39.1
Introduction
The gut microbiota (GM) comprises the largest number of cells in the human body, and it is well established that the interaction between the GM and its host profoundly shapes many aspects of our physiology . However, it was not until 2012 the role of the GM for bone homeostasis was first recognized in a seminal publication ( Fig. 39.1 ; ). Thereafter, the number of published studies in the field of “GM” and “bone” has increased exponentially by time ( Fig. 39.2 ). Experimental animal studies, starting by the first evidence of a role of the GM for adult bone metabolism using the germ-free (GF) mouse model, followed by several sophisticated studies manipulating the normal GM composition, have clearly demonstrated that the gut microbiome is a key regulator of both postnatal skeletal development and adult bone metabolism in several different rodent models . These rodent models revealed that alterations in microbiota composition and host responses to the microbiota contribute to pathological bone loss, while changes in microbiota composition that prevent, or reverse, bone loss may be achieved by nutritional supplements with prebiotics or probiotics. A new term “osteomicrobiology” was introduced in 2015 for the rapidly emerging research field of the role of the microbiota in bone health and the mechanisms by which the microbiota regulates postnatal skeletal development, bone aging, and pathologic bone loss. This cross-disciplinary research field is aimed to bridge the gaps between bone physiology, gastroenterology, immunology, and microbiology . Although there are major recent advances regarding the possible mechanisms for the GM to influence adult bone metabolism, including involvement of the immune system, intestinal permeability, short-chain fatty acids (SCFAs), calcium absorption and serum insulin-like growth factor 1 (IGF-1), the exact detailed cellular and molecular mechanisms remain to be determined ( Fig. 39.3 ). The first promising human translation findings from the primary endpoints of two placebo controlled randomized clinical probiotic treatment trials were recently published, revealing that probiotic treatment may regulate bone mass also in humans .
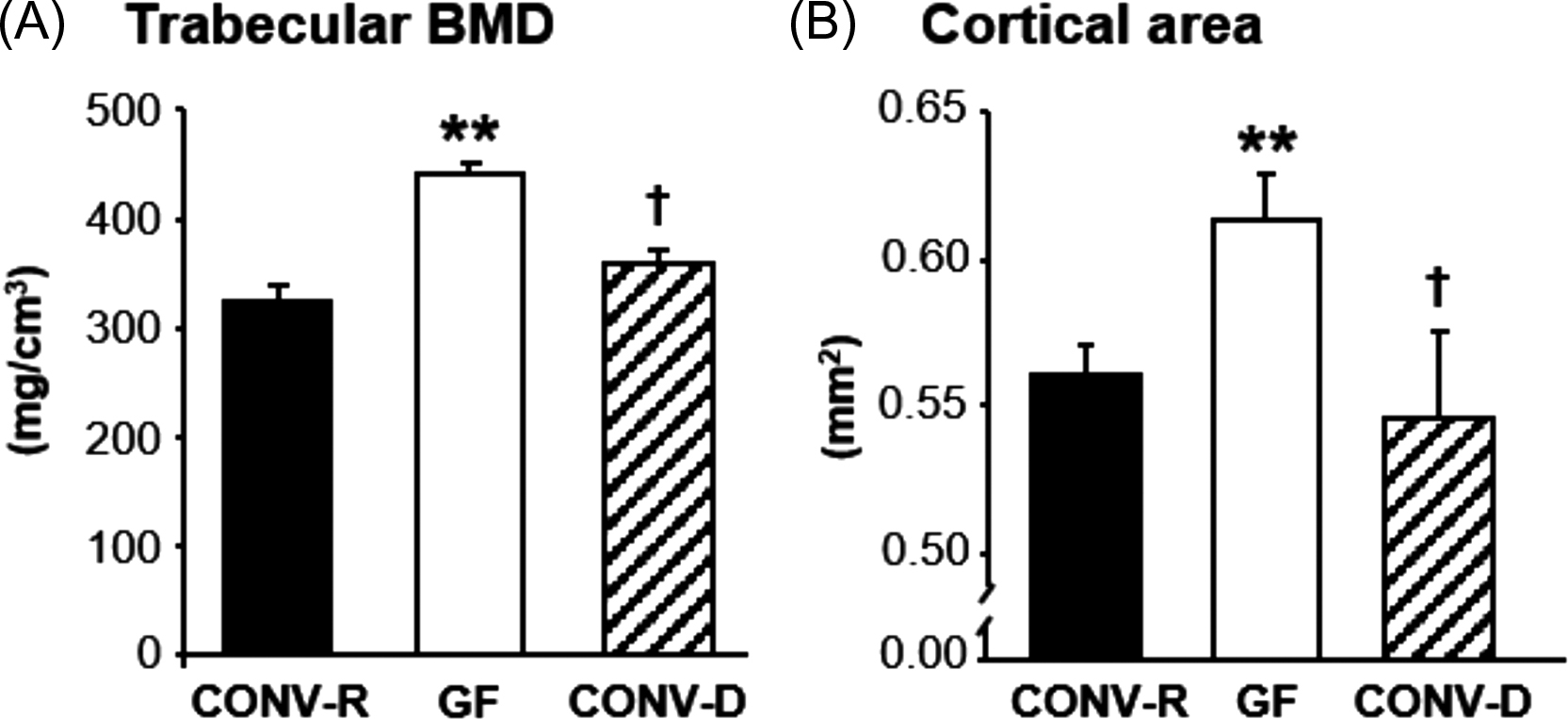
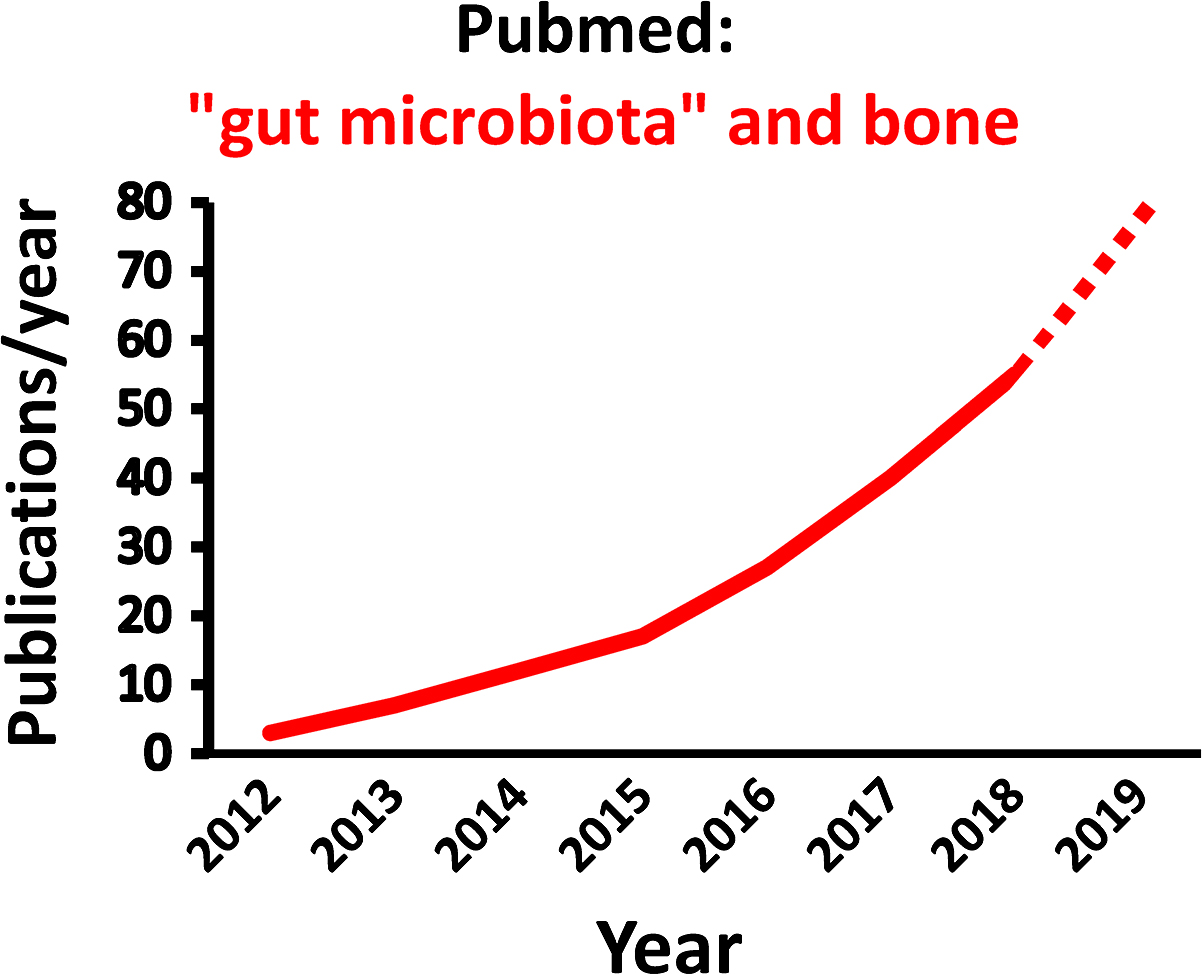
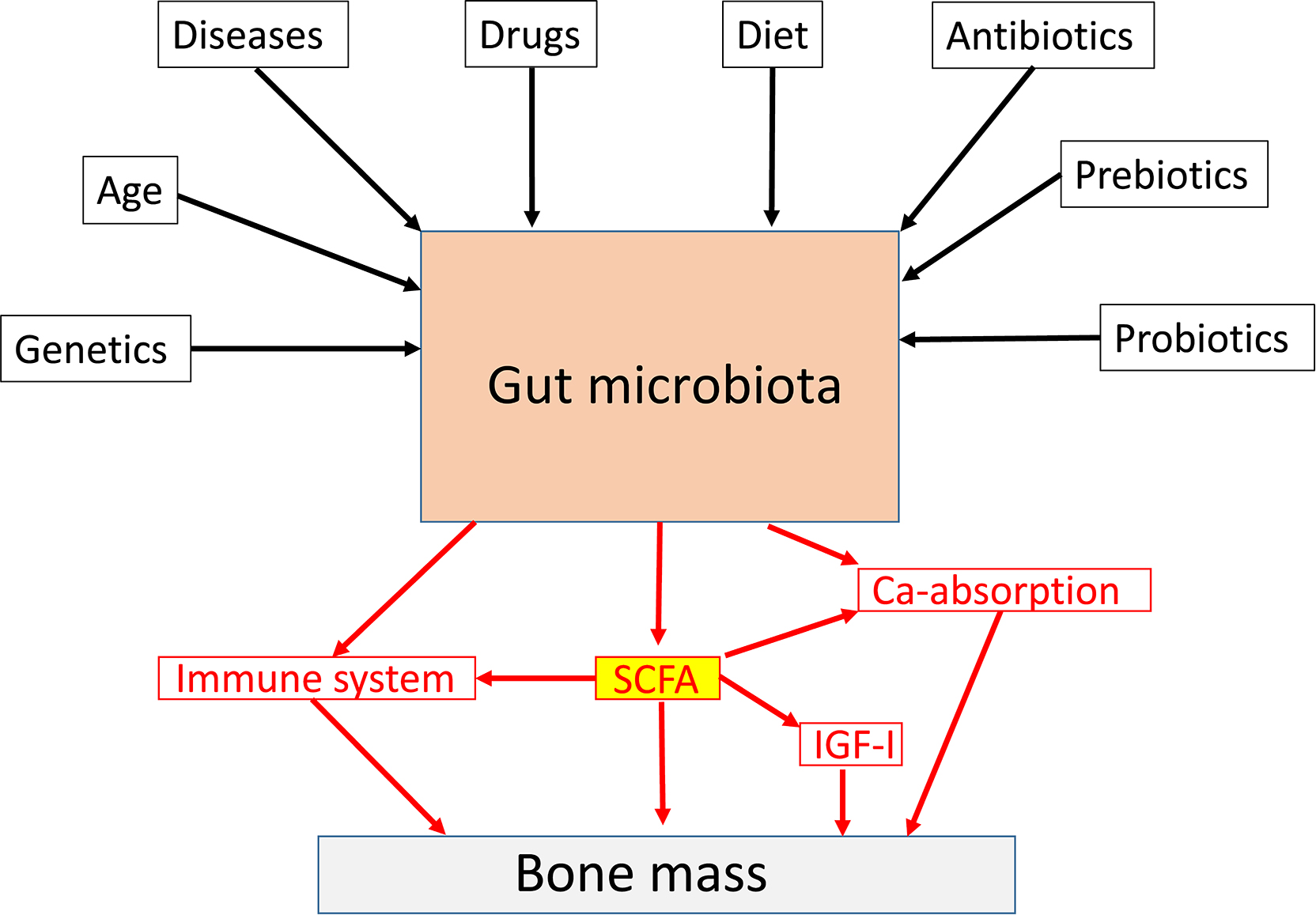
39.2
The gut microbiota could be considered as an additional organ
There are trillions of microbes in the GM, and in this chapter, we will focus on the role of bacteria in the gut, but there are also viruses and fungi with less known functions. The largest microbiota is the intestinal microbiota. The gut microbes live in proximity with the intestinal wall, a boundary between the self and the foreign of the host . The fetal gut is sterile and the GM is acquired at birth and is stabilized during the first 3 