Carcinomas of Follicular Epithelium: Epidemiology and Pathogenesis
Arthur B. Schneider
Alina V. Brenner
The thyroid gland is an uncommon site of cancer, accounting for only 1.4% and 4.6% new cases of carcinomas among men and women, respectively, in 2010 in the United States (1). Because of its favorable prognosis, thyroid carcinoma causes an even lower percentage of cancer deaths (1), 0.24% and 0.36% for men and women, respectively. Since 1980, however, the incidence of thyroid carcinoma in the United States has been increasing rapidly (2). As shown in Figure 50A.1, the increase has been particularly steep since 1998 with 6.5% increase per year among women (p <0.05) and 5.3% per year (p <0.05) among men, making thyroid carcinoma the most rapidly increasing malignancy in both women and men in the general population (2).
In contrast to incidence, the changes in mortality rates were much smaller. From the 1980s to 2007, the mortality rate for thyroid carcinoma increased by 1.1% per year (p <0.05) among men, whereas there was no change (0.3% per year) in women. Improved treatment, a decline in anaplastic thyroid carcinoma (3), and the fact that the increase is primarily in early stage papillary carcinomas likely explain why, despite the increasing incidence, there has been little or no change in mortality.
Thyroid cancer has an unusual age distribution, somewhat between the pattern for adult and childhood carcinomas (Fig. 50A.2). Although the median age at diagnosis for carcinomas at all sites combined is 66 years, it is only 49 years for thyroid cancer (2). In the United States, thyroid carcinoma occurs about three times more frequently in women than men; the female-to-male ratio is highest after puberty and decreases monotonically with age to 1.2 around 70 years (Fig. 50A.3). While the female-to-male ratio is relatively constant over different racial and ethnic groups, thyroid carcinoma incidence rates vary substantially (2). Age-adjusted rates are about two-fold higher in white men and women compared with black men and women, whereas white Hispanics have nearly the same rates as non-Hispanic whites. The highest rates among US residents are found in Filipino men and women, about twice those of whites. The most common histologic type of thyroid cancer is, by far, the papillary type (4,5,6). Its proportion has increased over time to 70% or more in many countries including the United States. This resulted, in part, from the 1988 revision of the WHO histopathologic classification of thyroid carcinomas resulting in some follicular carcinomas being reclassified as a follicular variant of papillary carcinoma (7) and from other factors including improved ascertainment. The relative frequency of follicular carcinoma ranges from about 10% to 40% depending on the iodine status of the region. Anaplastic and medullary carcinomas usually account for the remaining 5% of thyroid carcinomas.
The increasing incidence of thyroid carcinoma seen over the past three decades in the United States (8,9,10,11,12,13,14) occurred in other developed countries including Canada (15,16), Australia (17,18), Israel (19), and many European countries (20,21,22,23,24). The largest international study to date that compared age-standardized incidence rates of thyroid cancer over the 30-year period 1973 to 2002 in 19 populations from the Americas, Asia, Europe, and Oceania using incidence data from Cancer Incidence in Five Continents, concluded that from 1973–1977 to 1998–2002 the average increase in incidence of thyroid carcinoma was 66.7% among females and 48.0% among males for most of the populations (25). Analyses by histologic type and tumor size for those populations with available data found that the sharpest increase occurred in incidence of papillary thyroid cancer, particularly of papillary microcarcinoma (<1 cm), similar to what was found in US studies. There is a striking difference between more developed and less developed countries with respect to thyroid carcinoma rates. While the age-adjusted mortality rates are very similar, the age-adjusted incidence rates are three-fold higher in the more developed countries (26).
