Abstract
In addition to quantitative neutrophil abnormalities, innate immunity, and thus risk of infection in a neonate, may be negatively impacted by qualitative phagocyte defects. The term phagocyte stems from the Greek “phagein” meaning “to eat or devour” and “cyte” meaning “cell” and refers to hematopoietic derived cells, namely monocytes, macrophages and neutrophils capable of engulfing and digesting microorganisms, foreign particles, and cellular debris. Neutrophils are also classified as granulocytes, given the characteristic presence of granules in their cytoplasm that play a key role in neutrophil function.
Introduction
In addition to quantitative neutrophil abnormalities, innate immunity, and thus risk of infection in a neonate, may be negatively impacted by qualitative phagocyte defects. The term phagocyte stems from the Greek “phagein” meaning “to eat or devour” and “cyte” meaning “cell” and refers to hematopoietic derived cells, namely monocytes, macrophages and neutrophils capable of engulfing and digesting microorganisms, foreign particles, and cellular debris. Neutrophils are also classified as granulocytes, given the characteristic presence of granules in their cytoplasm that play a key role in neutrophil function.
Term and preterm infants have age-appropriate inherent limitations in the functional capabilities of their neutrophils but may also present with additional pathologic phagocytic disorders. It is imperative that clinicians who care for neonates understand the normal immunological dysfunction of the newborn neutrophil and are able to recognize and care for neonates suspected of having a functional phagocyte disorder.
Neutrophil Development in the Neonate
Leukocytes within the mononuclear-phagocyte system, including neutrophils, monocytes, macrophages, eosinophils, and basophils, are derived from a common progenitor cell located within the bone marrow, the colony-forming unit granulocyte-monocyte (CFU-GM). The proliferation and differentiation of these early progenitor cells depends on numerous cytokines with granulocyte-macrophage colony-stimulating factor (GM-CSF) and granulocyte colony-stimulating factor (G-CSF) being the most prominent. Neutrophil development proceeds from the most immature cells capable of mitotic division, i.e., myeloblast to promyelocyte to myelocyte, to immature cells undergoing maturation, i.e., metamyelocyte to band to mature neutrophils. It is these immature neutrophil progenitors that create a reserve pool within the bone marrow capable of cellular division and neutrophil mobilization at times of need [1, 2]. Term neonates have a vastly reduced proliferative pool with estimates at about 10% of adult values. This diminished pool results in a depressed ability to mount an appropriate response to infectious organisms, with newborns often exhausting their neutrophil reserves during active infections and having an elevated risk of mortality and morbidity secondary to sepsis. It is not until approximately 4 weeks of age that neutrophil pool quantities reach those of adults. Small for gestational age, preterm and extremely low birth rate neonates have further diminished proliferative pools and additionally may manifest neutropenia regardless of infection status, secondary to reduced neutrophil production. Reduced phagocytic cell production may be a result of decreased G-CSF and GM-CSF levels in these infants [2–4].
Granulopoiesis, the formation of granules within a cell, is an imperative developmental step during neutrophil maturation, with additional creation of secretory vesicles within the mature neutrophil to assist with cellular migration and adherence. Azurophilic, specific, and gelatinase granules contribute substantially to the extracellular/intracellular antimicrobial and migratory/extravasation properties of neutrophils. Certain neonatal granule protein levels, including lactoferrin and bactericidal permeability-increasing proteins, do appear to be reduced compared to adults with preterm infants also showing decreased degranulation capabilities [2, 3, 5]
Neutrophil Function in the Neonate
See Table 17.1 for a summary of neonatal neutrophil function characteristics and defects.
