Cancer-Related Thrombosis
Anna Falanga
Agnes Y. Y. Lee
Frederick R. Rickles
The close association between malignant disease and thrombosis is well known and was first described more than a century ago by Trousseau, a French doctor who first observed that unexplained episodes of migratory thrombophlebitis in patients with gastrointestinal symptoms might indicate an underlying, undiagnosed tumor. Subsequent postmortem studies showed an increased incidence of thromboembolic disease in cancer, particularly in patients who died of mucinous cancer of the pancreas, lung, and gastrointestinal tract. On the other hand, we know today that venous thromboembolism (VTE) may be indeed the first manifestation of an occult malignancy in an otherwise healthy individual, anticipating the clinical detection of tumor by months or even years. Unlike thrombosis in other settings, venous thrombosis in cancer can be migratory, may involve superficial as well as deep veins, may affect unusual sites such as the arms and chest, and may be relatively unresponsive to standard anticoagulant therapy. However, thromboembolism is not the only form of abnormal hemostasis found in patients with cancer. Also of importance is the bleeding diathesis that results from consumption of platelets and clotting factors as occurs in disseminated intravascular coagulation (DIC). Cancer patients commonly present with abnormalities in laboratory coagulation tests, indicating an ongoing subclinical hypercoagulable condition. The results of laboratory tests demonstrate that a process of fibrin formation and removal parallels the development of malignancy, which is of particular interest since fibrin and other clotting products are of importance for both thrombogenesis and tumor progression. Many factors can increase the thrombotic risk in cancer patients, including classical risk factors such as immobility, old age, surgery, and cancer-specific factors such as advanced disease stage, and cancer therapies. In addition, tumor cell-specific prothrombotic properties and the subsequent host cell inflammatory response dramatically enhance the risk for thrombosis in these patients. Cancer cells produce and release procoagulant and fibrinolytic proteins, as well as inflammatory cytokines. In addition, they are capable of directly adhering to host cells (i.e., endothelial cells [ECs], monocytes, platelets, and neutrophils), thereby stimulating additional prothrombotic properties of the host effector cells. Notably, most of these mechanisms, in addition to generating thrombosis, can also promote tumor growth and metastasis.
EPIDEMIOLOGY
VTE, including deep vein thrombosis (DVT) and pulmonary embolism (PE), is the most extensively studied thrombotic complication associated with malignant disease. However, arterial thromboembolism, migratory thrombophlebitis, thrombotic nonbacterial endocarditis, and DIC add to the spectrum of complications. The incidence of VTE in cancer populations has been estimated at approximately 1 out of 200 patients,1 and active malignant disease is an independent risk factor for VTE.2, 3 Overall, 18% to 29% of all VTE events in the population are associated with cancer.4, 5, 6 In a registry of 5,451 patients hospitalized with objectively confirmed DVT, cancer was reported in 39% of patients; of these 62% had ongoing cancer and 38% a history of cancer.7 Several large retrospective analyses of hospital discharge data reported a 28% to 36% increase in VTE events over the period 1995 to 2003.8, 9 Hospitalization, anticancer surgery, and active treatment regimens, particularly chemotherapy, further increase the risk of thrombosis in cancer patients.2, 8, 10
The Risk of VTE Associated with Cancer
Large, population-based studies have shown that the risk of VTE is increased between four- and sevenfold in patients with malignancy,2, 11 and studies based on hospital discharge have reported an overall VTE incidence of 0.6% to 7.8% in patients with cancer, more than double that in patients without cancer.8, 9, 12, 13, 14 Recently, a retrospective cohort study of nearly 2 million patient hospitalizations in the United States reported an overall VTE rate of 4.1% among 1,015,598 cancer patients.8 Nevertheless, the incidence of VTE in patients with cancer is probably underestimated by retrospective, population-based studies that report only symptomatic or objectively confirmed VTE events. Routine-staging CT scans in cancer patients have reported a high prevalence of unsuspected PE,15, 16, 17 for example, in 4% of inpatients and 0.9% of 589 outpatients.15