To understand this apparently uniform increase in thyroid carcinoma incidence, several age–period–cohort analyses have been performed. Calendar period effects are typically related to changes in screening practices, diagnostic techniques, or classification definitions, while birth cohort effects typically reflect the effect of risk factors on the incidence rates within birth cohort. In the United States the most convincing age–period–cohort studies used the Surveillance, Epidemiology, and End Results (SEER) data, a unique source of population-based information on cancer incidence, prevalence, and survival for selected geographic areas covering 28% of the US population (2,8,9,10). The studies found that the rise in thyroid carcinoma has been largely caused by an increase in incidence of papillary thyroid carcinoma; smaller, but significant increases in incidence of follicular and medullary thyroid carcinomas were reported as well. For papillary carcinoma, increases in incidence were more rapid among females than males, among whites and blacks than among other racial groups, and apparent in all age groups except the very young. Within each sex and racial/ethnic group, the largest increases were for smaller (<1 cm) and localized tumors which seem to be consistent with increased detection rather than a true increase in incidence of thyroid carcinoma. However, because rates for larger and more advanced tumors also increased, some have argued that increased ascertainment cannot fully explain these trends. In addition, several groups found that at every age over 20 years, successive birth cohorts in USA had higher incidence rates for papillary thyroid cancer than earlier ones, suggesting that these trends may reflect birth cohort–related changes in environmental exposures (8,9). Similar patterns were observed in an analysis of age–period–cohort effects for thyroid carcinoma of all histologic types in Canada (16).
In summary, it is very likely that introduction of ultrasound for thyroid examination in early 1980s, fine-needle aspiration biopsy in the 1990s, and recent widespread use of new imaging techniques such as computer tomography (CT) and magnetic resonance imaging (MRI) contributed to the increasing
incidence of thyroid carcinoma in USA and many other countries. This is supported by the fact that the sharpest increase involved small tumors. However, increases in large and more advanced tumors coupled with increase in incidence of thyroid cancer in the successive birth cohorts suggest that environmental exposures early in life may contribute to the increasing incidence of thyroid cancer.
incidence of thyroid carcinoma in USA and many other countries. This is supported by the fact that the sharpest increase involved small tumors. However, increases in large and more advanced tumors coupled with increase in incidence of thyroid cancer in the successive birth cohorts suggest that environmental exposures early in life may contribute to the increasing incidence of thyroid cancer.
Radiation exposure during childhood is the clearest pathogenetic factor associated with thyroid carcinoma (27). Thus, it has been suggested that some increase in thyroid cancer incidence for US and Canadian cohorts born before the 1950s is likely due to the use of radiation therapy for benign childhood conditions, radioactive fallout from nuclear tests conducted in Nevada in the 1940s to 1960s, or increased use of diagnostic irradiation. Environmental and other factors (e.g., diet, reproductive, genetic, anthropomorphic) have been implicated in the etiology of thyroid carcinoma. The evidence that relates these factors and radiation exposure to thyroid carcinoma is reviewed in this chapter. Many of these factors are thought to operate through the action of thyrotropin (TSH). There is considerable support from animal experiments that prolonged TSH stimulation can contribute to thyroid carcinogenesis, but in humans the evidence is less clear (28). An indication that prolonged TSH plays a role in humans comes from the recent observations that patients with malignant thyroid nodules tend to have higher TSH levels compared to patients with benign nodules (29,30,31).
Radiation
It is generally accepted that radiation causes thyroid carcinoma primarily by direct effects on DNA. The site of this initial DNA damage and the path leading to the eventual carcinoma involve multiple, as yet incompletely understood, steps (32,33). The proximity in the nucleus of chromosomes with genes that undergo oncogenic rearrangements plays an important role (33). While radiation can initiate thyroid carcinogenesis, additional factors and steps are required before clinically evident carcinomas occur, making it possible that interventions, such as giving thyroid hormone to lower TSH, will be found that prevent the progression to clinically important thyroid tumors.
National and international organizations issue comprehensive reports on the health effects of exposure to ionizing radiation (34,35,36,37). Wide recognition of the risk for thyroid carcinoma has resulted in the near disappearance of radiation treatments for benign conditions. Nevertheless, as a result of the increasing use of diagnostic imaging procedures, the thyroid continues to be exposed to radiation (38,39).
External Radiation and Thyroid Carcinoma
The relationship between radiation and thyroid carcinoma was first recognized by Duffy and Fitzgerald in 1950 (40). They found that an unusually large fraction of their childhood thyroid carcinoma patients had a history of radiation therapy for benign conditions of the head and the neck. This relationship was subsequently confirmed by many case-control and cohort studies (35,36).