years of life. The introduction of solid foods are important determinants for the establishment of an adult-like GM that is rich in butyrate-producing anaerobes . The most abundant groups of bacteria phyla are Firmicutes and Bacteroidetes The GM is dominated by anaerobic bacteria, and there is about 500–1000 species of bacteria in the GM. The gut microbiome refers to the collection of genomes and genes present in GM and it is more plastic than the human genome as it to a large extent adapts to the environment. The GM and the gut microbiome may be modulated through life by multiple factors, including diet, antibiotics, drugs, prebiotics, probiotics, and diseases ( Fig. 39.3 ). In addition, the GM is to some extent shaped by the human host genetics , and it is also influenced by sex and age . In general, it is believed that the diversity of the GM is reduced by age and that a low degree of diversity is linked to several age-dependent diseases . There are about 10 14 bacteria in the adult intestine and that is actually 3–10 times more than the number of human cells in the body . The bacteria density increases dramatically from the stomach (10 1 /g) to colon (10 12 /g). The biomass of the GM is about 1–1.5 kg, and it is mainly located in the colon. The microbiome contains more than 10 million genes and that is approximately 300 times more than the number of human genes . The GM has a high metabolic capacity, and it could be regarded as an additional human organ with a high plasticity to adapt to environmental changes. The intestinal epithelium is the main interface between the outside world and the hosts immune system and here the GM plays a role in modulating local and systemic immune response . The weaned GM are enriched in genes for polysaccharide breakdown and vitamin production . Bacterial components and molecules produced by the gut bacteria may influence several physiological and pathophysiological processes in the host. The GM is capable of fermenting undigested nutrients into SCFAs with local and systemic effects. The development of effective sequencing technologies has made it possible to study the composition of the GM, and it’s importance for both health and disease.
39.3
Experimental animal studies
39.3.1
Lessons from germ-free mouse models
GF mice are devoid of all microorganisms that make them a useful model to analyze host–microbiota interactions . Colonization experiment can demonstrate how the introduction of an intact GM or selected strains of bacteria impact on the physiology of the mouse. GF mice have immature mucosal immune systems and fewer and smaller germinal centers within the spleen, demonstrating the important role of GM in shaping systemic immunity . Sjogren et al. was first to show that the GM regulates bone mass using the GF mouse model. Absence of GM in GF mice leads