The increased risk of VTE associated with cancer portends an increased likelihood of VTE recurrence. For example, in a retrospective analysis of 1,303 patients with symptomatic VTE receiving oral anticoagulant therapy, patients with cancer (n = 264) experienced greater VTE recurrence compared with patients without malignancy (27.1 vs. 9 events per 100 patient-years, P = 0.003).18 Likewise, a prospective follow-up study of 842 patients with confirmed DVT, with 181 patients with cancer and 661 without cancer, reported an annual VTE recurrence rate of 20.7% in cancer patients compared with 6.8% in patients without malignancy.19 These studies also found that cancer patients are more likely to suffer bleeding complications during VTE treatment,18, 19 both bleeding and recurrence being correlated with the extent of cancer.19
Among cancer patients, those experiencing a VTE event have a worse survival outcome compared to thrombosis-free subjects.8, 9, 20, 21 A report from the Danish Cancer Registry highlighted a mortality ratio of 2.2 in cancer patients with symptomatic
VTE compared with matched cancer patients without VTE.21 A retrospective analysis of 68,142 patients reported that VTE was a significant predictor of mortality within 1 year of colorectal cancer diagnosis among patients with local (hazard ratio [HR] 1.8, 95% confidence interval [CI] 1.4 to 2.3) and regional malignancies (HR 1.5, 95% CI 1.3 to 1.8),22 and a necropsy review revealed that 21.8% of fatal PE events occurred in hospitalized medical cancer patients.23
VTE compared with matched cancer patients without VTE.21 A retrospective analysis of 68,142 patients reported that VTE was a significant predictor of mortality within 1 year of colorectal cancer diagnosis among patients with local (hazard ratio [HR] 1.8, 95% confidence interval [CI] 1.4 to 2.3) and regional malignancies (HR 1.5, 95% CI 1.3 to 1.8),22 and a necropsy review revealed that 21.8% of fatal PE events occurred in hospitalized medical cancer patients.23
These studies are strengthened by findings from the RIETE registry of 14,391 patients with symptomatic, acute VTE, in which the 2,945 patients with cancer had significantly higher rates of fatal PE (2.6% vs. 1.4%) and fatal bleeding (1.0% vs. 0.3%) than those without cancer.24 Among 512 patients who presented with upper-arm DVT,25 those with cancer had a higher rate of recurrent VTE (6.1% vs. 2.8%, odds ratio [OR] 2.2), major bleeding (4.1% vs. 0.9%, OR 4.4), and mortality (22% vs. 3.5%, OR 7.8) than did patients without cancer. The risk of VTE steadily accumulates with each additional patient-related thrombotic risk factor in cancer patients, for example, advanced age, prolonged immobility, prior history of VTE, and comorbid conditions of infection and cardiopulmonary disease.2, 9, 19, 26
The Incidence of VTE According to Tumor Type and Stage
Cancer with the highest rates of VTE include those involving the pancreas, stomach, and kidney,27 and studies based on hospital discharge data confirm autopsy data that link VTE with tumors of the brain, pancreas, ovary, lung, and uterus and leukemia9, 28 (FIGURE 125.1). Among the hematologic malignancies, lymphoma and myeloma have the highest rates of VTE.8 A retrospective cohort study of 66,106 adult neutropenic cancer patients reported an overall rate of symptomatic VTE of 8%: among these, patients with lymphoma and leukemia represented a third of all cases of VTE and almost half of the arterial thrombotic events.9 Advanced metastatic cancer is associated with an increased risk of VTE compared with localized tumors.27 Data from the California Cancer Registry (1993 to 1995) highlighted metastatic disease as the strongest predictor of VTE complications.20 Similarly, Blom et al.28 reported that cancer patients with distant metastases had almost a twofold increased risk of VTE compared with those without metastases.