Several difficulties arise in studying the relationship between radiation exposure and thyroid carcinoma. Since thyroid carcinoma remains an uncommon disease, even with its increasing incidence, few studies have sufficient statistical power to adequately quantify risk; the very good survival rate of thyroid carcinoma patients requires that incidence rather than mortality be assessed; because radiation exposure frequently occurs at a young age, people often are unaware of, or uncertain about, their exposure; and, as discussed above, the diagnosis of thyroid tumors is highly dependent on the extent of the procedures used to look for them (diagnostic bias). In the case-control studies, cases of thyroid carcinoma were identified by their entry into a tumor registry or by their admission to a hospital. The control subjects were comparable subjects without thyroid carcinoma. Information on risk factors, such as radiation exposure, was obtained retrospectively and the distribution in the two groups compared. In case-control studies, cases may report exposure to risk factors more completely than controls (recall bias). In the cohort studies exposure to radiation generally was documented and the characteristics and amount of exposure were known. The frequency of thyroid carcinoma in the radiation-exposed group was compared with a group of similar subjects who had little or no exposure. Therefore, in cohort studies, recall bias was minimized, but diagnostic bias could have been important. The evidence concerning radiation exposure and thyroid carcinoma is especially strong because multiple studies conducted in various locations using different methodologies report similar findings (41,42,43,44,45,46,47,48,49,50,51,52,53,54,55,56,57,58,59).
Ron et al. (27) conducted the most comprehensive analysis of external radiation exposure and thyroid carcinoma, combining the data from seven studies that had individual thyroid dose estimates. Their analysis of childhood exposure, which included nearly 500 patients with thyroid carcinomas, demonstrated a strong positive association between radiation dose and thyroid carcinoma. On the basis of an excess relative risk (ERR) model (i.e., risk increases multiplicatively with dose), a linear dose–response relationship fit the data well. A consistent and strong relationship between radiation exposures to doses as low as 0.1 Gy (10 rad) and thyroid carcinoma was found. A more recent report from one of the studies included in the pooled analysis suggests that the effects extend down even
further, to about 0.05 Gy (55). At very low doses, because of the large numbers needed to prove an effect, the results are equivocal, but a linear no-threshold dose–response model describes the data well and is used for purposes of radiation safety analyses. At high doses, typical of those associated with the treatments of malignancies, there is a flattening or downturn in the dose–response relationship, but a considerable risk remains (54,60,61).
further, to about 0.05 Gy (55). At very low doses, because of the large numbers needed to prove an effect, the results are equivocal, but a linear no-threshold dose–response model describes the data well and is used for purposes of radiation safety analyses. At high doses, typical of those associated with the treatments of malignancies, there is a flattening or downturn in the dose–response relationship, but a considerable risk remains (54,60,61).
There is a very strong inverse relationship between age at exposure and radiation-related thyroid carcinoma risk. In the pooled analysis, there was little evidence for a radiation effect among persons exposed after age 15 years (Fig. 50A.4). Subsequent analyses of data from atomic bomb survivors extend this finding, showing that the risk for exposure after age 10 years, although reduced, remained significantly elevated (58). Even in this age range there was a strong (but not significant) decrease in ERR with an increasing age at exposure. The fitted ERR of thyroid carcinoma for exposure at age 30 years was about twice as large as that for exposure at age 50 years and for exposure at age 10 years the ERR was twice that for exposure at age 30 years (58). A separate re-analysis of data for female atomic bomb survivors who were 20 years or older at the time of bombings found positive association between radiation exposure and thyroid cancer (62). Similar findings were made in another study where the analysis was limited to microcarcinomas found in autopsy examinations of people who were predominantly adults when exposed as a result of the atomic bomb (63). Women tend to be more sensitive to the effects of radiation overall, although the difference in radiation-related risk of thyroid carcinoma between men and women was not statistically significant and was not consistent across studies (27). The radiation risk remains elevated several decades after the initial exposure. Between 5 and 30 years after exposure the risk is essentially constant. After 30 years, it appears to decline, but still remains elevated (35).
Internal Radiation and Thyroid Carcinoma
It is now clear from the 1986 Chernobyl accident experience that exposure to radioactive iodines (RAI), predominantly iodine 131 (131I), during childhood is associated with an increased risk for thyroid carcinoma (64,65,66,67). Nevertheless, substantial evidence supports the safety of using 131I in the clinical setting for diagnostic and therapeutic purposes. The following two sections summarize the evidence for both of these exposure settings and discuss the likely explanations for this apparent paradox.