to increased bone mass, compared to conventionally raised (CONV-R) mice . Both the trabecular bone mass and the cortical bone mass were increased in GF mice compared with CONV-R mice . Colonization of mice born in a GF environment with GM from mice raised in a conventional environment normalized both the trabecular and cortical bone mass ( Fig. 39.1 ). GF mice had reduced number of osteoclasts and a decreased number of osteoclasts was formed in bone marrow cultures from GF mice suggesting that the increased bone mass in the GF mice was caused by reduced bone resorption, as a result of inhibited osteoclastogenesis. The GF mice had reduced mRNA expression of osteolytic cytokines in the bone, suggesting that the decreased osteoclastogenesis was mediated by the immune system. After this study in 2012, there have been several other studies evaluating the bone mass in different GF mouse models. Similar to the first study, Li et al. observed increased bone mass but normal body growth and longitudinal bone growth in GF female C57BL/6J mice . An increased bone mass was demonstrated also in GF female C57BL/6J mice from the gnotobiotic facility at the Pasteur Institute, France . In a study in male mice on a C57BL/6 background, GF mice exhibited increased trabecular bone mass, normal bone length and decreased osteoclast size and eroded bone perimeter which is similar to what Sjogren et al. and Li et al. found using female mice on the same genetic background . Furthermore, in a study by Uchida et al. GF, mice had increased bone mineral density (BMD) and bone volume fraction (BV/TV) compared to CONV-R mice . To summarize the above studies, colonization with GM leads to an activation of the immune system resulting in increased levels of proinflammatory cytokines and activation of osteoclastic bone resorption ( Fig. 39.4 ). In contrast to the studies discussed previously, Quach et al. reported that colonization of mice, both outbred and inbred strains, did not have a major impact on bone health . Interestingly, Schwarzer et al. observed that male GF mice on a BALB/c background had a general growth defect reflected by reduced body weight gain as well as decreased longitudinal and radial bone growth compared to CONV-R mice . The reduced body growth was associated with reduced IGF-I levels and could be normalized by treatment with a specific microbial strain. Novince et al. showed that male GF mice on a C57BL/6 background had normal body growth and femur length at 12 weeks of age and bone growth was not affected in other short time studies in GF mice . Therefore the study by Schwarzer et al. suggests that the capacity of the GM to increase nutritional uptake and energy harvest and thereby increase body growth is depending on the given diet, the GM composition and possibly the genetic background of the mouse strain.
