Bone Marrow Micrometastases and Circulating Tumor Cells
Costanza Paoletti
Jeffrey B. Smerage
Daniel F. Hayes
INTRODUCTION
The development of distant metastases is the result of the spread of tumor cells through the lymphatic and vascular compartments. In breast cancer, the most important example of this process is the identification of tumor cells in the axillary lymph nodes. Therefore, lymph node staging continues to be one of the most important prognostic variables in early-stage breast cancer. However, axillary staging is far from definitive. Women with newly diagnosed breast cancer who have uninvolved axillary lymph nodes still have a 20% to 30% chance of recurrence. Conversely, even in the absence of adjuvant systemic therapy, roughly half of women with node positive cancer will not have recurrence of their cancer (1). This observation suggests that micrometastatic process might be independent of lymphatic involvement. Therefore, development of new methods to detect and characterize micrometastatic disease might improve the ability to make clinical treatment decisions.
Detection of micrometastases in visceral organs, such as liver or lung, is fraught with considerable logistical and safety concerns. In this regard, bone marrow and blood have been the most commonly studied non-lymphatic sites of micrometastases. The finding of micrometastases in bone marrow or blood is designated as “minimal detectable disease” (MDD). When detected in the bone marrow, MDD has also been called “disseminated tumor cells” (DTCs), while the term “circulating tumor cells” (CTCs) has been used to refer to micrometastases in the blood.
CLINICAL VALUE OF DETECTION OF MICROMETASTASES
Detection, enumeration, and characterization of MDD might have several clinical uses relative to breast cancer. However, to date, few if any practice guidelines have recommended either screening for or monitoring DTCs or CTCs in patients at risk for or affected by breast cancer (2, 3).
Why are these guidelines so conservative? There are several criteria that must be met before any tumor biomarker test, including those for MDD, are introduced into clinical care (4, 5 and 6). First, the specific use must be defined—risk categorization or screening of an unaffected person, or prognosis and/or prediction of benefit from therapy in the adjuvant or metastatic settings, or monitoring for occult metastases or for response or progression in patients who are either free of disease or are being treated for metastatic breast cancer. Second, the assay must have been shown to have analytical validity, which requires evidence of accuracy, reliability, and reproducibility (7). Finally, the biomarker test must have clinical utility. Clinical utility is defined as demonstration that use of the assay to direct patient care improves a patient’s outcome when compared to care of the patient without knowledge of the assay results (5, 6). High levels of evidence are required to established clinical utility. It is important to distinguish “clinical utility” from “clinical validity.” The latter implies that the biomarker test has been shown to separate two subgroups within a population according to outcome or biological characteristics, but the
assay may or may not be useful to care for patients. Taking these considerations in mind, the remainder of this chapter will review methods of detection of MDD and their analytical validity, and the potential clinical uses and evidence for clinical utility of tests for MDD.
assay may or may not be useful to care for patients. Taking these considerations in mind, the remainder of this chapter will review methods of detection of MDD and their analytical validity, and the potential clinical uses and evidence for clinical utility of tests for MDD.
METHODS OF DETECTION OF MINIMAL DETECTABLE DISEASE
The greatest barrier in detecting MDD is the accurate separation and identification of cancer cells from other cells that are found within the hematopoietic environment. In this regard, over the past two decades, several methods have been developed to enrich these cells from the bone marrow or hematopoietic environment. These methods have been based on either physical or biological properties, or both, that distinguish MDD from normal hematopoietic cells. Moreover, once the capture step has been concluded, further characterization is required to confirm that the presumed isolated cells are indeed at least epithelial, if not malignant. The most commonly used strategy is to demonstrate that the captured cells are epithelial in origin is by monitoring expression of cytoplasmic cytokeratin (CK) (8). Additional molecular evaluation can be conducted, investigating other established or putative biomarkers that might provide further biological or clinical insight.