The Effect of Anticancer Treatments on VTE Incidence
Many anticancer treatments increase the risk of VTE, including surgical procedures, nonsurgical treatments, and use of a central venous catheter (CVC).29
Surgery
In 1975, V.V. Kakkar et al. analyzed 4,031 patients undergoing major abdominal surgery; half of these received low-dose unfractionated heparin (UFH) to prevent thromboembolism. Overall, 170 (4.2%) of patients died postoperatively. The autopsies, performed in about 70% of patients who died after surgery, demonstrated the great benefit of heparin prophylaxis. In particular, among the 23% of patients operated for malignant disease, the frequency of fatal PE was 1.6% in the control group and 0.4% in the heparin group.30 Patients with cancer are at increased risk (i.e., three- to fivefold) for postoperative thrombosis compared to noncancer patients.31 Without prophylaxis, the postoperative incidence of symptomatic or asymptomatic DVT in cancer patients is 40% to 80% and that of proximal DVT 10% to 20%.30 Data from the @RISTOS registry identified VTE as the most common cause of death 30 days after surgery in cancer patients (46%); 40% of VTE events occurred >21 days after surgery.10
Nonsurgical Cancer Treatments
Nonsurgical cancer treatments are associated with a high incidence of VTE, including the use of chemotherapy, adjuvant chemotherapy, hormones, and antiangiogenic agents. In a population-based study of VTE risk factors among 625 patients, malignant disease increased the risk of VTE 4.1-fold, but with chemotherapy it increased by 6.5-fold.2 By contrast, radiotherapy has not been proven to increase the risk of VTE.28, 32
Chemotherapy and Hormonal Therapy
A prospective study of nearly 4,500 patients receiving outpatient chemotherapy reported a 2.7-fold increase in arterial thrombosis, and a 47-fold increase in the mortality rate from VTE compared with the general population.33 At present, it is not known if specific chemotherapeutic agents carry a higher risk of thrombosis. An elevated prechemotherapy platelet count is associated with an increased risk of VTE.32 There is a cause-and-effect relationship between L-asparaginase use and thrombosis,34 and high-dose corticosteroids are associated with a 3.5-fold increased risk of VTE.35 Women who receive the selective estrogen receptor modulator tamoxifen have a 1.5- to 7.1-fold increase in the risk of developing symptomatic VTE, compared with placebo or no treatment.36
Adjuvant Chemotherapy
The incidence of VTE in women with early-stage, node-negative, or node-positive breast cancer receiving chemotherapy treatment is 0.9% to 9.6%,37 with the greatest risk seen in older postmeno-pausal women.38 In 1988, Levine et al.39 published the results of a randomized multicenter trial comparing chemotherapy with chemohormonal therapy in 205 women with stage II breast cancer. There were 14 objectively confirmed symptomatic VTE events distributed in both groups, and all occurred during active treatment. Anastrozole, a third-generation aromatase inhibitor used as an alternative agent for adjuvant treatment in women with early breast cancer and a low risk of recurrent tumors,37 may be associated with a lower risk of VTE complications than tamoxifen.40
Antiangiogenic Agents
Targeted antiangiogenic agents, such as bevacizumab, the monoclonal antibody to vascular endothelial growth factor (VEGF), have shown efficacy in improving survival rates among patients with advanced malignant disease.41, 42, 43 However, the addition of bevacizumab to chemotherapy regimens is associated with a high incidence of thrombotic events. A recent meta-analysis from 15 randomized controlled trials of 7,956 patients with advanced solid tumor indicated that bevacizumab was associated with an increased risk of VTE, with a relative risk of 1.33.44 Similarly, treatment regimens involving thalidomide or lenalidomide are associated with an increased risk of VTE when used concomitantly with chemotherapy or corticosteroids in patients with multiple myeloma.45, 46
Supportive Therapy
The use of recombinant human erythropoietin (EPO) and other hematopoietic growth factors as supportive therapy in cancer patients appears to increase the risk of VTE. A study in 147 patients with nonmetastatic cervical or vaginal cancer showed that EPO increases the risk of thrombosis by 15-fold.47 The use of EPO and granulocyte growth factors is independently associated with VTE risk among patients on chemotherapy. 32 Red blood cell transfusion may increase the risk of VTE, one study reporting that 7.2% of patients receiving transfusions developed VTE compared with 3.7% of patients who did not receive transfusions; transfusions were also associated with an increased risk of in-hospital mortality.48
Central Venous Catheters
CVCs promote thrombosis through a number of mechanisms such as trauma to vessel wall, and by local high drug concentrations delivered by the CVC.49 Retrospective studies indicate a risk of CVC-related thrombosis in malignancy of 12% to 66%, depending on the type of cancer and its treatment, and the type, position, and duration of catheter use.50 However, recent randomized controlled trials in cancer patients report lower rates of CVC-related thrombosis, 3.1% to 4.0% symptomatic and 18.1% asymptomatic. The use of warfarin, dalteparin, or enoxaparin did not reduce the incidence of catheter-related DVT.51, 52, 53 The RIETE registry revealed that among patients diagnosed with an objectively confirmed symptomatic DVT, upper-extremity DVT was more frequent in patients with cancer than in those without.25 Upper-extremity DVT typically presents as symptomatic PE less often than lower-extremity DVT (9% vs. 29%), but is associated with a higher rate of recurrent VTE (6.1% vs. 2.8%) and overall mortality (22% vs. 3.5%).25
PATHOPHYSIOLOGY AND RISK ASSESSMENT
Patients with cancer display many types of hemostatic disorders,39 including overt hemorrhage, thrombosis, and DIC, or only a finding of hemostatic abnormality recognized as the result of laboratory testing that reflects an in vivo hypercoagulable state.