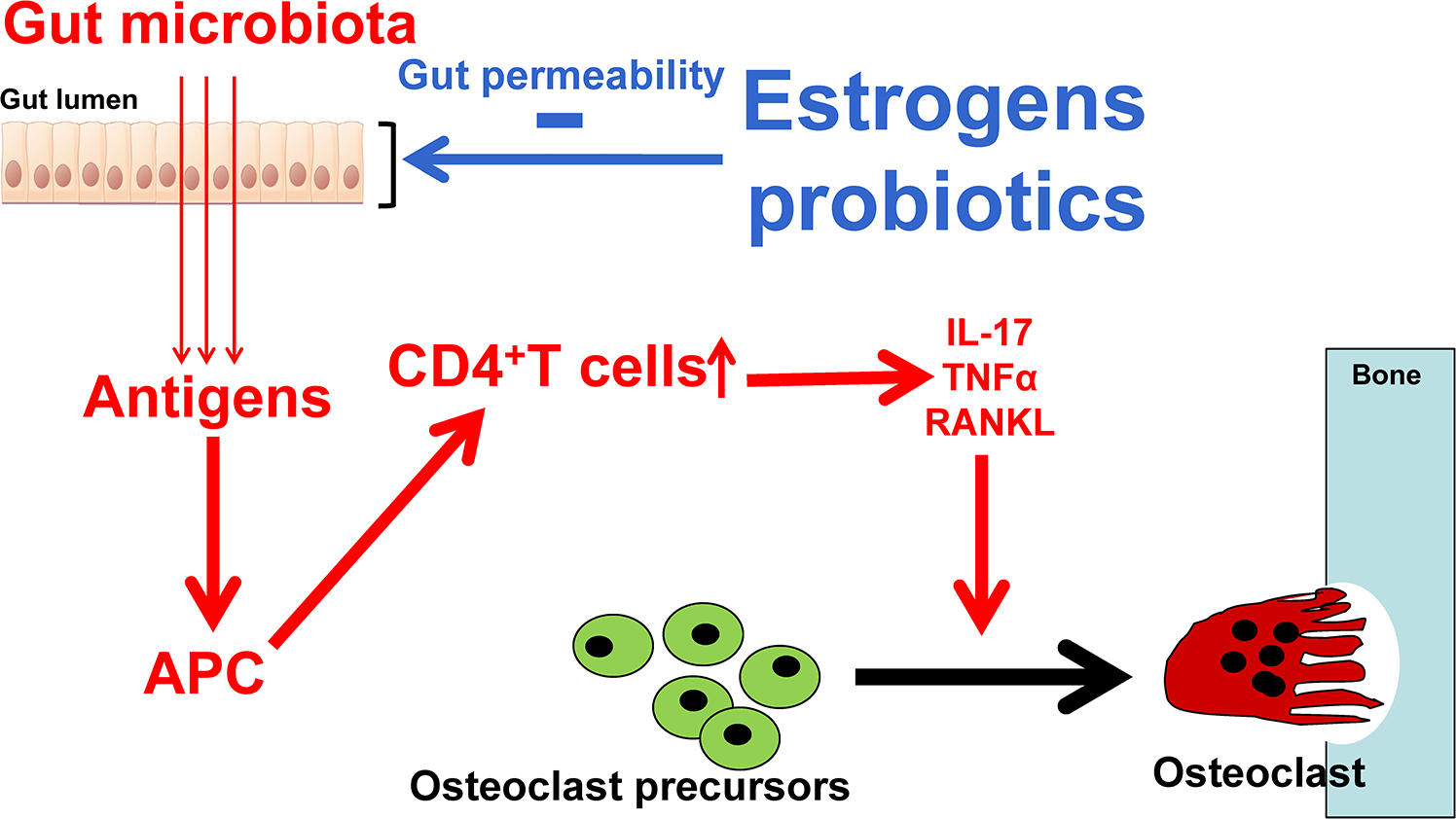
It has been suggested that the growth promoting effect by GM is important for body growth and bone dimensions under certain conditions, while the more acute immune-mediated effects of the GM on the skeleton is reflecting situations of adult bone metabolism such as postmenopausal osteoporosis and inflammation-mediated bone loss. In line with this, Yan et al. showed that the short-term effect of the GM 1 month after colonization of GF mice was a reduction of bone mass and increased bone resorption, while long-term colonization increased overall body growth and serum IGF-I, resulting in enhanced longitudinal and radial bone growth .
In conclusion, the overall immune-related effect of short term colonization of GF mice with GM results in an activation of osteoclastic bone resorption. Besides these immune-mediated effects on bone mass, the GM is involved in nutritional uptake and may, thereby, regulate overall body growth and bone sizes. All these studies using GF mice and colonization are extreme situations and it might be more physiological to look on the bone effects of treatments resulting in minor but specific changes of an already present GM.
39.3.2
Antibiotic treatment and bone
Treatment with certain broad spectrum antibiotics deplete commensal flora and can result in long-term changes in intestinal microbiota composition . In one study a sub therapeutic antibiotic treatment to mice at weaning increased bone mass after 3 weeks of exposure suggesting that the GM composition may regulate bone mass . The low dose of antibiotics in that study was associated with shifts in the composition of the GM. Furthermore, low-dose penicillin delivered from birth or from weaning caused an increased BMD in adult female mice . In another study higher doses of antibiotics were given repeatedly to young mice for short periods of time to mimic antibiotic use in children . This resulted in increased bone mass in early life that later normalized. In addition, tetracycline treatment can prevent bone loss and improve mechanical properties of bone after ovx . In contrast to the above studies a high dose of antibiotics administered to young mice for 12 weeks to disrupt the GM resulted in impaired bone strength and tissue material properties . In a study by Schepper et al., adult mice received a similarly high dose of antibiotics but bone properties were studied 4 weeks after the termination of treatment when the intestinal microbiome had repopulated the gut . These mice exhibited changed GM composition, increased intestinal permeability, and markedly decreased femoral trabecular bone volume. Adult mice with a repopulated GM after antibiotics treatment had no effect on cortical bone or tissue material properties in contrast to the earlier study of long-term treatment of antibiotics to young mice . The above studies on antibiotics highlight the complexity in the GM mediated effects on bone. Age and genetic background of the mice, the animal facility, length and type of treatment all play a role for the outcome but in general these studies demonstrate that alterations in the GM regulates bone mass, supporting an important role for the GM on bone homeostasis.