Methods to Detect and Characterize DTCs in Bone Marrow
Detection and characterization of DTCs in marrow has involved an initial separation of nucleated cells through density centrifugation, followed by immunostaining for cytokeratin versus hematologic markers. The latter is usually performed on smears on glass slides. Further characterization for other markers of interest, such as tumor-associated antigens, can be performed using immunostaining, reverse transcriptase polymerase chain reaction (RT-PCR), or other molecular techniques (9, 10, 11, 12, 13, 14, 15 and 16). Standardized guidelines have been developed to reconcile different approaches of DTC quantification (17, 18).
TABLE 30-1 Characteristics and Techniques to Isolate and Identify CTCs | ||||||||||||||||||
---|---|---|---|---|---|---|---|---|---|---|---|---|---|---|---|---|---|---|
|
Methods to Isolate and Detect CTCs
Although malignant cells were identified in human blood over 150 years ago (19), the technology to capture and characterize CTCs with high analytical validity has only been available over the last decade. Over 40 different devices have been reported to isolate and characterize CTCs from whole blood (20). These are based on exploiting the differences between epithelial CTCs and normal hematopoietic cells in size, weight, electric charge, or flow characteristics or expression of epithelial or cancer-specific markers. Table 30-1 provides a description of these devices as of the publication of this chapter. A more detailed description of these devices is available in a recent review (20).
Currently, the most commonly used strategy to capture CTCs involves coating a solid phase matrix of some sort (magnetic or plastic beads, microposts, fluidic channels, etc.) with an antibody directed against a surface-expressed epithelial marker, usually the epithelial cellular adhesion molecule (EpCAM) (21, 22 and 23). The only FDA-approved, commercially available assay based on this strategy has been designated CellSearch® (Veridex, LLC; Raritan, NJ), and has been shown in several studies to be associated with worse prognosis in breast as well as colorectal and prostate cancer (24, 25 and 26).
However, this strategy is not perfect. EpCAM is only expressed by approximately 80% of all breast cancers, and the intra-patient expression of EpCAM by CTCs can be highly variable (27, 28). In this regard, EpCAM may be lost by cancer cells that are undergoing epithelial to mesenchymal transformation (EMT), a recently recognized process that may be a fundamental property of the metastatic phenotype (29). Nonetheless, of all the markers studied to date, this strategy is the most clinically practical method to capture epithelial CTCs, and, although not ideal, assays based on this strategy are associated with poor prognosis and can be used to monitor patients with metastatic breast cancer.
After the CTCs have been captured, they must be further characterized to prove that they are non-hematopoietic cells. In the case of most solid cancers, this additional characterization involves demonstration that they are of epithelial origin. Again, the most common strategy has been staining with 4, 6-diamidino-2-phenylindole (DAPI) to demonstrate
the presence of nuclei, and then with fluoresceinated antibodies directed toward CK and CD45 to demonstrate that they are epithelial and not hematopoietic, respectively.
the presence of nuclei, and then with fluoresceinated antibodies directed toward CK and CD45 to demonstrate that they are epithelial and not hematopoietic, respectively.
Over 30 other strategies to capture and characterize CTCs have been reported (20). These include use of membrane micropore filters or other devices that separate larger and more rigid epithelial cancer cells from smaller and more flexible hematopoietic cells (30). CTC have also been separated based on microfluidics techniques, which may also incorporate anti-EpCAM capture or not (31). An alternative method has involved smearing whole blood onto a specially charged slide and then identifying the epithelial cells (presumably CTC) by immunofluorescent staining (32, 33). Another approach is based on detection of specific proteins secreted only by viable cells using an adaptation of enzyme linked immunoassay technique (Epithelial ImmunoSPOT (EPISPOT) (34). This strategy permits generation of a “protein fingerprint” at the individual cell level.
CTCs can also be presumptively identified by determination of expression of epithelial or cancer-associated genes using RT-PCR (35, 36 and 37). Quantitative real-time RT-PCR (qRTPCR) may increase the specificity of this approach by differentiating mRNA derived from non-tumor and tumor cells. This strategy may be used with or without a prior epithelial enrichment. For example, in one commercially available assay (AdnaTestBreastCancer™), epithelial cells are isolated by immunomagnetic beads labeled with antibodies against MUC1 and EpCAM. Real-time PCR is then applied to quantify mRNA transcripts for a variety of epithelial-specific markers such as GA73.3, EpCAM, and human epidermal growth factor-2 (HER2) (38, 39 and 40).