The Hypercoagulable State
The underlying principle in patients with cancer is that the clotting system is systemically activated, coagulation factors are consumed, and fibrinolysis is activated. Approximately half of all patients with cancer, and as many as 90% of those with metastases, exhibit abnormalities in one or more routine coagulation parameters.54, 55, 56, 57 The development of novel laboratory tests with increased sensitivity has enabled the detection of subtle alterations in the hemostatic system. Levels of plasma markers of activation of coagulation, such as the thrombin-antithrombin (TAT) complex, prothrombin fragment 1+2 (F1+2), fibrinopeptide A (FPA), and D-dimer, tend to be universally elevated in patients with cancer,58, 59 indicating the presence of in vivo thrombin generation and fibrin formation and fibrinolysis. These abnormalities can worsen with the start of antitumor therapies.60 For example, Falanga et al.61 demonstrated that the plasma levels of F1+2 and plasminogen activator inhibitor type 1 (PAI-1) in patients with non-Hodgkin lymphoma and multiple myeloma were elevated at baseline and significantly increased after receiving high-dose cyclophosphamide, followed by granulocyte colony-stimulating factor (G-CSF) for hematopoietic stem cell mobilization (FIGURE 125.2).
Hemostatic Markers and Risk of VTE
Few prospective studies have evaluated the utility of serial measurements of hemostatic markers for predicting the occurrence of VTE (as confirmed by objective test) in cancer patients. One such study of patients undergoing surgery for abdominal cancer found that presurgical TAT complex levels were significant predictors of postoperative DVT.62 Khorana et al. showed that a prechemotherapy platelet count >350,000/µL is predictive of subsequent thrombosis during chemotherapy,32 and data from the Vienna Cancer and Thrombosis Study (CATS) show that a P-selectin level above the 75th percentile is predictive of thrombosis in cancer patients63 and increased levels of both D-dimer and F1+2 characterize the highest risk of VTE in patients with cancer.64
Improvements of laboratory techniques allow the measure of plasma microparticles (MPs), the small vesicles shed by platelets,
leukocytes, and endothelium in response to cell activation. The MPs are characterized by a diameter of <1 µm, the presence on their surface of antigens of the parental cell, and by procoagulant properties. The procoagulant capacity is related to the presence of higher amount of the anionic plasma membrane phospholipid phosphatidylserine and of tissue factor (TF). Toth et al.65 showed that levels of MP derived from platelets are elevated in patients with breast cancer, compared to patients with benign breast lesions, and Trappenburg et al.66 showed that the levels of circulating platelet and EC MPs in patients with essential thrombocythemia increased with the number of risk factors for thrombosis. TF-bearing MPs are elevated in 60% of cancer patients with VTE and in 27% of those without VTE, thus predicting a significantly increased risk of VTE.67, 68 A large prospective study is currently assessing the predictive value of determining TF-rich MP levels, along with other factors, in patient populations with cancer at high risk for developing VTE (Clinicaltrials.gov NCT00876915). The MicroTEC study (Microparticles and Thromboprophylaxis with Enoxaparin in Cancer Study, Clinicaltrials.gov NCT00908960) is evaluating the benefit of primary thromboprophylaxis in patients with advanced colon, lung, or pancreatic cancer, administered on the basis of their level of TF-bearing MPs.69