39.4
Effects of probiotics on bone mass in rodents
Probiotic is defined as “live microorganisms that, when administered in adequate amounts, confer a health benefit on the host” One important advantage of using probiotic treatment compared with the GF mouse model when evaluating the role of the GM for adult bone metabolism is that no developmental effects might confound the findings. Probiotic treatments changes composition and/or metabolic activity in an already present GM. A large number of studies have evaluated the role of probiotic treatments in different rodent bone disease models as well as in healthy gonadal intact rodents and these studies, which in general have reported beneficial effects of probiotic treatment on skeletal health, will be summarized in this section .
39.4.1
Ovariectomized rodent models
The rapid bone loss caused by the pronounced drop in estradiol levels during menopause contributes to the lower bone mass and higher risk of fractures in women compared with men . Current available approved osteoporosis medications have postmenopausal osteoporosis as a main indication. The rodent ovariectomy (ovx)-model is a well-established animal model of postmenopausal osteoporosis.
Ohlsson et al. was the first to show in a preventive study that probiotics can protect from ovx-induced bone loss in mice . The ovx-mice were treated with either a single Lactobacillus paracasei or a mix of L. paracasei and Lactobacillus plantarum. Both treatments protected the mice from ovx-induced bone loss, and this was associated with decreased expression of inflammatory cytokines and increased frequency of regulatory T cells in the bone marrow. Shortly after this study, an independent study by Britton et al. confirmed that probiotics has the capacity to protect mice from ovx-induced bone loss . These authors demonstrated that preventive treatment with Lactobacillus reuteri protected mice from ovx-induced bone loss associated with decreased osteoclastic gene expression in the bone marrow. Sex steroid deficiency increases intestinal permeability causing inflammation that can lead to bone loss . In an elegant study by Li et al. it was demonstrated that treatment with Lactobacillus rhamnosus GG or the combination of probiotic strains known as VSL3# protected from ovx-induced bone loss associated with a protection from increased intestinal permeability, suggesting that probiotics at least partly may protect from ovx-induced bone loss via a modulation of the intestinal permeability and thereby inflammation ( Fig. 39.4 ). Moreover, Lactobacillus acidophilus treatment protected mice from ovx-induced bone loss by decreasing inflammatory cytokines and increase regulatory T cells in both bone marrow and spleen . Besides treatment with several different Lactobacillus strains, both Bacillus clausii and Bifidobacterium longum , have been reported to protect rodents from ovx-induced bone loss . Collectively, these studies clearly demonstrate that probiotic treatments have the capacity to protect female rodents from ovx-induced bone loss. Currently, it has not been reported if probiotics may also protect male rodents from castration-induced bone loss.
39.4.2
Healthy gonadal intact rodents
The effect of probiotics on the skeleton has also been investigated in healthy gonadal intact female and male rodents. McCabe et al. was the first to show that probiotic treatment, using L. reuteri , increased the trabecular bone mass in femur and vertebra in healthy gonadal intact male but not female mice . The increased bone mass in male mice was associated with decreased intestinal inflammation. Collins et al. demonstrated that the positive effects of L. reuteri on bone mass in healthy gonadal intact male mice is dependent on lymphocytes . Two other studies support that treatment with certain Lactobacillus strains does not increase bone mass in healthy gonadal intact female mice . In contrast, treatment with L. rhamnosus GG was in another study shown to increase trabecular bone mass in both femur and spine in healthy gonadal intact female mice . In a subsequent study it was reported that the increased trabecular bone mass by L. rhamnosus GG treatment was associated with increased intestinal production of the SCFA butyrate. Butyrate diffuses to the bone marrow where it increases the number of regulatory T cells. This lineage stimulates the production of the osteogenic Wnt ligand Wnt10b by CD8+ T cells. Wnt10b activates Wnt signaling in osteoblastic cells, thus stimulating bone formation in healthy gonadal intact female mice . Taken together, the effect of Lactobacillus on bone mass in healthy gonadal intact mice might be strain dependent, and it is also possible that the animal facility or the genetic background of the mouse strain used might contribute to the conflicting results regarding the effects of different probiotic treatments on bone mass in healthy gonadal intact rodents.
39.4.3
Inflammation-induced bone loss
Perturbations in the GM homeostasis can be caused by pathogens, antibiotic treatment, and diet causing inflammation, tissue destruction and dysbiosis that may lead to disease development . Perturbed microbial composition has been postulated to be involved in a range of inflammatory conditions, within and outside the gut, including inflammatory bowel diseases, rheumatoid arthritis (RA), multiple sclerosis, food allergies, eczema, and asthma as well as obesity and the metabolic syndrome . Low-grade inflammation has been reported to affect physiological bone turnover and play a role in pathological skeletal conditions such as osteoporosis . Furthermore, gut-associated inflammatory and autoimmune conditions have been associated with low bone mass . Recently, the protective capacity of probiotic treatments for bone health in inflammation-induced bone loss has been evaluated in different rodent disease models. In one study, L. reuteri increased bone mass in female mice that were subjected to dorsal skin incision to induce a chronic inflammation . Treatment with L. reuteri was also shown to prevent type 1 diabetes induced bone loss in male mice by increasing Wnt10b expression . The autoimmune disease RA is associated with severe bone destructions. Treatment with Lactobacillus casei in adjuvant-induced arthritis in rats partly protected against the inflammation-induced bone loss and this protective effect was associated with decreased inflammatory cytokine levels in serum . Taken together, available evidence indicate that at least certain Lactobacillus strains have the capacity to protect rodents from inflammation-induced bone loss.