As with bone marrow, the EPISPOT strategy has also been applied to whole blood to detect CTCs (41). This novel approach appears to have reasonable sensitivity and specificity relative to what has been reported with EpCAM capture, yet may provide an opportunity to characterize non-EpCAM expressing cells with greater clarity.
Each of these methods is predicated on evaluation of a certain volume of blood drawn from the patient and evaluated ex vivo. In order to monitor large volumes of blood, Eifler et al. have reported the high recovery of cultured human ovarian cancer cells that had been spiked into whole blood mononuclear fractions separated by leukapheresis (42). However, this approach is not terribly practical as a routine diagnostic assay. Recently, European investigators have reported pilot studies using an indwelling, intravascular guidewire coated with EpCAM monoclonal Ab (NanoDetector®, GILUPI, Greifswald Germany) (43). The catheter remains in vivo for 30 minutes, interrogating up to 1,500 mL of blood. This strategy permits collection of a substantially higher number of CTCs over a longer time window than is possible with a single blood draw. Direct comparisons to CellSearch® suggest increased sensitivity, but no data regarding clinical outcomes or clinical utility are available.
SENSITIVITY VERSUS SPECIFICITY IN DTC AND CTC ASSAYS
DTCs and CTCs are rare events in the bone marrow and circulation, respectively. For example, in bone marrow only 1 DTC can be detected for every 105 to 106 leukocytes (44). Likewise, CTCs are estimated to be present in a ratio of roughly one tumor cell per 1 × 105-7 peripheral mononuclear cells (45). Therefore, it is challenging to reliably separate DTCs and CTCs from the bone marrow and the blood, respectively. Sensitivity might be reduced for one of many reasons. First, most of the available strategies involve ex vivo, in vitro assays, limiting the volume of blood that can be interrogated. Second, any in vitro assay is fraught with cell loss due to device manipulation, such as flow through the device, incubations, and washings. Efforts to increase sensitivity may, with any assay, be hampered by loss of specificity, as discussed below.
Sensitivity of DTCs
Collection of DTCs requires a bone marrow aspirate and/or biopsy, which can easily be done at the time of surgery. However, for patients not undergoing surgery, bone marrow aspirate and biopsy is not terribly practical. Therefore, most DTC data are derived from patients with newly diagnosed breast cancer, and little if any data are available to estimate the incidence of DTCs in patients with metastatic breast cancer. Using standard techniques, DTCs can be detected in approximately one-third of patients with newly diagnosed, early-stage breast cancer, ranging from 12% to 42% depending on the study and the population selected (46). Following primary and adjuvant systemic therapy, it appears that the incidence of DTCs declines, presumably coincident with efficacy of therapy, although the clinical implications of this effect are not proven (46, 47 and 48).
The scant data available in metastatic patients suggest that approximately one-fifth or more have DTCs, even without documented bone metastases. Using the EPISPOT assay, the detection of cells that secret MUC1 and/or cytokeratin 19 allowed the detection of viable DTCs in 90% and 54% of patients with metastatic breast cancer and non-metastatic breast cancer, respectively (34).
Sensitivity of CTCs
CTCs are less commonly detected than DTCs in patients with early-stage breast cancer, regardless of the currently available assays used. Several investigators have reported that, using the CellSearch® system, sensitivity ranges between 10% and 25%, when positivity is defined as one or more CTC/7.5-22.5 mL whole blood (49, 50). RT-PCR methods appear to detect CTCs in approximately 41% (35, 51). Too few studies have been performed with the other assay techniques to provide reliable estimates.
In contrast, CTCs are commonly found in patients with metastatic breast cancer. Using CellSearch®, approximately 70%, 60%, and 50% of patients with metastatic breast cancer have one, two, or five or more detectable CTC/7.5 mL whole blood, respectively. Sensitivity using other assays may be higher.