Table 17.1 Neonatal neutrophil function characteristics
Neutrophil function | Neonatal defect |
---|---|
Migration and adherence | Neonatal neutrophils, particularly preterm infants, have reduced L-selectin expression, L-selectin shedding and beta2-integrin protein upregulation |
Immature vascular endothelium with suboptimal P-selectin | |
Diapedesis and basement membrane passage | All newborns exhibit increased neutrophil rigidity secondary to altered F-actin levels and abnormal membrane fluidity |
Reduced elastase degranulation capabilities in preterm infants affects passage through the endothelial basement membrane | |
Chemotaxis | Reduced expression and function of chemotactic sensory receptors in newborns |
Suboptimal intracellular free calcium levels following activation | |
Preterm infants may not show significant improvement in chemotaxis until 2 months of age | |
Sepsis, indomethacin, and magnesium sulfate may negatively affect chemotactic abilities | |
Phagocytosis | Impaired ability to upregulate CR3 in both preterm and term infants |
Preterm neonates show significantly less expression of Fc gamma receptors | |
Preterm neonates have greatly reduced phagocytosis, but nearly normal phagocytic abilities are restored if focus of phagocytosis is opsonized appropriately | |
Respiratory burst | Ill term and preterm neonates exhibit suppressed respiratory burst |
Healthy preterm newborns show decreased capacity to upregulate oxidative burst following bacterial stimulation | |
Respiratory burst deficiencies appear to persist throughout times of infection and until 2 months of age | |
Neutrophil extracellular traps (NETs) | NETs creation impaired in both term and preterm infants |
Migration and Adherence
Once released from the bone marrow storage pool, neutrophils are capable of migrating from the circulatory system into extravascular sites of inflammation. This process involves multiple steps, all of which show altered ability within the neonate. Following entry into the bloodstream, adherence to the vascular endothelial cells with subsequent leukocyte rolling and adherence is facilitated by cellular membrane molecules. As neutrophils transiently interact and roll along vascular cell surfaces they adhere to sites of inflammation through an interaction between L-selectin on neutrophils and P- and E-selectins on endothelial cells [1]. A firm attachment to the vascular surface is formed by shedding of L-selectin and increased expression of beta2-integrins on the neutrophil with ensuing binding of the beta2-integrins to intercellular endothelial adhesions molecules 1 and 2 (ICAM-1 and ICAM-2) on endothelial cells. Neonatal neutrophils, especially those found in preterm infants, appear to have decreased rolling and adhesion capabilities due to reduced L-selectin expression, L-selectin shedding, and beta2-integrin protein upregulation. An immature vascular endothelium with suboptimal P-selectin may also contribute to poor neutrophil rolling and adhesion [2, 3, 6].
Diapedesis and Basement Membrane Passage
Diapedesis, in which neutrophils migrate between endothelial cells and into the extravascular space, requires a cytoskeleton capable of deformability in addition to the beta2-integrin and ICAM interactions noted above. Furthermore, successful movement through the endothelial basement membrane is facilitated by neutrophil release of digestive enzymes, including elastase and collagenase [1, 2]. Neutrophils from newborns exhibit increased rigidity likely secondary to altered F-actin levels and abnormal membrane fluidity with resultant inability to modify cellular shape upon stimulation. Reduced elastase degranulation capabilities in preterm infants likely also affects passage through the endothelial basement membrane [2–4, 6].
Chemotaxis
The movement of neutrophils within the extravascular space across a gradient of chemotactic factors is termed chemotaxis. This cellular propulsion requires sensory receptors for various chemotactic peptide molecules. Impaired kinesis of newborn neutrophils results from not only reduced expression but decreased function of these receptors. Intracellular calcium plays an important role in chemotaxis following receptor stimulation and neutrophils within neonates appear to have suboptimal intracellular free calcium levels following activation [1, 2]. Term infants reach adult chemotactic abilities by approximately 2 weeks of age but preterm infants may not show significant improvement in chemotaxis until 2 months of age [6]. Sepsis, indomethacin administration, and intrapartum exposure to magnesium sulfate may further negatively affect chemotactic abilities [3, 7].
Phagocytosis
Upon entry into areas of inflammation neutrophils may encounter pathogens necessitating phagocytosis to aid in microbial clearance. Successful phagocytosis by neutrophils is aided by complement receptors, particularly the CR3 receptor, and Fc gamma receptors, which bind immunoglobulins as both complement and immunoglobulin are key contributors to opsonization. The ability to upregulate CR3 is decreased in both preterm and term infants. The expression of Fc gamma receptors in term infants appears to be comparable to adults but preterm neonates show significantly less expression of these receptors [6]. Interestingly, measurement of the phagocytic ability of neutrophils from term newborns was similar to adult controls but preterm neonates manifested greatly reduced phagocytosis when whole blood assays were used. The phagocytic defect observed in preterm infants can be improved by exposing the neutrophils to serum from adults or therapeutic immunoglobulin. Thus it appears that preterm and term neonates can show nearly normal phagocytic abilities if the focus of phagocytosis is opsonized appropriately [3].
