ-carboxylation process of the VK-dependent factors, FII, FVII, FIX, FX, and inhibitor proteins C and S. These proteins bind calcium through the gamma carboxyl groups permitting subsequent concentration on negatively charged phospholipid surfaces. The lack of functional activity for VK-dependent coagulation proteins causes bleeding which is reversible with VK therapy.
Sources of Vitamin K
Sources of VK in humans are the diet that provides VK1 (phytonadione) which is particularly present in leafy green vegetables and intestinal bacteria, which synthesize VK2 (menaquinone). Newborns are at increased risk of VK deficiency due to poor placental transfer of VK (10%) during pregnancy, insufficient bacterial colonization of the colon at birth, and inadequate dietary intake of VK in breast-fed babies [120–122]. Finally, drugs including oral anticoagulants, anticonvulsants, rifampicin, or isoniazid administered to mothers during pregnancy can interfere with either VK storage pool or VK function in fetus and newborns [123–125].
Clinical Presentation of Vitamin K Deficiency
Bleeding due to VK deficiency can be classified into three patterns based on the timing and type of complications [126]. A first, early pattern of VK deficiency bleeding (VKDB) is rare and presents in the first 24 hours of life with serious bleeding including ICH, intrathoracic or intra-abdominal bleeding. This early pattern usually manifests in newborns whose mothers have been taken VK antagonists, certain anticonvulsants, or antituberculous drugs. Cases of fetal extensive intracranial hemorrhage due to latent maternal vitamin K deficiency due to severe eating disorder and recurrent vomiting since early pregnancy were reported [127–128]. A second, classic pattern of VKDB presents on days 1 to 7 of life in breast-fed infants, with nose or gastrointestinal bleeding, bruising, bleeding from puncture sites or umbilical stump, or following circumcision. The third, late pattern of VKDB presents beyond the first week of life in breast-fed infants and is usually associated with a variety of diseases that compromise the supply of VK such as diarrhea, cystic fibrosis, α1-antitrypsin deficiency, hepatitis, coeliac disease. and other rare disorders. The late pattern can also occur when recommended prophylactic vitamin K is not administered [129]. This late pattern is characterized by bruising, gastrointestinal bleeding or ICH.
Laboratory Evaluation of Suspected Vitamin K Deficiency
The laboratory tests for detecting the presence of VK deficiency include screening tests (PT and aPTT), and specific factor assays (FII, FVII, FIX, FX) (see Table 18.1). Patients with VK deficiency produce decarboxylated forms of the VK dependent factors (PIVKA) which can be measured directly, or as a discrepancy between coagulant activity and immunological concentration [130–131].
Treatment of Vitamin K Deficiency
Any patient suspected to have VK deficiency should be treated immediately with VK while awaiting laboratory confirmation. The route and specific type of therapy is dictated by the urgency of the clinical situation and potential side effects of therapy.
Bleeding due to VK deficiency should be treated with intravenous or subcutaneous VK at doses appropriate for age. Depending on the severity of bleeding, 10 to 20 ml/kg of stored plasma or FFP should be given. Fresh frozen plasma is particularly useful when the precise nature of the coagulopathy is unknown. Prothrombin complex concentrates can be administered to newborns with life threatening bleeding or ICH in alternative to FFP. However, administration of PCCs requires careful monitoring because of the risk of thromboembolic complications. The amount of plasma required to totally correct a severe VK deficiency is so large that it may result in volume overload. The successful administration of rFVIIa has been reported in five newborns with ICH due to VK deficiency. In all patients, rapid correction of coagulopathy allowed early neurosurgical intervention, and no bleeding extension was observed after infusion of rFVIIa at doses ranging between 90 and 200 µg/kg (see Table 18.4) [128, 132–134].