Specificity of DTCs and CTCs
Specificity has both technical and biologic distinctions. Technically, it is essential to distinguish an identified cell from both normal constituents of the surrounding bone marrow or blood environment. Most assays accomplish this task with accuracy by staining for epithelial and hematopoietic markers, although it is essential to use non-specific quenching techniques to avoid false-positive staining of granulocytes (if using immunohistochemistry) and plasma cells (any technique with a secondary antihuman antibody step). To circumvent these issues of specificity, multiple markers are used for the positive identification of CTCs and dismissal of leukocytes.
It is important to appreciate that some presumed epithelial markers may also be transiently expressed by normal or undifferentiated hematopoietic components of the marrow and blood. For example, MUC1, which is the soluble protein captured by the commonly used CA15-3 and CA27.29
circulating assays, has been shown to be expressed by precursor bone marrow hematopoietic cells (52).
circulating assays, has been shown to be expressed by precursor bone marrow hematopoietic cells (52).
Even with careful attention to separation of epithelial from hematopoietic cells, on occasion, normal subjects are found to have circulating epithelial cells. For example, the CellSearch® assay captures epithelial cells in 1% of normal subjects (21, 53). However, analysis by fluorescence in situ hybridization (FISH) with multiple chromosomal markers has shown that captured epithelial cells from normal subjects are eusomic, while cells captured by CellSearch® in patients with metastatic breast cancer are almost always aneusomic (54, 55). Furthermore, each of the capture strategies described above is really only an imperfect enrichment step. Even if the assay clearly captures well-defined epithelial or malignant cells, secondary analytical steps that do not directly visualize the interrogated cell, such as omicsbased multi-gene RT-PCR, may well include contaminating white blood cells that have either simply not been removed or may even be phagocytosing, and therefore nearly covalently attached to, the CTCs.
Even single gene specific RT-PCR methods are plagued by false positive findings, ranging from 10% to 40% (22). These false-positive results are attributed to issues with laboratory technique, primer selection, and illegitimate expression of the target genes in leukocytes. For example, cytokeratin 19 and CEA overexpression can be induced in leukocytes by cytokines and growth factors (56, 57 and 58). Many strategies have been used to increase the specificity, such as the use of qRT-PCR, which increases specificity compared to nested RT-PCR. As with whole-cell capture devices, qRT-PCR methods are positive in approximately 2% to 6% of normal control populations (59).
In addition to technical specificity, biologic specificity hampers clinical studies of MDD. Biological specificity refers to the detection of DTCs or CTCs that are morphologically malignant, yet may not have the capacity to produce lethal metastases. In this case, the clinician runs substantial risk of overdiagnosis. Indeed, not all MDD has malignant potential. Although detection of MDD in lymph nodes (60), bone marrow (46), and blood (21, 50) is consistently associated with a statistically significantly higher risk of a future event, patients with positive findings are not absolutely destined to suffer recurrence, progression, or death in the future. For example, Wiedswang et al. have reported that approximately 15% of women who are free of any evidence of disease 2 to 3 years after initial diagnosis and treatment have positive bone marrow aspirates (47). Although this finding is associated with a statistically significant increase in subsequent relapse and death, many of these women did not suffer recurrence over the succeeding several years of follow-up. Likewise, Meng et al. have identified aneusomic CTCs in blood of approximately one-third of women who were 7 to 15 years after diagnosis but who were free of disease (55). These data suggest that one can identify morphologically distinct, viable DTCs and CTCs, but for one of many reasons they may not have long-term malignant potential. Thus, clinical studies demonstrating robust separation in outcomes are essential before a new assay claiming increased sensitivity is applied to patient care.