Respiratory Burst
Following ingestion by phagocytosis, microorganisms are internalized into phagosomes, which then fuse with lysosomes and granules that contain proteolytic enzymes. These enzymes are next activated in the phagolysosome and digest the microorganism via generation of reactive oxygen species (using NADPH oxidase) and nitric oxide (using nitric oxide synthetase). Myeloperoxidase may additionally use the reactive oxygen species to create hypochlorous acid, which further assists in destruction in pathogens [1,3]. Under non-stressed conditions neutrophils from healthy newborns appear to show an intense respiratory burst response with increased reactive oxygen species generation. This elevated response is not sustained though at times of stress, including pulmonary or infectious insult, with neutrophils from ill neonates exhibiting suppressed respiratory burst. Healthy preterm newborns additionally show a reduced capacity to upregulate oxidative burst following bacterial stimulation. These neonatal respiratory burst deficiencies appear to persist throughout times of infection but will often normalize by 2 months of age in healthy infants [2–4].
Neutrophil Extracellular Traps (NETs)
Neutrophils also participate in a non-phagocytosis method of killing through the creation of neutrophil extracellular traps (NETs). NETs consist of DNA fragments and granule proteins extruded from neutrophils that form an extracellular scaffold of fibers with a high concentration of antimicrobial components with the ability to capture and destroy microbes. NET formation results from a cellular process that is separate from necrosis or apoptosis. The creation of NETs by both term and preterm infants is impaired and appears to further potentially increase their risk of infections [3, 4, 8].
Therapies to Overcome Neonatal Neutrophil Functional Deficiencies
The administration of G-CSF and GM-CSF can increase mobilization of neutrophils from the bone marrow, increase beta2-integrins expression on neutrophils (although they reduce L-selectin levels), and improve the phagocytic and respiratory burst properties of neutrophils [4, 7]. Studies show conflicting results on whether treatment with colony-stimulating factor reduces mortality in neonates with confirmed or presumed sepsis, although absolute neutrophil counts do increase in these patients following the administration of G-CSF [9–11]. Further large randomized studies are necessary to clarify any potential benefit of colony-stimulating factors in ill neonates. It has been found, however, that early postnatal prophylactic GM-CSF in extremely preterm infants (below 31 weeks’ gestation) improves neutropenia but does not decrease rates of sepsis or improve survival [12].
It has been theorized that treatment with intravenous immunoglobulin may improve opsonic and phagocytic activity in septic neonates and potentially lead to improved survival rates but trials exploring this have had diverging results with no definitive conclusion being reached as to whether administration of immune globulin is beneficial in neonates with sepsis or not [4, 13–16]. Studies do show that there is not sufficient benefit to premature infants to routinely recommend prophylactic treatment with immune globulins [4, 16–18].
Functional Phagocyte Disorders in the Neonate
Table 17.2 summarizes disorders of the neutrophil, clinical manifestations, testing and treatments. Further details can be found in the sections that follow.