Iodine 131 Releases into the Environment
Even prior to the Chernobyl accident, there was concern about the carcinogenic potential of 131I. Some people living on certain atolls of the Marshall Islands who were exposed to fallout from a nuclear test explosion in 1954 subsequently developed thyroid tumors, including carcinomas (42,68,69,70). However, their radiation exposure came from a combination of 131I, other more rapidly decaying isotopes of iodine, and external gamma radiation.
Shortly after the accident at the Chernobyl nuclear power plant, which released an estimated 41 to 51 MCi of 131I, reports began to appear of thyroid carcinoma occurring in exposed children. Because of the unusually short latency and the intense thyroid screening performed in the area, it was not immediately clear whether these carcinomas were attributable to exposure to 131I from the accident. The data collected during the years since the accident now indicate that the sharp increase in thyroid carcinoma, now estimated to be over 6,000 cases in contaminated areas of Belarus, Russian Federation, and Ukraine, is associated with exposure to 131I and, to a much lesser extent other iodine isotopes, from Chernobyl (64,65,66,67,71,72). This conclusion is drawn from several lines of evidence. In the key studies, an international panel of pathologists has reviewed and confirmed the thyroid carcinoma diagnoses. The incidence of thyroid neoplasms is far higher than the incidence prior to the accident, and the cases have been confined almost exclusively to those who were exposed as children or while in utero. As has been demonstrated in studies of external radiation, the risk for developing thyroid carcinoma in the Chernobyl area has been found to increase with dose. Prospective cohort studies in Ukraine and Belarus, and several case-control studies in Russian Federation and Belarus in the areas affected by the accident with detailed reconstruction of individual thyroid doses have been conducted (73,74,75,76). In the cohort studies the mean 131I thyroid doses were 650 mGy in Ukraine and 560 mGy in Belarus. Participants were examined using standardized ultrasound examination irrespective of dose, prevalent cases of thyroid cancer were detected during the initial screening examination, and dose–response relationships and their associated risk estimates were calculated. Perhaps the most persuasive evidence comes from the subsequent (incident) cases detected during follow-up screenings. The association between thyroid cancer risk and 131I thyroid dose persisted, although the magnitude of the risk had decreased. The risk estimates from these studies, while lower, were compatible to those reported for external radiation (27,65).
There are several distinctive features of the Chernobyl-related cases of thyroid carcinoma, particularly when diagnosed in childhood. The latency was shorter than previously
reported for external radiation-related cases. This may be a result of intense thyroid screening, the promotion of thyroid tumorigenesis by iodine deficiency (77,78), or the large number of exposed individuals, which increases the chance to observe an uncommon disease. Furthermore, many of the cases had a unique histologic pattern of papillary carcinoma with a large solid component (79,80,81), and cases appeared to be rapidly growing and aggressive, with infiltration beyond the capsule and lymph node involvement. Some of these findings are similar to those of thyroid carcinoma in children in general (81). Finally, the pattern of somatic mutations in the carcinomas, involving translocations causing rearrangements of the RET oncogene, appear to be relatively distinctive, and reflective of radiation-induced tumorigenesis (82). (see Chapter 50B). While some clinical correlations have been made, such as between RET/PTC3 and the solid variant of papillary carcinoma, in general it remains unclear how specific RET and other rearrangements are related to clinical behavior.
reported for external radiation-related cases. This may be a result of intense thyroid screening, the promotion of thyroid tumorigenesis by iodine deficiency (77,78), or the large number of exposed individuals, which increases the chance to observe an uncommon disease. Furthermore, many of the cases had a unique histologic pattern of papillary carcinoma with a large solid component (79,80,81), and cases appeared to be rapidly growing and aggressive, with infiltration beyond the capsule and lymph node involvement. Some of these findings are similar to those of thyroid carcinoma in children in general (81). Finally, the pattern of somatic mutations in the carcinomas, involving translocations causing rearrangements of the RET oncogene, appear to be relatively distinctive, and reflective of radiation-induced tumorigenesis (82). (see Chapter 50B). While some clinical correlations have been made, such as between RET/PTC3 and the solid variant of papillary carcinoma, in general it remains unclear how specific RET and other rearrangements are related to clinical behavior.