leukocytes, and endothelium in response to cell activation. The MPs are characterized by a diameter of <1 µm, the presence on their surface of antigens of the parental cell, and by procoagulant properties. The procoagulant capacity is related to the presence of higher amount of the anionic plasma membrane phospholipid phosphatidylserine and of tissue factor (TF). Toth et al.65 showed that levels of MP derived from platelets are elevated in patients with breast cancer, compared to patients with benign breast lesions, and Trappenburg et al.66 showed that the levels of circulating platelet and EC MPs in patients with essential thrombocythemia increased with the number of risk factors for thrombosis. TF-bearing MPs are elevated in 60% of cancer patients with VTE and in 27% of those without VTE, thus predicting a significantly increased risk of VTE.67, 68 A large prospective study is currently assessing the predictive value of determining TF-rich MP levels, along with other factors, in patient populations with cancer at high risk for developing VTE (Clinicaltrials.gov NCT00876915). The MicroTEC study (Microparticles and Thromboprophylaxis with Enoxaparin in Cancer Study, Clinicaltrials.gov NCT00908960) is evaluating the benefit of primary thromboprophylaxis in patients with advanced colon, lung, or pancreatic cancer, administered on the basis of their level of TF-bearing MPs.69
Risk Stratification
The interaction and relative effects of the risk factors associated with VTE in cancer patients is highly complex, making the pretreatment assessment of VTE difficult. Several demographic, cancer-associated and treatment-related factors increase the risk of thrombosis in patients with cancer (FIGURE 125.3). A VTE risk assessment model for use in patients undergoing chemotherapy patients described in 2008 by Khorana et al. is based on five predictive variables, namely, cancer site, platelet count, hemoglobin level (or the use of erythropoiesis-stimulating agents), leukocyte count, and body mass index,70 and is under evaluation in a prospective cohort of cancer patients initiating outpatient chemotherapy but not receiving thromboprophylaxis.71 Preliminary results show that 27% of cancer outpatients deemed at high risk with this prediction rule developed VTE. Recently, Pabinger et al. expanded the Khorana model70 by adding P-selectin and D-dimer quantification, allowing an even better stratification of thrombotic risk. In particular, the HR of patients with the highest score compared with those with the lowest score was 25.9.72
THE PATHOGENESIS OF THE HYPERCOAGULABLE STATE
The pathogenesis of the prothrombotic state of cancer patients is due at least in part to the ability of cancer cells to activate the coagulation system and interactions that may favor tumor progression (FIGURE 125.4).
The Activation of the Hemostatic System by Tumor Cells
Several complex and not fully recognized interactions between malignant cells and coagulation shift the hemostatic balance toward a procoagulant state in multiple ways.73
Tumoral Production of Hemostatic Factors
Procoagulant Proteins
TF is a transmembrane glycoprotein that is the primary initiator of hemostasis. TF forms a complex with activated factor VII (TF/FVIIa) to trigger coagulation by proteolytically activating
FIX and FX. ECs and monocyte-macrophages do not normally express TF, unless they are stimulated.74 Differently, constitutive TF expression characterizes numerous malignant tumor tissues. In addition, TF expression can be induced on tumor- associated macrophages and EC either by inflammatory endogenous agonists, that is, tumor necrosis factor-alpha (TNF-α) and interleukin-1 beta (IL-1β), or by exogenous agonists, that is, bacterial endotoxin or tumor-specific antigens. In breast cancer, the expression of TF in tumor stroma correlates with progression to invasive cancer. Similarly, the expression of TF by
glioma cell lines and glioma surgical specimens correlates with the histologic grade of malignancy.
FIX and FX. ECs and monocyte-macrophages do not normally express TF, unless they are stimulated.74 Differently, constitutive TF expression characterizes numerous malignant tumor tissues. In addition, TF expression can be induced on tumor- associated macrophages and EC either by inflammatory endogenous agonists, that is, tumor necrosis factor-alpha (TNF-α) and interleukin-1 beta (IL-1β), or by exogenous agonists, that is, bacterial endotoxin or tumor-specific antigens. In breast cancer, the expression of TF in tumor stroma correlates with progression to invasive cancer. Similarly, the expression of TF by
glioma cell lines and glioma surgical specimens correlates with the histologic grade of malignancy.