Table 17.2 Congenital disorders of the neutrophil
Disorder | Gene mutation | Inheritance pattern | Clinical manifestations | Specific diagnostic testing | Treatment |
---|---|---|---|---|---|
CARD9 deficiency | CARD9 | AR | Invasive fungal infections, meningoencephalitis with Candida, chronic mucocutaneous candidiasis, deep and disseminated dermatophytosis | Skin biopsy with granulomatous dermatitis, extensive fungal evaluation | Anti-fungal prophylaxis, GM-CSF, consider HSCT |
Chédiak–Higashi syndrome | LYST | AR | Oculocutaneous albinism, progressive neurologic dysfunction, giant granules within granulocytes, recurrent infections, bleeding diathesis, risk of hemophagocytic lymphohistiocytosis (HLH) | Platelet aggregation study, neuroimaging, EMG/EEG, peripheral smear evaluation, light microscopy of hair shafts, HLH evaluation studies, genetic testing | Antimicrobial prophylaxis, G-CSF, +/− interferon gamma, HLH targeted therapies, HSCT |
Chronic granulomatous disease |
|
| Recurrent bacterial infections particularly with catalase-positive organisms, colitis, granuloma formation, risk of fungal disease and mulch pneumonitis | Neutrophil functional testing (DHR and NBT), ESR/CRP trending, imaging to rule out infections with inflammatory marker rises, genetic testing | Prophylaxis with TMP-SMX and itraconazole, +/− interferon-gamma, aggressive infection management with broad spectrum antimicrobials, glucocorticoids for liver abscess/mulch pneumonitis/Nocardia pneumonia, immunosuppression for severe inflammation, bone marrow transplantation |
Glycogen storage disease type 1b | SLC37A4 or G6PC | AR | Hypoglycemia, seizures, lactic acidosis, hyperuricemia and hyperlipedimia. Neutropenia with defective chemotaxis, perioral and perianal infections, protracted diarrhea and colitis | Biallelic pathogenic variants in G6PC or SLC37A4 genes on molecular genetic testing or hepatic enzyme activity of glucose 6 phosphatase | Metabolic support by expert metabolic team to provide nutrition and particularly to control hypoglycemia, hyperuricemia and renal and hepatic complications. G-CSF therapy for neutropenia and recurrent infections |
Hyperimmunoglobulin E syndromes (STAT3 LOF) | STAT3 | AD | Recurrent “cold” skin abscesses (esp. S. aureus), eczema, retained primary teeth, coarse facial features, vascular anomalies, elevated IgE, eosinophilia, fractures, allergies | Serum IgE and eosinophils, vaccine response studies, dental radiology imaging, Th17 cell count, usage of diagnostic scoring system, annual PFTs/CXRs, genetic testing | Aggressive eczema management, TMP-SMX prophylaxis, +/− interferon-gamma |
IRAK4 deficiency | IRAK4 | AR | Lack of fever, unexpectedly low C-reactive protein (CRP) in setting of infection, recurrent invasive bacterial infections, inadequate polysaccharide vaccine response | Vaccine response studies, absence of toll-like receptor induced cytokine production, genetic testing | Antimicrobial prophylaxis, intravenous immunoglobulin (IVIg), antipneumococcal vaccination |
MyD88 deficiency | MYD88 | AR | Recurrent infections, especially pneumococcal | Vaccine response studies, absence of toll-like receptor induced cytokine production, genetic testing | Quality skin care, antimicrobial prophylaxis, IVIg, antipneumococcal vaccination |
Leukocyte adhesion deficiency (LAD) | ITGB2 | AR | Leukocytosis, delayed umbilical cord separation, impaired wound healing, severe periodontitis, gingivitis, ulcerative skin lesions, colitis, HPV infections | CD18 and CD11 evaluation via flow cytometry, genetic testing | Excellent oral hygiene, antimicrobial prophylaxis, GM-CSF not useful, HSCT with severe disease |
SLC35C1 | AR | Leukocytosis, recurrent infections, intellectual disability, depressed nasal bridge, Bombay blood phenotype | Analysis of blood phenotype, absence of CD15a on flow cytometry, genetic testing | Antimicrobial prophylaxis, excellent oral hygiene, fucose supplementation | |
FERMT3 | AR | Leukocytosis, recurrent infections, bleeding, osteoporosis-like bone abnormalities |
| Antimicrobial prophylaxis, platelet transfusions with bleeding, HSCT | |
Mendelian susceptibility to mycobacterial diseases (IRF8 deficiency) | IRF8 | AR | Severe monocytopenia and lack of dendritic cells, disseminated BCG infection, intellectual delay | Mendelian susceptibility to mycobacterial diseases flow cytometry | Mycobacterial prophylaxis, consideration for HSCT in severe disease |
Myeloperoxidase deficiency | MPO | Mixed | Often asymptomatic, Candida infections in the setting of diabetes | Histochemical staining for MPO | Antimicrobial prophylaxis in those with recurrent infections |