In addition to the Marshall Islands and Chernobyl, 131I was widely dispersed into the environment in the United States and elsewhere by above-ground nuclear tests (83) and as a by-product of plants preparing isotopes for use in nuclear weapons (84). An epidemiologic study of children exposed to nuclear fallout from weapons testing at the Nevada Test Site found a significant association between all thyroid nodules and dose, but there were too few malignant cases to estimate the risk for thyroid carcinoma separately (47,85). In a detailed evaluation of thyroid doses received by Americans during the testing period, the estimated average collective dose was 2.0 cGy. For people under age 20 years at the time of exposure, the mean dose was about 10 cGy (83). A study of US thyroid carcinoma incidence and mortality rates using place of residence to estimate 131I doses from the bomb tests found no association with cumulative dose, although associations were observed for children exposed under 1 year of age and those in the 1950 to 1959 birth cohort (86). Although it is difficult to determine the number of potential excess thyroid carcinomas caused by exposure to the tests, a National Academy of Sciences (USA) committee reported in 1999 that, if there is an excess, it is probably no more than 11,000 cases and that about 45% of them had already been diagnosed (65).
The Hanford nuclear site in Washington state released 131I into the atmosphere, particularly in its early years, as part of the process of preparing fissionable materials for the US atomic weapons program. Prior to studying the potential consequences of the resulting exposure, methods were devised to estimate (“reconstruct”) individual thyroid doses. Subsequently, study individuals were selected to represent the spectrum of doses, to determine if there were any dose–response relationships for several thyroid diseases, particularly thyroid carcinoma. In the Hanford Thyroid Disease Study, the thyroid glands of 3,441 people who were born in the vicinity of the Hanford nuclear plant between 1940 and 1946 were thoroughly examined, including by ultrasound imaging. Among the 3,193 participants who lived in the Hanford site region during the years of atmospheric emissions, the mean and median thyroid doses were 18.6 and 10.0 cGy, respectively. No evidence of an increased risk for developing benign or malignant thyroid neoplasms associated with childhood exposure to the atmospheric releases of RAI from the Hanford nuclear site was found (87). The reasons for the apparent differences in the findings at Chernobyl and at Hanford are not clear, although differences in the level of exposure, intake of dietary iodine, exposure to short-lived isotopes of iodine, and genetic predisposition may be involved.
Medical Uses of Iodine 131
Iodine 131 Therapy
A great deal of effort has gone into assessing the relationship between 131I used for therapeutic or diagnostic medical purposes and the development of thyroid carcinoma (88,89,90,91,92,93,94,95,96,97,98). These studies generally have been reassuring about the use of 131I, but small increased risks for anaplastic thyroid carcinoma have been reported following 131I therapy for hyperthyroidism (95,96). The US Thyrotoxicosis Therapy Follow-up Study Group reported that among 35,593 hyperthyroid patients treated during 1946 to 1964 and followed through 1990, 63% of whom were treated with 131I, total carcinoma mortality was not associated with 131I use (96). However, the standardized mortality rate for thyroid carcinoma was slightly, but significantly, elevated, resulting in a very small number of excess thyroid carcinomas (96). Most of the deaths occurred soon after 131I treatment, and were associated with toxic multinodular goiter rather than Graves’ disease, suggesting that the underlying thyroid disease may have been involved in the development of thyroid carcinoma, or may have influenced the surveillance or reporting on death certificates (96,99). In a study conducted in Birmingham, England, 634 carcinomas were diagnosed among 7,417 hyperthyroid patients treated with 131I (95,100). Although the overall cancer incidence and mortality rates were lower than expected compared with age, sex, and period-specific national rates, both the thyroid cancer incidence and mortality rate were significantly higher than expected.
In a study of 10,552 hyperthyroid patients treated with 131I in Sweden, there was a nonsignificant 30% increased risk for thyroid carcinoma incidence that was more pronounced among the toxic nodular goiter patients (90). No dose–response relationship was demonstrated. Although the number of excess cases of thyroid cancer was small in these investigations, the increased relative risk suggests that these patients need continued follow-up.