39.4.4
Probiotics modulates the effects of medications on bone health in rodents
One may hypothesize that different prescribed medications may exert their positive or negative effects on bone health via different GM dependent mechanisms and some recent studies support this notion ( Fig. 39.3 ). Glucocorticoids (GCs) are immune-modulating drugs with severe side effects, including GC-induced osteoporosis. It was recently shown in a groundbreaking study that GCs treatment in mice alters the GM composition . Importantly, depletion of the GM by broad spectrum antibiotics prevented the GC-induced bone loss, demonstrating that the GM is crucial for mediating GC-induced bone loss. Interestingly, treatment with L. reuteri prevented from GC-induced bone loss by protecting from increased intestinal permeability and increased apoptosis of osteoblasts and osteocytes. Severe bone loss is associated with the antiretroviral agent tenofovir disoproxil fumarate (TDF) which has been widely used as a first-line treatment of human immunodeficiency virus (HIV) and hepatitis B virus (HBV) infections worldwide TDF plays an antiretroviral role by inhibiting adenine analog reverse transcription and improves the survival of patients with HIV or HBV . However, TDF treatment is associated with substantially increased bone resorption and bone loss that is larger compared with other anti-HIV agents . Recently, it was shown in a mouse model that L. rhamnosus GG prevented TDF-induced bone loss, and this protection was associated with increased intestinal barrier integrity, expanded regulatory T cells, decreased Th17 cells, and downregulated osteoclastogenesis-related cytokines in the bone marrow, spleen, and gut in the TDF treated mice . In a study by Schepper et al., they evaluated the postantibiotic effects on bone and found that mice that were treated with L. reuteri or a mucus supplement were protected from postantibiotic bone loss by preventing from increased intestinal permeability . These three recent important studies demonstrate that probiotic treatment has the capacity to prevent medication-induced bone loss involving the GM and future clinical studies should determine if GCs and/or TDF regulate the human GM composition and if the deleterious effects of GCs and TDF on bone health might be avoided by specific probiotic treatments.
Similar as recently described for the first-line medication for treatment of type 2 diabetes metformin, the GM might also contribute to the beneficial bone effects of the different available osteoporosis treatments . Metformin was reported to alter the gut microbiome of individuals with treatment-naïve type 2 diabetes, contributing to the therapeutic effect of the drug. It was first demonstrated in descriptive studies that metformin altered the GM composition in humans and subsequent functional studies, using fecal transplantation from metformin-treated donors to GF mice, revealed that metformin treatment of the donors improved the glucose tolerance in the recipient mice . Intermittent treatments with PTH analogs are approved for osteoporosis treatment, and in a recent report, it was demonstrated that the GM is permissive for the bone anabolic effect of intermittent PTH treatment in mice . In that study, it was shown that intermittent PTH does not increase bone mass in GF mice or mice with the depletion of GM using broad spectrum antibiotics. Subsequent mechanistic studies revealed that the GM produces permissive butyrate acting on GPR43 to enable intermittent PTH to increase Treg cells and thereby bone formation . Thus there is now data from animal studies that at least some of both the negative and positive effects of different medications on bone health are mediated via or dependent on the GM. Future human translational studies are clearly warranted to determine if the effect of different medications on bone health is dependent or mediated via the GM also in human.
39.5
Effects of prebiotics on bone in rodents
Prebiotics are defined as a substrate that is selectively utilized by host microorganisms conferring a health benefit to the host . In contrast to probiotics, prebiotics are nonviable and serve as substrates for beneficial microorganisms harbored by the host. Most prebiotics are carbohydrate based, known to resist digestion in the human small intestine and reach the colon where they are fermented by the GM, but other substances such as polyphenols and polyunsaturated fatty acids might also exert prebiotic effects. Two of the most well-studied prebiotics are fructans [fructooligosaccharides (FOS) and inulin] and galactans [galactooligosaccharides (GOS)] that act through the enrichment of Lactobacillus and/or Bifidobacterium among others . The end products from bacterial breakdown of polysaccharides are SCFAs . Acetate and lactate are the main metabolic end products of Bifidobacteria and lactic acid bacteria and are utilized by other microorganisms to produce, for example, propionate and butyrate. The proposed underlying mechanisms for the beneficial effects of prebiotics include increased solubility and absorption of minerals, improved gut barrier function, and antiinflammatory effects .
Calcium is the key bone mineral and an adequate supply of calcium is essential for bone health. Growing evidence exists for prebiotic effects on calcium metabolism and bone health in both animals and humans . The combination of prebiotics with other bioactive ingredients such as probiotics or polyphenolic compounds, so called synbiotics, is another research area with regard to bone health. Studies in rodents and humans treated with different types of prebiotics and synbiotics and effects on calcium absorption and bone have recently been reviewed . Positive effects have been reported on calcium absorption and bone parameters in rodents under various conditions such as puberty, sex-hormone deficiency, and old age. Few studies have evaluated the role of the GM in the prebiotic-bone interactions. In rats, GOS supplementation altered the GM composition, with an increase in the relative proportion of Bifidobacteria , and increased the bone mass . In that study, greater cecal wall and content weight, and decreased luminal pH were correlated with improved calcium absorption and BMD. Resistant starch have also attenuated ovx-induced bone loss and increased the abundance of Bifidobacterium species in faeces in mice . Collectively these studies demonstrate that prebiotic treatment have the capacity to regulate bone mass in several different rodent animal models. Recent elegant studies by the Gordon research group have demonstrated that microbiota-directed foods have the capacity to regulate bone growth in gnotobiotic mice, piglets, and in undernourished children . They also characterized the mechanism by which sialylated milk oligosaccharides impact bone growth in a gnotobiotic mouse model of infant undernutrition . They hypothesized that identifying components of breast milk that influence postnatal development through their effects on the GM and immune system could provide new therapeutic approaches for childhood undernutrition, including treatment-refractory linear growth faltering. They demonstrated that gnotobiotic mice, colonized with a stunted infant’s GM, exhibited decreased bone resorption when consuming diets supplemented with a purified bovine oligosaccharide mixture dominated by sialylated structures found in human breast milk. Supplementation decreased osteoclastogenesis while sparing osteoblast activity; the GM, intestinal cell populations, and immune mediators contribute to these responses. Based on these findings, the authors proposed that the influence of certain milk oligosaccharides on the GM–bone axis may have diagnostic and therapeutic implications in undernourished children.