NF-κB essential modifier (NEMO) deficiency | IKBKG | XL | Immunodeficiency, mycobacterial susceptibility, ectodermal dysplasia, inflammatory/autoimmunity | Immunoglobulin levels, vaccination responses, genetic testing | IVIg, azithromycin, and TMP-SMX prophylaxis, HSCT |
Neutrophil-specific granule deficiency | CEBPE SMARCD2 | AR | Increased pyogenic infections, absence of specific granules, frequent bi-lobed neutrophils, risk of MDS/AML | Light and electron microscopy evaluation of neutrophils, biochemical measurements of granule proteins | Antimicrobial prophylaxis, aggressive management of infections, GM-CSF with severe infections, HSCT in those with risk of malignant transformation |
Shwachman–Diamond syndrome |
|
| Pancreatic exocrine insufficiency, steatorrhea, skeletal abnormalities, short stature, elevated transaminases, bone marrow failure, risk for MDS/AML | Bone marrow evaluation, ultrasound of pancreas, serum trypsinogen (<3yo) or isoamylase (>3yo), genetic screening | G-CSF, annual bone marrow evaluations with FISH for MDS genetic alterations, pancreatic enzyme replacement, HSCT in those with severe disease |
Wiskott–Aldrich syndrome | WAS | XL | Bleeding, immunodeficiency, eczema, malignancy | WAS protein analysis via flow cytometry, mean platelet value, genetic testing | Prophylactic antimicrobials, irradiated platelets, IVIG, rituximab in those with autoimmune cytopenias, HSCT |
CARD9 Deficiency
CARD9 deficiency is an autosomal recessive disorder due to mutations in caspase recruitment domain-containing protein 9 (CARD9), an intracellular myeloid signaling molecule downstream of C-type lectin and Toll-like receptors [19]. Mutations in CARD9 render the neutrophil defective against Candida and other serious fungal infections. Patients with CARD9 deficiency not unexpectedly present with a heightened susceptibility to fungal diseases including central nervous system and gastrointestinal tract infections with Candida, invasive Exophiala infections, and persistent mucocutaneous candidiasis. The invasiveness of the fungal disease is likely secondary to the killing defect of neutrophils and separates CARD9 deficiency from other disorders with chronic mucocutaneous candidiasis.
The deficit in fungal immunity is a result of the breakdown of the normal host defense. Normal host defense against Candida relies on opsonins, especially complement, and importantly the interaction of β-glucan and mannan residues on the surface of Candida with the C-type lectins expressed on the plasma membrane of myeloid cells to trigger an inflammatory response [20]. Activation of the C-type lectin or Toll-like receptors via recognition of fungal cell wall components results in phosphorylation of CARD9 and formation of a trimeric complex with B-cell lymphoma 10 (BCL10) and mucosa-associated lymphoid tissue lymphoma translocation gene 1 (MALT1). Formation of this trimeric CARD9-BCL10-MALT1 complex subsequently activates NF-ĸB and generates a pro-inflammatory response with IL-6 and IL-1β production that enhances antifungal, particularly anti-Candida, neutrophil function. CARD9 is also induces T-helper cells to produce interleukin-17 (IL-17), which plays a major role in the clearance of Candida [20]. Treatment of CARD9 deficiency relies on aggressive anti-fungal therapy in combination of with G-CSF and/or GM-CSF.
Chédiak–Higashi Syndrome
Chédiak–Higashi syndrome (CHS) is an autosomal recessive disorder that manifests with oculocutaneous albinism, neurologic dysfunction, abnormal coagulation, an elevated risk of hemophagocytic lymphohistiocytosis (HLH), and frequent infections with bacterial pathogens. A defect in the CHS1/LYST gene leads to abnormalities in lysosomal/vesicular transport and granulopoiesis. Giant azurophilic granules develop within patient neutrophils and other granulocytes and their appearance can assist in the diagnosis of patients [1]. Additionally, CHS neutrophils are deficient in granule proteases, suffer from delayed/incomplete degranulation and display faulty chemotaxis. [1, 21]. These functional neutrophil defects lead to recurrent and potentially serious cutaneous, respiratory tract, and mucous membrane bacterial infections. The lysosome and granule dysfunctions of the disorder lead to the clinical manifestations of albinism and coagulopathy as a result of improper transportation of melanosomes within melanocytes and reduction in platelet-dense bodies creating a platelet storage pool deficiency. Further hematological manifestations of the syndrome include neutropenia and a risk of development of hemophagocytic lymphohistiocytosis as the patient ages and enters the “accelerated phase” of the disorder. Patients are additionally at risk of developing progressive neurologic abnormalities, including ataxia, peripheral neuropathy, seizures, motor/sensory deficits, and cognitive decline [1, 22].