Cancer procoagulant (CP) is a cysteine protease that directly activates FX in the absence of FVIIa. CP is synthesized by malignant cells, and its activity has been found in extracts of different tumors.75, 76, 77 The presence of CP antigen as well as of CP procoagulant activity (PCA) have been identified in cancer patients’ sera. Although CP is found almost exclusively in malignant cells, its role as a tumor marker or as a factor predictive of clinical thrombosis has not been demonstrated. CP expression in acute promyelocytic leukemia blasts parallels the degree of malignant transformation and is lost upon cellular differentiation after therapy with all-transretinoic acid (ATRA). In contrast, cells resistant to ATRA maintain their malignant phenotype, including CP expression.78 Similar observations have been reported for breast cancer.79
MPs released by tumor cells have been associated with the hypercoagulable state of cancer. The first demonstration that tumor-shed vesicles possess PCA was made by Dvorak et al.80 in 1981, who showed that a mouse breast carcinoma cell line and a guinea pig hepatocarcinoma cell line released PCA in culture and in vivo, in association with small vesicles shed by the tumor cell surface. Circulating MPs have been described in the plasma of patients with various tumors, such as pancreas and breast adenocarcinoma, giant-cell lung carcinoma, colorectal cancer, and acute leukemia.81, 82, 83 MPs contribute to intravascular thrombin generation by exposure of phosphatidylserine and procoagulant proteins such as TF84 and may explain the increased TF level in the blood of cancer patients. Patients with colorectal cancer have twofold higher TF-positive MPs compared to healthy controls, and levels of MP correlate positively with the levels of D-dimer as a marker of clotting activation.85 Elevated MP-associated TF activity significantly relates to the development of VTE in patients with disseminated mucinous carcinoma.81
Fibrinolytic Proteins
Tumor cells possess both the urokinase-type and the tissue-type plasminogen activators and can also produce PAI-1 and PAI-2. There are specific receptors on tumor cells that support the assembly of the fibrinolytic components, thus facilitating the activation of the fibrinolytic cascade.86 In patients with leukemia, the expression of these activities by blast cells may play a role in bleeding symptoms. On the other hand, a defect in the generation of normal plasma fibrinolytic activity, for example, by increased production of PAI-1, has been found in patients with solid tumors and may represent another mechanism for these patients to develop VTE.59