MINIMAL DETECTABLE DISEASE AND CLINICAL OUTCOMES
Over the last two decades, several studies have demonstrated that the presence of detectable DTCs or CTCs in patients with both primary and metastatic breast cancer is indicative of a worse prognosis. These studies establish clinical validity of the respective assays. However, only recently have appropriately designed studies been reported that begin to provide insight into clinical utility of these assays for their intended use; in other words, whether and how this information can be used in routine clinical care to improve patient outcomes. Many of the available studies are pilot in nature or conducted as correlative studies of convenience, in which the specimens happened to be available for a given assay. Most of these studies have significant limitations in their ability to assess the value of MDD as either a prognostic or predictive factor in breast cancer. These limitations are largely due to the small size of studies, retrospective acquisition of samples, and wide variations in treatments received by the patients. Thus, while analytical validity and clinical validity have been established for some of the assays (in particular, the CellSearch® system), high levels of evidence demonstrating clinical utility are still lacking.
Prognosis in Early-Stage and Metastatic Stage of Disease for DTCs
Almost all of the data regarding DTCs present in the literature have been generated in early-stage breast cancer and not in the metastatic setting. In a pooled analysis of all available studies, Braun et al. reported that approximately one-third of 4,703 patients with stage I-III breast cancer had detectable DTCs prior to surgery. They showed that the presence of occult cytokeratin positive metastatic cells in early breast cancer is associated with a statistically significantly higher risk of distant metastases and death from death-related causes (p < .001) (46). In multivariate analysis, the presence of DTCs was the strongest and most highly significant predictor of death (hazard ratio [HR] = 1.81), disease recurrence (HR = 1.85), and the development of distant metastases (HR = 2.03) (Fig. 30-1). These increased risks were seen in all treatment groups, including patients who only received hormonal therapy, or chemotherapy, and lowrisk patients (TNM stage T1N0M0) who did not received any adjuvant systemic therapy.
However, although the pooled analysis demonstrates clinical validity, the clinical utility of these findings is not clear. Given the nature of the pooled studies, adjuvant treatments were variable, representing the local standard of care. Furthermore, although statistically significant, the results may not be clinically relevant. For example, the magnitude of difference in outcomes between those with versus those without MDD was highest in patients who received adjuvant systemic therapy (AST), in particular chemotherapy (Fig. 30-2C and D). Because these patients already received AST, and in the absence of data supporting further or different therapy for such patients, knowledge of this residual risk is of little clinical value.
The most obvious clinical use would be to use DTCs to determine whether to give AST. In this regard, in patients who did not receive AST, the magnitude of the difference in breast cancer-specific survival (BCSS) and disease-free survival (DFS) (Fig. 30-2E and F) between those with positive versus negative nodes is quite small, although still statistically significant. Indeed, even though those with positive DTC had a slightly worse prognosis, it was still quite favorable even without AST. These observations suggest that detection of bone marrow micrometastases in early disease is simply recanting what the clinicians already suspected from analysis of the primary and lymph node status: that patients who received AST had a worse prognosis. Unfortunately, in the very patients for whom further prognostic information would be valuable, the assay was
not very informative. Furthermore, because these studies were not conducted to specifically address clinical utility, one cannot determine if lack of MDD might have identified patients whose primary and lymph node status suggested high risk of recurrence but who actually would have done well without AST.
not very informative. Furthermore, because these studies were not conducted to specifically address clinical utility, one cannot determine if lack of MDD might have identified patients whose primary and lymph node status suggested high risk of recurrence but who actually would have done well without AST.