Diagnosis should be considered in those caring for neonates exhibiting albinism and frequent pyogenic infections. Examination of the infant’s blood smear may reveal absolute neutropenia and the presence of the classic large granules within all granulocytes, including neutrophils. Examination of fetal hair may reveal giant melanin granules. Definitive diagnosis is made via mutational testing for the CHS1/LYST gene. In those with a family history of the syndrome, prenatal diagnosis is additionally possible via analysis of chorionic villus or amniotic cells [1, 22].
Therapy is aimed at prophylactic administration of antibiotics, often trimethoprim-sulfamethoxazole, and aggressive treatment with antimicrobial therapy in those with proven or suspected infection. G-CSF administration may improve neutropenia. Patients often are referred to undergo hematopoietic stem cell transplantation (HSCT) prior to the development of hemophagocytic lymphohistiocytosis or devastating infections, but HSCT does not correct the neurologic deficits [1]. Treatment with ascorbic acid at high doses may improve the function of neutrophils in those with CHS and is reasonable to trial in patients [1, 23–25]
Chronic Granulomatous Disease
Chronic granulomatous disease (CGD) occurs with an estimated frequency of 1:200,000 live births and is due to mutations that disrupt the oxidative burst capacity of the nicotinamide adenine dinucleotide phosphate (NAPDH) oxidative complex in phagocytes [26]. Mutations in any of the genes (CYBB, CYBA, NFC1, NFC2, NFC4) that encode the five subunits (gp91phox, p22phox, p47phox, p67phox, p40phox, respectively) of NAPDH oxidase result in CGD. Defective oxidative burst results in the inability of neutrophils, monocytes and macrophages to kill many organisms, particularly catalase-positive bacteria such as Staphylococcus aureus, Burkholderia cepacia, Serratia marcescens, Nocardia, Samonella, and fungi such as Aspergillus.
Patients with CGD frequently present in infancy with the majority of patients presenting by childhood with severe or recurrent catalase-positive bacterial or fungal infections affecting the skin, lungs, liver, and lymph nodes. Fungal infections are the most common cause of death and carry a higher mortality risk than bacterial infections, although infections in CGD patients may be clinically underwhelming even with extensive disease [27]. Besides infections, patients with CGD are prone to inflammatory complications including granuloma formation and inflammatory bowel disease [28]. The dihydrorhodamine 123 (DHR) oxidation test can functionally assess the oxidative burst in clinically concerning patients. Gene sequencing can further confirm the disorder. Of note, patients with the X-linked form of CGD, due to mutations in CYBB, generally present with more severe disease as do patients with completely absent or extremely low residual superoxide production [29].
Treatment of CGD relies on aggressive treatment of active infections as well as prophylactic antimicrobials, e.g., trimethoprim-sulfamethoxazole, and antifungals, e.g., itraconazole [30]. Many patients also benefit from the use of interferon-gamma, although treatment with interferon-gamma is not universal [31]. Patients can be cured with hematopoietic stem cell transplant but the decision to proceed to transplant is based on the degree of disease severity and the quality of the donor match [32]. A limited number of patients have also been treated with gene therapy.
Glycogen Storage Disease Type 1b
Glycogen storage disease type 1b, also known as glucose-6-phosphatase deficiency or von Gierke disease, is an autosomal recessive condition most prominently associated with hypoglycemia and renal/hepatic abnormalities, but the disorder may also manifest neutropenia with dysfunctional neutrophil chemotaxis and respiratory burst capabilities [33, 34]. The majority of patients present with neutropenia prior to 1 year of age, although the neutropenia may be intermittent. The clinical impact of the neutropenia and neutrophil dysfunction is often clinically apparent in patients with the presence of perioral and perirectal infections as well as protracted diarrhea/colitis frequently reported [33]. A definitive diagnosis is made via genetic testing and G-CSF administration may reduce the frequency and severity of infections.