Inflammatory Cytokines
Tumor cells synthesize and release a variety of cytokines including proinflammatory (TNF-α, IL-1β) and proangiogenic factors (VEGF, basic fibroblast growth factor [bFGF]). Cytokine overproduction also derives from inflammatory tissues as a part of the host-reactive process in response to the tumor. Most of these cytokines induce the expression of a procoagulant phenotype by monocytes and ECs. Monocyte activation occurs in malignant tissue both in vitro and in vivo, expressed, for example, as significantly more TF than in control cells.59 Cytokines stimulate ECs to increase the production of PAI-1 and upregulate EC surface TF expression and downregulate expression of thrombomodulin,87, 88 a potent anticoagulant that forms a complex with thrombin to activate the natural anticoagulant protein C.88
Adhesion Molecules
The expression of tumor cell-surface adhesion molecules and/or their receptors allows the direct interaction of these cells with host cells, including EC, platelets, and leukocytes. The attachment of tumor cells to vascular ECs is relevant to promote localized clotting activation to the vessel wall and to start thrombus formation. The adhesion can occur directly or via the cooperation of granulocytes. The interaction between tumor cells and ECs is mediated by different pathways of adhesion molecules expressed by different tumor types.89 In flow conditions, the interaction involves rolling and firm adhesion, depending on the respective adhesive properties of both ECs and tumor cells. Some tumor cell types roll and adhere using E-selectin, while others adhere without rolling using vascular cell-adhesion molecule-1.90 Thus, a variety of molecules expressed on EC surface, including selectins, integrins, and immunoglobulins, are necessary for the adhesion of cancer cells and their migration to distant sites to form metastases. P-selectin (CD62P) is an important cell-adhesion molecule expressed by stimulated ECs and activated platelets, which mediates heterotypic aggregation of activated platelets to cancer cells and the adhesion of cancer cells to stimulated ECs. In addition, P-selectin mediates the homotypic aggregation of activated platelets and the transfer of procoagulant MPs between cells, which further contribute to fibrin deposition and microthrombus formation.91
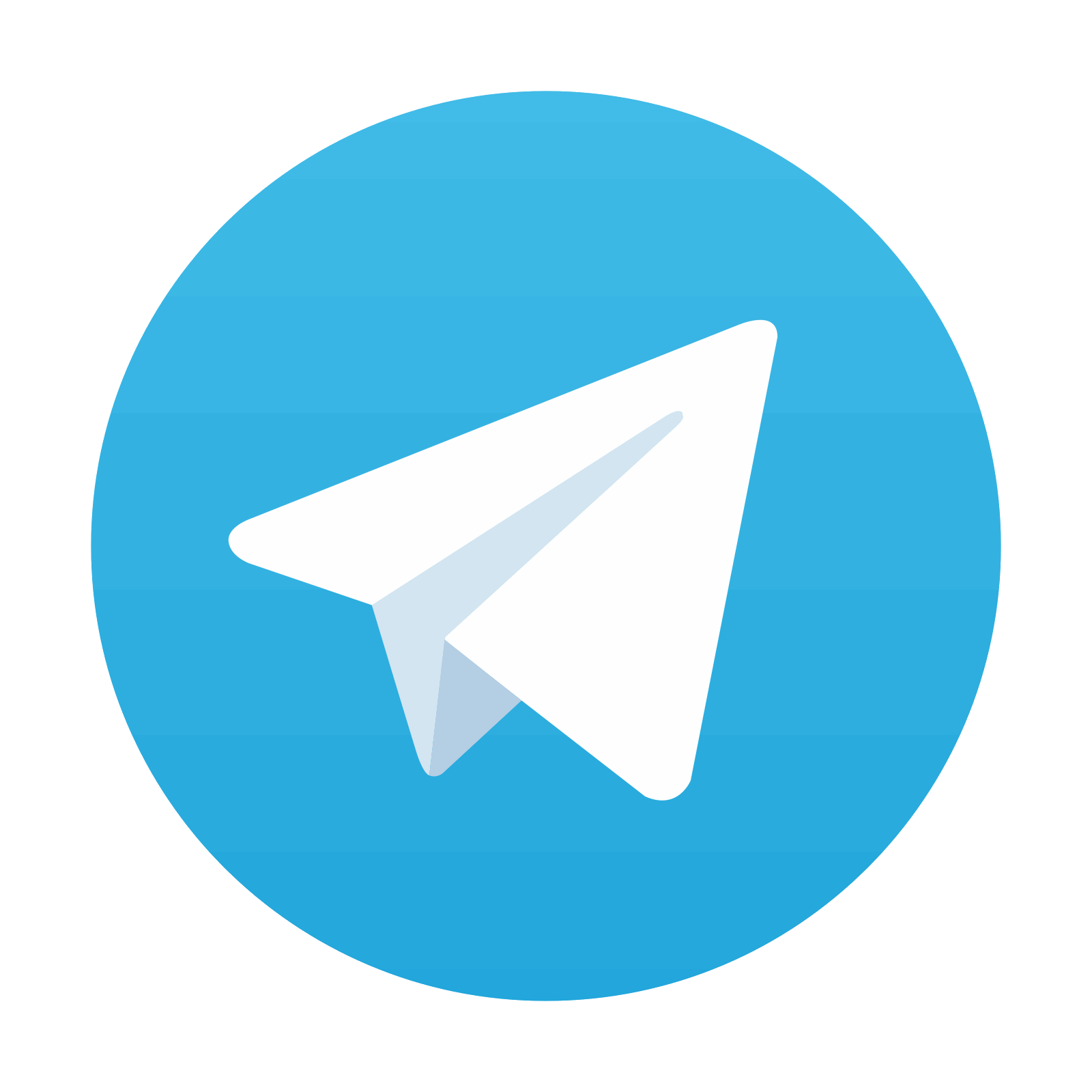
Stay updated, free articles. Join our Telegram channel

Full access? Get Clinical Tree
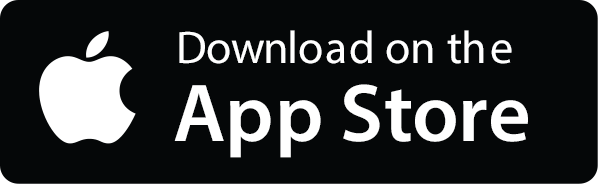
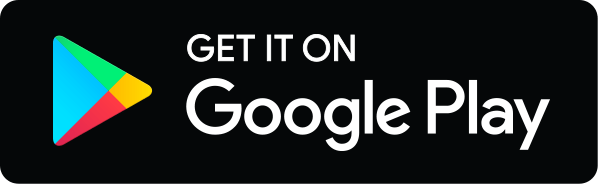