Diagnostic Use of Iodine 131 Therapy
The health effects following diagnostic use of 131I have been studied most thoroughly in Sweden (93,94,98). Among 36,792 predominantly adult patients administered diagnostic 131I and followed through 1998, 24,010 had scans for reasons other than suspicion of a thyroid tumor and did not have a history of exposure to external radiation. The average absorbed thyroid dose was estimated to be 0.94 Gy. The number of observed thyroid carcinomas was very close to the number expected [respectively, 36 vs. 39.5 SIR (standardized incidence ratio) = 0.91; 95% confidence interval (CI) 0.64–1.26]. In contrast, there was an increased risk of thyroid carcinoma among patients whose scan was performed due to suspicion of a thyroid tumor. Among patients with a history of external radiation exposure, the risk was increased about 10-fold. However, there was no evidence that the 131I exposure contributed to the risk, because there was no effect of age or dose of 131I exposure (98). When a sample of approximately 1,005 female patients examined with 131I and 248 nonexposed female patient controls were examined for palpable thyroid nodularity, no excess was found among exposed women compared with nonexposed women. However, among the exposed women there was a
significant relationship between 131I dose and the prevalence of nodules (93).
significant relationship between 131I dose and the prevalence of nodules (93).
It is of special interest to know if diagnostic 131I exposure in children is associated with thyroid carcinoma; however, the available data are too sparse for any conclusions. After a mean of 20 years, examinations were performed on 789 people who had a history of diagnostic 131I procedures (mean thyroid dose of 100 cGy) before age 18 and 1,118 controls who had other thyroid-related tests. Although no radiation effect was observed on the basis of two exposed and three nonexposed cases (relative risk 0.86; 95% CI 0.14–5.13), this study provides little relevant information (97). In this study, only 147 people were 10 years of age or less at the time of 131I administration, and the study was severely limited by the low and differential participation rates for exposed and nonexposed subjects (35% and 41%, respectively), which could result in substantial bias.
In general, studies of adult 131I exposure for therapeutic and diagnostic purposes are reassuring, but some aspects of the studies do not exclude a small effect, apparently related to 131I exposure, on thyroid nodularity, thyroid carcinoma incidence, and thyroid carcinoma mortality. Although a causal relationship is possible, it may be that the observations are related to underlying thyroid condition or an increase in surveillance or diagnostic misclassification.
Comparison of External and Internal Radiation
The differences in the carcinogenic effects of external and internal radiation are probably more apparent than real, although considerable uncertainty remains. The strongest reason to believe that the effects are comparable is a comparison of the findings in the Chernobyl area with those in children treated with external radiation. However, this does not accord completely with the findings with the medical uses of 131I and in people exposed by releases of 131I from the Hanford nuclear facility. One explanatory factor is undoubtedly age at exposure, which has a very strong modifying effect for external radiation (Fig. 50A.4). Since most of the patients included in studies of the medical uses of 131I have been adults, the results cannot be extrapolated to children. Another factor is that compared with the instantaneous dose received from external radiation, the lower dose rate of 131I may allow repair of radiation damage. Finally, when 131I is administered, it results in a wide range of doses to different areas of the thyroid, whereas external radiation exposes the entire thyroid to the same dose, making it difficult to compare the two types of radiation. Exposures to 131I, as occurs in the medical setting, may not be applicable to the more prolonged exposures people generally receive from fallout or living near nuclear production facilities.
Preventing Iodine 131–related Thyroid Carcinoma
Functioning nuclear plants continue to generate iodine isotopes in large quantities that could, in the case of an accident or terrorist attack, pose a threat to the surrounding population. The events at the Fukushima nuclear power plant in Japan in 2011 serve as a reminder of this danger. Potassium iodide (KI), if taken promptly, effectively reduces the dose to the thyroid (101,102,103). Following the Chernobyl accident, KI was widely administered to residents of Poland (104). The success of the program and the rarity of observed side effects support the distribution of KI as an effective means of reducing 131I exposure in the event of a nuclear accident. The World Health Organization and the U.S. Food and Drug Administration have both adopted age-based guidelines for the use of KI in case of an emergency, taking into account the age-dependent sensitivity of the thyroid (105,106). In the United States, for everyone less than 18 years old and for pregnant women KI is advised when the projected radiation dose to the thyroid is ≥50 mGy (Table 50A.1). For ages 18 to 40 years the threshold is 100 mGy and for over 40 years the threshold is 5 Gy. The World Health Organization advocates lower thresholds (106). In some countries the distribution of KI to homes around nuclear facilities has been achieved. In the United States pre-distribution is federally supported for a 10 mile radius around nuclear facilities, but implementation varies from state to state (http://www.huffingtonpost.com/2011/03/31/us-rules-on-radiation-pills-vary-widely_n_843281.html, accessed 4/4/2011).