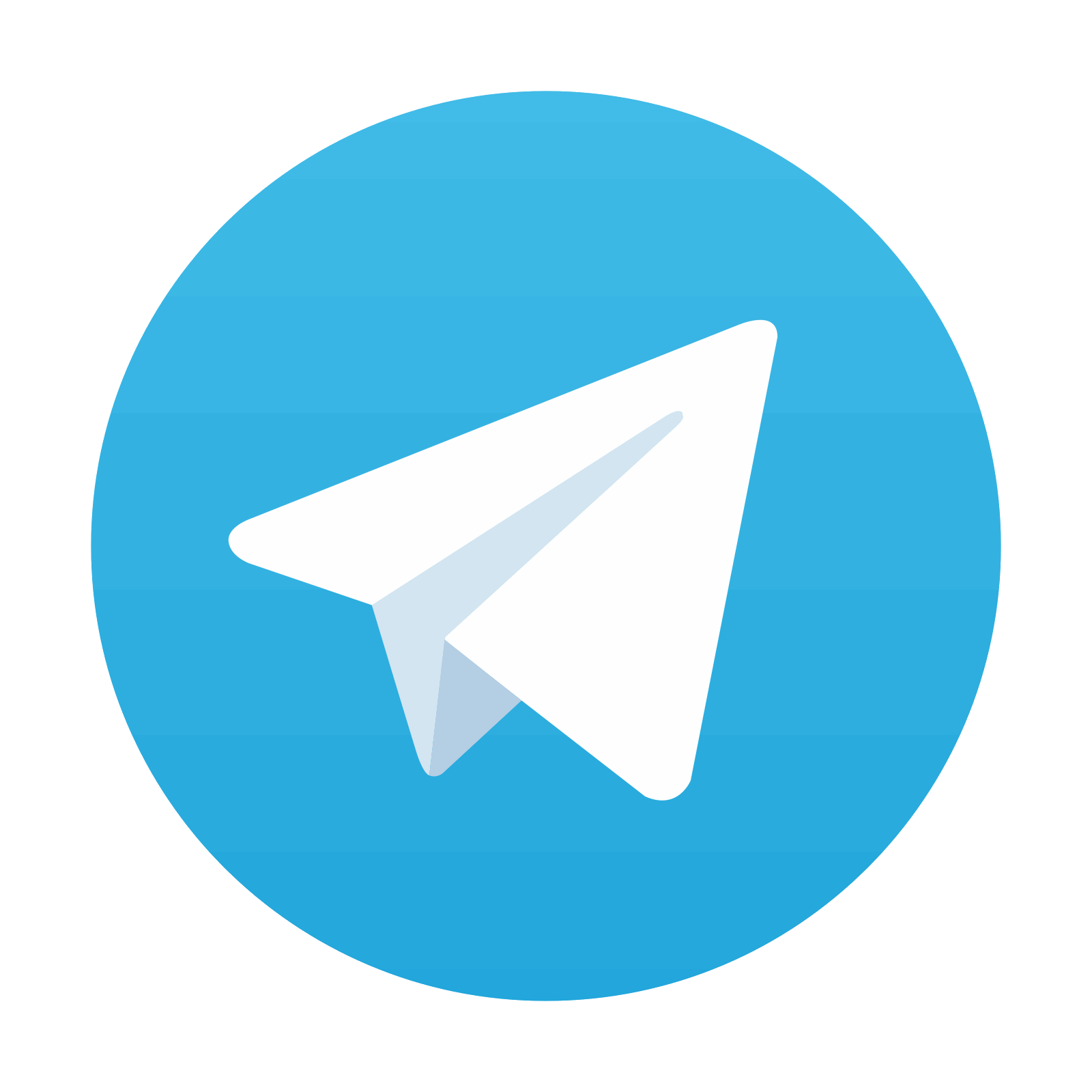
Stay updated, free articles. Join our Telegram channel

Full access? Get Clinical Tree
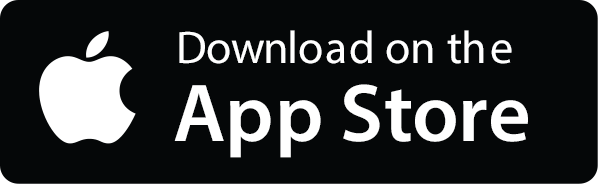
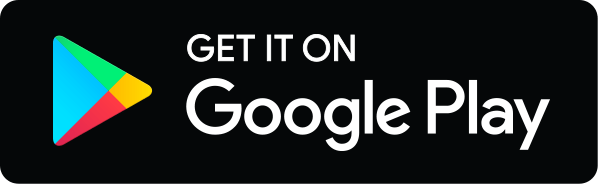