39.6
Mechanisms for gut microbiota to affect adult bone homeostasis
39.6.1
Immune cells of intestinal origin regulate bone homeostasis and is modulated by the gut microbiota
The gut microbiome harbors hundreds of bacterial genera that reside in the luminal stream or adhere to the gut mucosa. The intestinal microbiome affords the host-enhanced energy extraction from foodstuff, regulatory effects on epithelial growth, the exclusion of colonization by pathogens, and many other benefits . In addition, the gut microbiome is essential for efficient immune system maturation, as well as protecting the intestinal epithelium against exogenous insults. Gut microbes modulate intestinal and systemic immune responses . For instance, gut-resident microbes have a robust influence on the emergence and/or maintenance of CD4+ T cell subsets. Examples include the effects of segmented filamentous bacteria (SFB) on the expansion of Th17 cells and the impact of Bacteroides fragilis in Th1 cells and regulatory T cells (Treg) differentiation . Indeed, abnormalities in gut microbial diversity (dysbiosis) have been suggested to be sufficient to aggravate intestinal pathologies related to the immune system such as in inflammatory bowel disease . However, a more intriguing paradigm is emerging evidence that the commensal microbes also influence immune responses distant from mucosal surfaces, including, but not limited to, the CNS, joints, bone, and lungs . The capacity of the gut microbiome to regulate distant organs has led to common use of terms such as the gut–bone axis and the gut–brain axis.
Gut permeability, the passage of bacteria, bacterial constituents, and metabolites produced by bacteria is an essential component of the gut–distant organs axes. The intestinal wall is in intimate contact with the gut luminal microbiota and constantly samples and responds to the antigenic load within the intestinal lumen . The intestine controls molecular trafficking between the lumen and the epithelial submucosa through paracellular space that under physiological conditions exclude the passage of molecules with a radius >15 Å . Increased permeability allows larger molecules and potential antigenic load to enter the gut wall and initiate intestinal inflammatory responses . Thus maintaining a tight selective physiological barrier between the gut lumen and submucosa is essential for health. Maintaining tight gut permeability is also critical in the context of bone health because osteoclastogenic cytokines are produced by immune cells that reside in intestinal subepithelial compartments of the intestine, and any change in gut permeability elevate local and systemic levels of osteoclastogenic cytokines and influence bone homeostasis. Gut permeability can be directly altered by dysbiosis, diseases of the GI tract such as inflammatory bowel disease, aging, and nutritional abnormalities. Sex-steroid deficiency increases gut permeability by downregulating the expression of a number of the gap junction proteins of the claudin and JAM families , leading to increased antigenic load entering the epithelial submucosa, thereby initiating the production of osteoclastogenic cytokines . Increased gut permeability is indeed a critical mechanism by which estrogen deficiency induces bone loss . The link between estrogen deficiency, increased gut permeability, and bone loss explains why mice raised in GF conditions are protected against the bone loss induced by estrogen deficiency . This mechanism also explains why treatment with probiotics capable of decreasing gut permeability such as L. rhamnosus GG prevents ovx-induced bone loss ( Fig. 39.4 ).
Interestingly, little information is available on whether increased gut permeability regulate distant organs through the migration from the gut to the bone marrow of bacteria, cytokines, immune cells, or a combination of these factors. Bacterial translocation, the passage of viable bacteria across the intestinal wall into the systemic circulation, is a recognized phenomenon, although very rare in healthy individuals . Bacterial translocation is unlikely to be a frequent contributor because bacteria are efficiently killed by the immune system once they enter the systemic circulation. Translocation of GM-derived ligands such as endotoxin and flagellin into the circulation occurs physiologically , and this may be sufficient to activate immune cells in distant organs, including the bone marrow. Cytokines are unlikely to reach distant organs in significant concentrations as these molecules, contrary to hormones, do not travel efficiently in the blood stream. On the other hand, activated T cells and B cells constantly migrate from the gut to peripheral lymphoid organs or inflamed tissues driven by chemokine gradients. Therefore migration of lymphocytes that differentiate and expand in the gut in response to the microbiome and then travel to the bone marrow is likely to be an important mechanism by which the microbiome regulates bone resorption and bone formation. A central paradigm is offered by Th17 cells. This lineage plays a pivotal role in the bone loss resulting from estrogen deficiency but also from pathologic conditions such as psoriasis, RA, periodontal disease, and inflammatory bowel disease . In these inflammatory states, Th17 cells potently induce osteoclastogenesis by secreting IL-17A, RANKL, TNF, IL-1, and IL-6, along with low levels of IFNγ . Th17 cells are mostly produced in the intestinal lamina propria. From there, Th17 cells migrate to the Peyer’s patches. Th17 cells express sphingosine 1 phosphate (S1P) receptor 1 (SIP1R1), and egress from Peyer’s patches when attracted to circulating S1P . Once in the circulation, Th17 cells home to distant peripheral sites of inflammation . For example, studies in autoimmune disorders have shown that SFB-induced Th17 cells migrate from the gut to the lungs, kidneys, and joints where they cause tissue injuries . The homing of Th17 cells to the inflamed peripheral areas is driven by the combined action of the chemokine CCR6 on Th17 cells and the chemokine ligand CCL20, which is expressed by cells at sites of inflammation . CCL20 is strongly induced by inflammatory cytokines including TNF . These reports suggest that estrogen deficiency, which increases bone marrow levels of TNF, may trigger the recruitment of intestinal Th17 cells to the bone marrow.
Bone loss is a frequent but not universal complication of hyperparathyroidism. Studies in mice models of primary and secondary hyperparathyroidism show that PTH only caused bone loss in mice whose microbiota was enriched by the Th17 cell-inducing taxa, SFB. In the presence of SFB in the GM, PTH induces the expansion of intestinal Th17 cells, which migrate to the bone marrow attracted by a chemokine gradient, where they stimulate bone resorption and induce bone loss .
The development of Th17 cells in the gut is heavily influenced by the presence of SFB , which are spore-forming, Gram-positive commensal bacteria that potently induce differentiation of Th17 cells . Although in healthy mice SFB is the only known taxa of the GM that expands Th17 cells, it is noteworthy that several pathogens that cause intestinal infection, such as Candida albicans and Citrobacter rodentium , are also known to expand gut Th17 cells in mice. In humans several saprophytes are known to induce Th17 cell generation in the gut. Therefore alterations in the composition of the microbiome can alter the generation of Th17 cells and the size of the pool of Th17 cell in the gut. The presence of a reservoir of Th17 cell in the intestine and the capacity of inflamed tissues o recruit Th17 cells provide an example of how the microbiota regulate distant organs via immune cells.