Table 18.4 Newborns with vitamin K deficiency bleeding treated with rFVIIa
Publication | Age (weight) | Clinical presentation | Dose of rFVIIa (µg/kg) | Outcome |
---|---|---|---|---|
Brady et al. [132] |
|
|
|
|
Flood et al. [134] | 5 weeks | Subdural hemorrhage | 200 | Rapid correction of coagulopathy. No bleeding extension |
Hubbard et al. [129] |
|
|
|
|
Prophylactic Vitamin K Administration
Two major randomized controlled trials assessed the benefits of VK prophylaxis using clinical bleeding as the outcome measure [135–136]. In the first study, 3338 full-term infants were randomized to receive either placebo, or menadione (100 μg or 5 mg) intramuscularly. Healthcare personnel, who were unaware of the type of therapy, assessed minor and major bleeding outcomes. The incidence of ICH as well as minor bleeding was significantly increased in the placebo group compared to both treatment arms. This clinical result was supported by laboratory tests showing that the PT was prolonged in infants with hemorrhagic complications and corrected when VK was given. In the second study, 470 infants were randomized to receive VK or nothing. The study population consisted of male infants who were undergoing circumcision. Bleeding following circumcision was observed in 32 out of 230 infants not receiving VK compared to 6 out of 240 infants treated with VK. The same investigators reproduced these results in a subsequent, smaller, non-blinded trial [137].
The benefit of prophylactic VK in neonates has also been demonstrated by several epidemiological trials conducted in different countries. Independently of the administration route and dosing regimen used, VK prophylaxis at birth clearly protects newborns against classical VKDB [138]. However, for the prevention of late VKBD, some controversy still exists with regard to the mode of application and dosing. While oral administration of a single dose of 1 mg VK at birth protects against early and classical VKDB, it seems indeed not effective in preventing late VKDB [138–139]. Repeated or continuous oral VK supplementation was shown to be as effective as intramuscular prophylaxis but requires appropriate compliance, and may not fully protect infants with cholestatic disease from late VKDB [139–140]. The efficacy of oral VK prophylaxis may be improved by the use of a new mixed-micellar, water-soluble VK formulation [141–142]. Current recommendations for VK prophylaxis in healthy newborns in different countries are listed in Table 18.5. In 2016, the ESPGHAN Committee on Nutrition published a position paper to provide recommendations for setting up local guidelines for the prevention of VKDB in newborns and infants [143]. The group recommends that healthy newborn infants should either receive 1 mg of vitamin K1 by intramuscular injection at birth; or 3 × 2 mg vitamin K1 orally at birth, at 4 to 6 days and at 4 to 6 weeks; or 2 mg vitamin K1 orally at birth, and a weekly dose of 1 mg orally for 3 months. Intramuscular application is the preferred route for efficiency and reliability of administration. The oral route is not appropriate for preterm infants and for newborns who have cholestasis or impaired intestinal absorption or are too unwell to take oral vitamin K1, or those whose mothers have taken medications that interfere with vitamin K metabolism [143].
Table 18.5 Current recommendations for vitamin K prophylaxis in healthy newborns in different countries
Country | Recommended vitamin K prophylaxis |
---|---|
Australia |
|
Canada |
|
Germany | All newborns: 2 mg oral on day 1, day 4 to 10, and day 28 to 42 |
Switzerland | All newborns: 2 mg* oral at 4 hours, 4 days, and 4 weeks |
USA | All newborns: 1–2 mg IM on day 1 |
Note: * Mixed-micellar VK preparation.
Abbreviations: milligram (mg); gram (g); intramuscular (IM); United States of America (USA).