Table 50A.1 Threshold Thyroid Radioactive Exposures and Recommended Doses of Ki in the Us for Different Risk Groups | |||||||||||||||||||||||||||
---|---|---|---|---|---|---|---|---|---|---|---|---|---|---|---|---|---|---|---|---|---|---|---|---|---|---|---|
|
Evaluation of Irradiated Patients
An essential part of evaluating a person with a history of irradiation is determining the type of radiation, the site or sites treated, the age at treatment, and the dose (27,107). Among these factors, the dose received by the thyroid is the most important, because risk increases linearly with an increasing dose. Age at the time of radiation exposure is an important modifier of radiation risk, with younger age at exposure associated with greater radiation risk for thyroid carcinoma. There is a greater spontaneous thyroid carcinoma risk for women than men, but it is uncertain whether radiation increases the risk for thyroid carcinoma equally for women and men. It also remains somewhat unclear whether a fractionated radiation exposure carries less risk than an acute exposure. The prolonged exposure resulting from the Chernobyl accident suggests that, with respect to thyroid cancer, the two are about equivalent.
External irradiation formerly was used to treat a wide range of benign conditions during childhood, but is rarely used now (39,108). Indications for these included enlargement of the thymus, tonsils, adenoids, and cervical lymph nodes, as well as pertussis, asthma, bronchitis, tinea capitis, and acne. In
most cases the radiation treatment resulted in an increased risk for thyroid carcinoma. Other commonly used forms of radiotherapy involved local application of radioactive plaques to treat hemangiomas, other localized lesions, and enlarged tonsils, impaired eustachian tube function and hearing loss using radium rods inserted through the nose into the nasopharynx. Following treatment for hemangioma, the mean dose to the thyroid was about 0.2 Gy, and the ERRs were significantly increased (48,49) and consistent with those from the pooled analysis of external radiation (27). In contrast, treatment with nasopharyngeal radium rods resulted in very low doses to the thyroid (mean dose <0.02 Gy) and no excess of thyroid carcinomas (109,110,111).
most cases the radiation treatment resulted in an increased risk for thyroid carcinoma. Other commonly used forms of radiotherapy involved local application of radioactive plaques to treat hemangiomas, other localized lesions, and enlarged tonsils, impaired eustachian tube function and hearing loss using radium rods inserted through the nose into the nasopharynx. Following treatment for hemangioma, the mean dose to the thyroid was about 0.2 Gy, and the ERRs were significantly increased (48,49) and consistent with those from the pooled analysis of external radiation (27). In contrast, treatment with nasopharyngeal radium rods resulted in very low doses to the thyroid (mean dose <0.02 Gy) and no excess of thyroid carcinomas (109,110,111).
Currently, external radiation of the neck for malignant conditions such as childhood cancer, Hodgkin’s disease, and carcinoma of the larynx is used widely. They often result in subclinical or overt hypothyroidism, with changes in thyroid volume and hormone levels seen as early as 6 months (112,113). Such treatments in childhood also increase the risk for nodular thyroid disease and thyroid carcinoma (54,60,114,115). At very high doses (20 to 30 Gy), where cell killing may outweigh carcinogenesis, the radiation risk for thyroid carcinoma starts to decrease, but it remains significantly elevated.
With the increasing use of diagnostic radiologic examinations, particularly CT scans in children, some patients are receiving thyroid doses at which significant increase in thyroid cancer risk might occur (39). Prospective studies are not yet available to evaluate this directly. A large case-control study in Sweden (484 cases of thyroid carcinoma and 484 controls), completed before the wide-spread use of CT scans, found no association with prior diagnostic radiography (116). A smaller case-control study from Sweden, using medical records and dentist cards to estimate radiation exposure, reached the same conclusion (117). Given the close relationship between thyroid cancer risk and dose, it is important to obtain the best radiation history possible in order to decide how to monitor the exposed thyroid and, specifically, whether the use of thyroid ultrasound is required.