39.6.2
Gut-derived bacterial metabolites regulate bone homeostasis
The GM produces metabolites that account for anatomically distant biological effects. These metabolites may diffuse to distant organs such as the bone marrow to regulate bone and hematopoietic cells or can act in the intestine modulating local immune cells, which in turn migrate to distant organs.
Indole derivatives were among the first bacterial metabolites to be described to influence intestinal immunity . In addition, trimethylamine N-oxide (TMAO), an amine oxide present in food or generated within the human intestine by the microbiota from choline and carnitine, was found to negatively affect the vascular system and kidneys. 4-Ethylphenol sulfate, a metabolite produced by intestinal saprophytes, was shown to regulate human behavior and has been implicated in autism . IGF-1, produced predominantly in the liver in response to food intake and regulated by microbes and microbial products, was the first metabolite identified as a linker in the gut–bone axis . Another bone-regulating molecule is hydrogen sulfide (H 2 S), a gasotransmitter generated by gastrointestinal cells and by bacteria residing within the gut . The microbiota accounts for a substantial portion of the overall blood levels of H 2 S, as GF mice have low serum and gastrointestinal tissues levels of H 2 S . In turn, H 2 S can modify the composition of the microbiota . H 2 S has been implicated in inflammatory bowel disease and other GI pathologic conditions. Importantly, H 2 S stimulates bone formation and postnatal skeletal development . In addition, the H 2 S-donating compound GYY4137 increases bone formation by activating Wnt signaling, via increased Wnt10b production and prevents the loss of trabecular bone induced by ovx . However, the family of metabolites produced by intestinal bacteria that has received the greatest attention for their capacity to diffuse to distant organs and induce potent regulatory effects is SCFAs. These metabolites are derived from bacterial fermentation of complex nondigestible carbohydrates present in the diet . The main SCFAs are butyrate, propionate, and acetate. The highest concentration of SCFAs is found in the cecum.
SCFAs bind to four receptors, the free fatty acid receptors GPR43 and GPR41 (also known as FFAR2 and FFAR3) , the niacin/butyrate receptor GPR109a (also known as HCA2) , and the olfactory receptor Olfr78 . These receptors show distinct binding affinities for specific types of SCFAs, as well as different patterns of expression. In addition, SCFAs inhibit histone deacetylases (HDACs) and stimulate histone acetyltransferase via a receptor-independent mechanism .
SCFAs regulate gut permeability by inducing the expression of the tight junction proteins such as claudin-1 . Moreover, the interaction of SCFAs with intestinal epithelial cells or immune cells of the gut mucosa leads to essential antiinflammatory effects. Among them is the enhanced production of antimicrobial peptides by intestinal macrophages and the activation of the NLRP3 inflammasome .
In addition, SCFAs are critical regulators of the immune system . They act by modifying gene expression profiles , cell chemotaxis , differentiation , proliferation , and apoptosis . Among the immune cells regulated by SCFAs are B cells , macrophages , and neutrophils . However, the most relevant effect of SCFAs is their capacity to induce the differentiation and proliferation of Tregs in the intestine and in distant organs . Attesting to the relevance of SCFAs for the maintenance of the normal Treg pool is the observation that GF mice have fewer Tregs than CONV-R mice, and the fact that nutritional supplementation with SCFAs alone is sufficient to increase Treg numbers in the intestine of GF mice . Butyrate and propionate are the most potent inducers of Tregs . SCFAs support the development of peripheral Tregs in the intestine through multiple mechanisms. A key effect of SCFAs is to increase the differentiation of naïve CD4 + T cells into Tregs via intrinsic epigenetic upregulation of the Foxp3 gene in T cells. This effect, which is surface receptor-independent , is mediated by increased histone H3 acetylation of the promoter for Cns3 and Cns1 gene loci . Another effect of SCFAs is to increase the proliferation of mature Tregs . This effect was reported to be GPR43-dependent in one study and GPR43-independent in another study . Additional effects of SCFAs, especially butyrate, on Treg maturation are mediated by dendritic cells (DCs) , which express both GPR109A and GPR43 . Since GPR43 is expressed at high levels by myeloid cells , it is likely that GPR43 mediates the effects of SCFAs on DCs. Butyrate and propionate prevent the development of DCs via inhibition of histone acetyltransferase and by upregulating antiinflammatory genes . This effect results in an increased capacity of DCs to support Treg differentiation, inhibiting the generation of effector T cells .
Importantly, certain probiotics such as L. rhamnosus GG and intermittent PTH (iPTH) treatment induce bone anabolism either by increasing the production of butyrate or making use of permissive levels of butyrate produced by the GM . The functional link between butyrate and stimulation of bone formation is the capacity of butyrate to expand bone marrow Tregs ( Fig. 39.5 ). In fact, if the expansion of Tregs is prevented by the injection of antiCD25 antibody or other manipulations, butyrate, L. rhamnosus GG , and PTH are all unable to induce bone formation . Tregs are critical because they stimulate the production of the osteogenic Wnt ligand Wnt10b by CD8+ T cells. Studies have shown that Wnt10b produced by CD8+ T cells is a critical inducer of bone formation in response to PTH and L. rhamnosus GG . Wnt10b is a key activator of Wnt signaling in stromal cells and osteoblasts. The activation of Wnt signaling leads to increased osteoblast proliferation , differentiation , and survival , and altered production of OPG . In humans, Wnt10b is a predictor of bone mass , while in mice Wnt10b is essential for bone mass acquisition at baseline conditions , and its deficiency results in age-dependent bone loss . The function of Wnt10b as an endogenous Wnt ligand operating in bone is further supported by the observation that heterozygous Wnt10b +/− mice exhibit a significant reduction of trabecular bone . Moreover, the probiotic L. reuteri prevents diabetes-induced bone loss by upregulating Wnt10b .