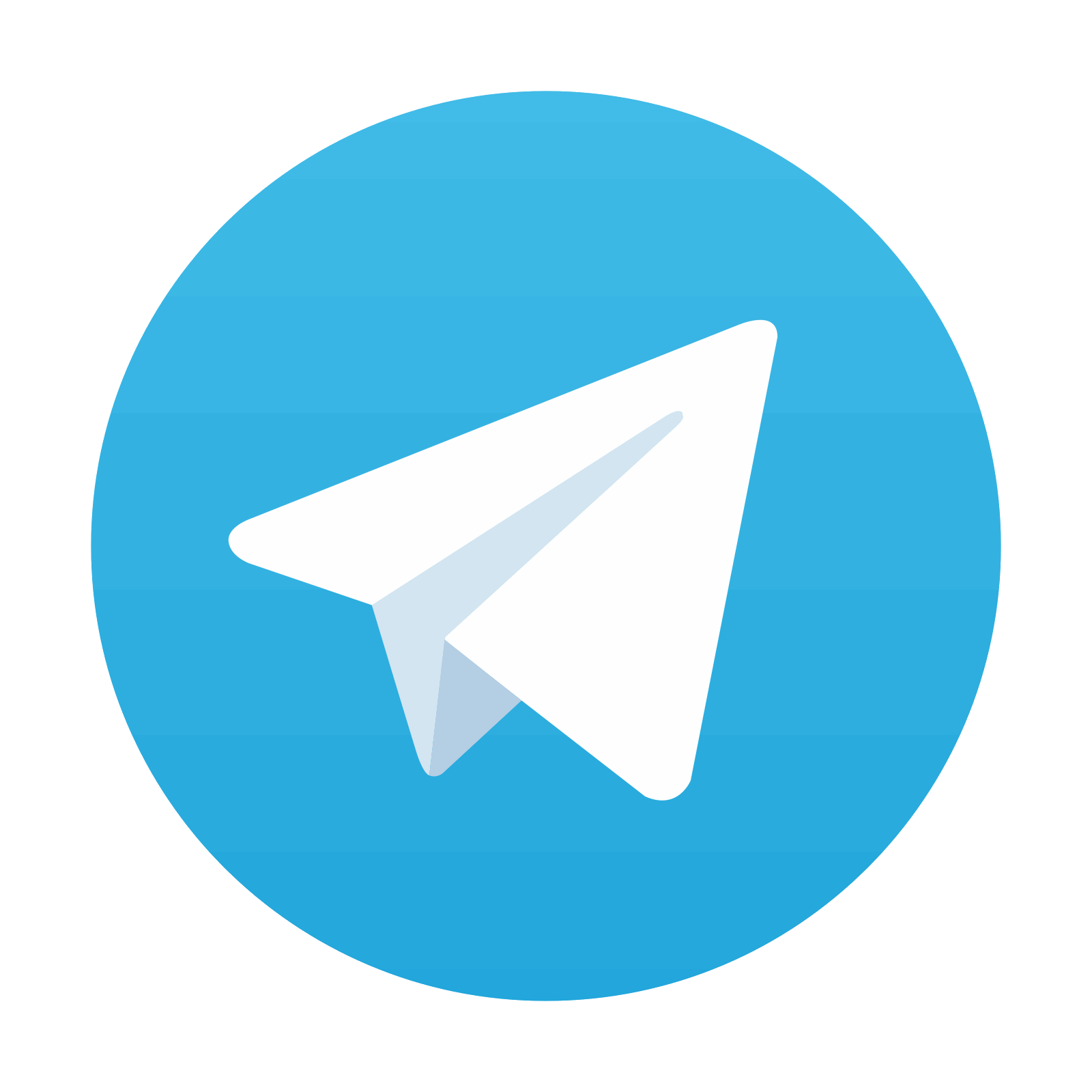
Stay updated, free articles. Join our Telegram channel

Full access? Get Clinical Tree
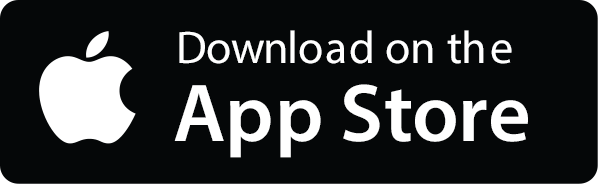
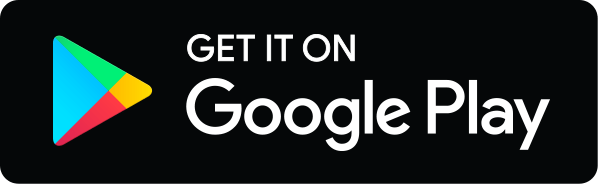