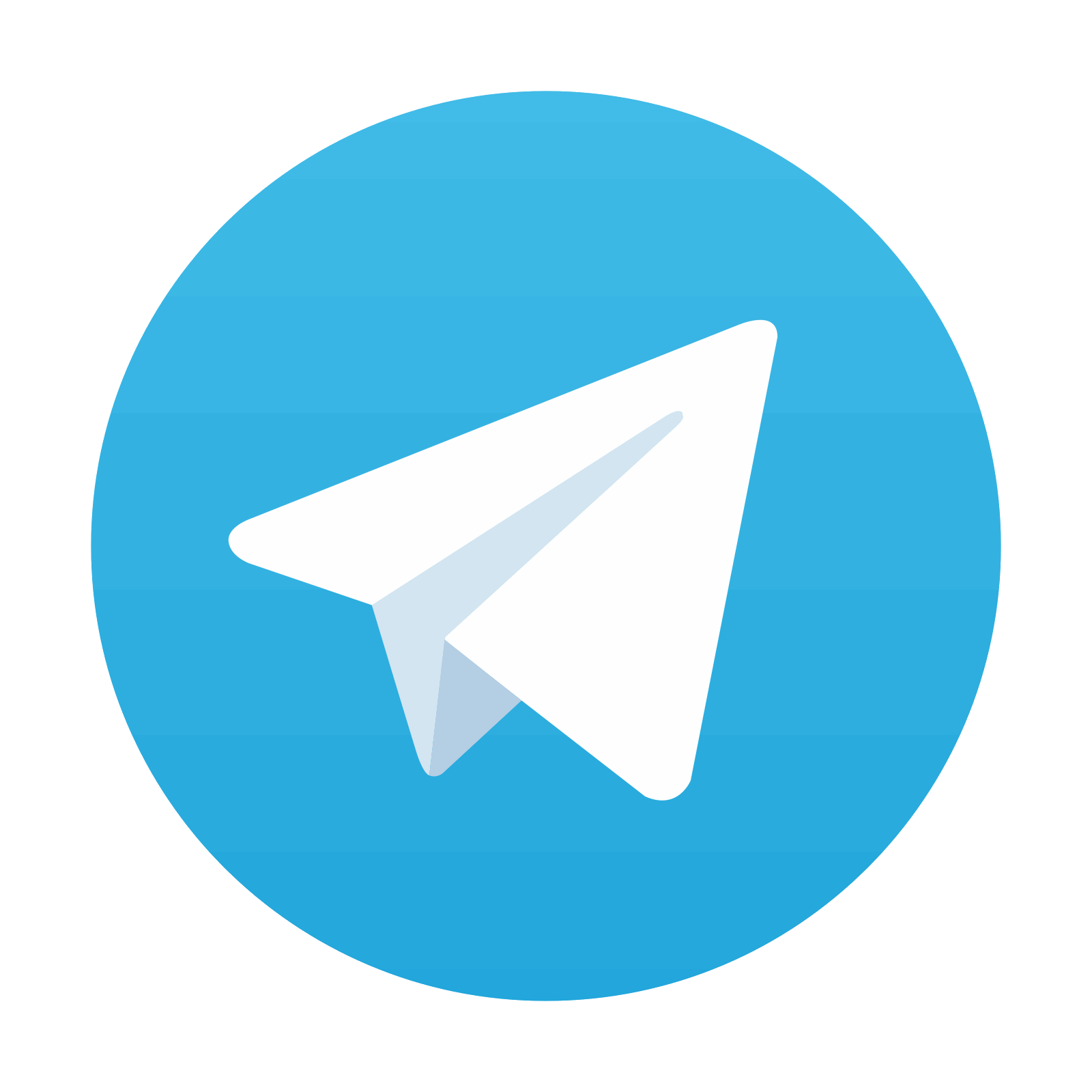
Stay updated, free articles. Join our Telegram channel

Full access? Get Clinical Tree
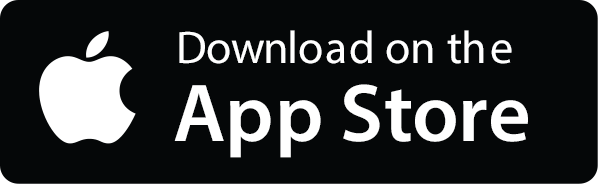
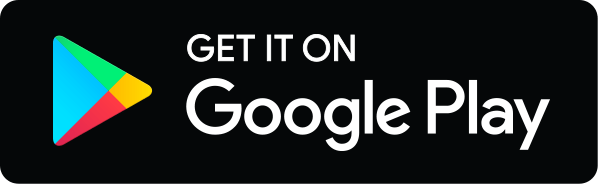