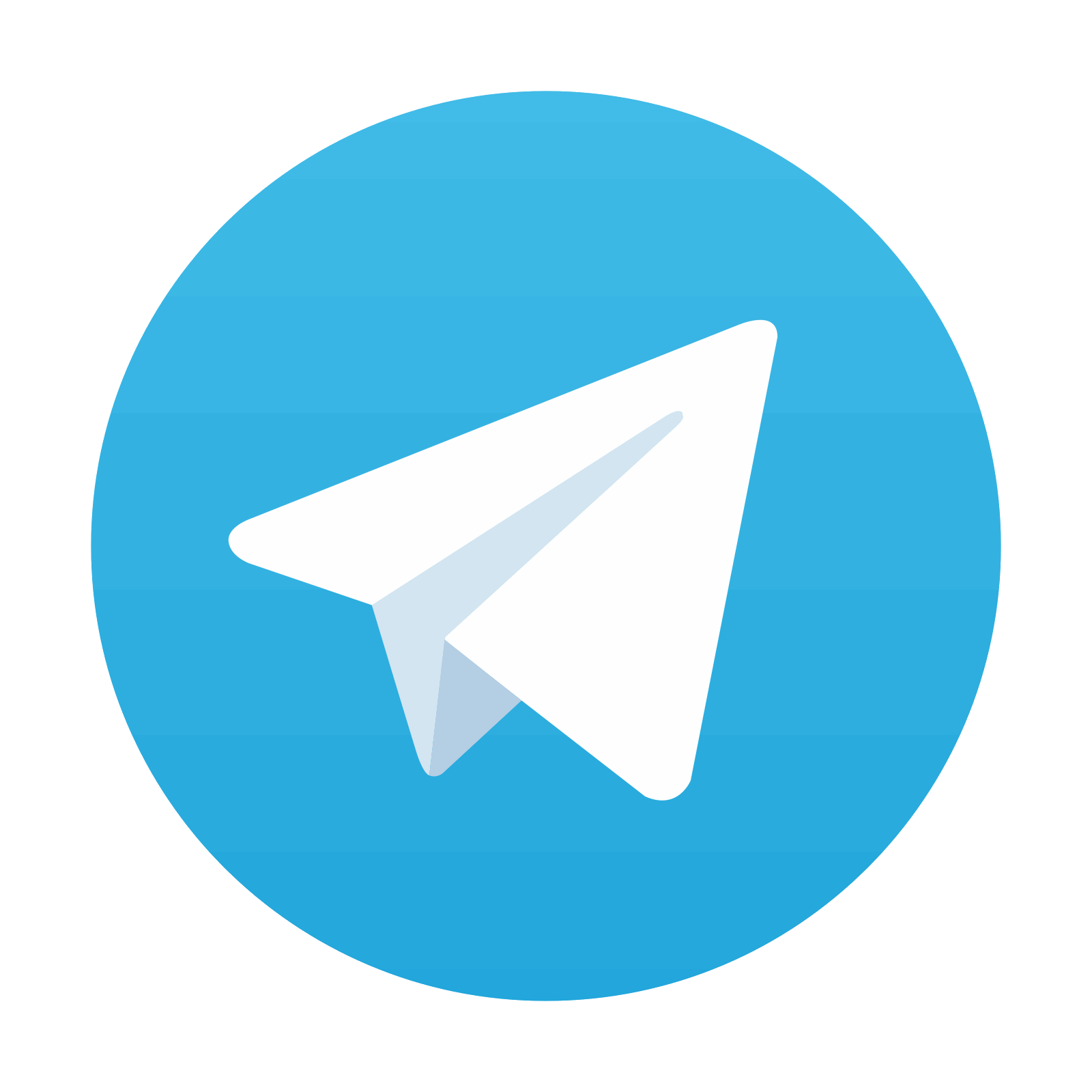
Stay updated, free articles. Join our Telegram channel

Full access? Get Clinical Tree
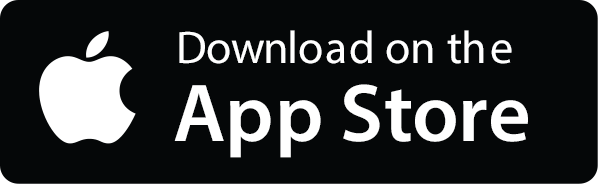
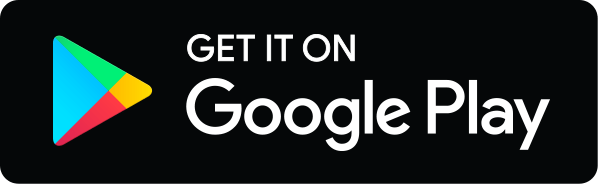