Biology of Personalized Cancer Medicine
Raju Kucherlapati
It is well established that cancer is a genetic disease. At the genetic level cancer cells are different from their precursor cells. It is understood that a series of genetic changes are necessary for a normal cell to begin the process of transformation, eventually leading to cancer. Cancers can be generally classified into sporadic cancers and those that result from a genetic predisposition. Some individuals in the population inherit specific mutations in particular genes that predispose them to certain types of cancers. Although these individuals are born with a mutation in a predisposition gene, they do not develop tumors until later in life; and it is now well understood that, besides the inherited predisposition gene mutation, additional genetic changes are required for the cells in these individuals to become tumors. In sporadic cases a randomly acquired somatic mutation or another type of genetic change in a gene that is critical for the normal regulation of growth in the appropriate cell type might initiate a series of events that eventually leads to tumor formation. In addition to genetic mutations, changes in copy number of individual genes or subsets of genes; chromosomal aberrations including translocations, insertions, deletions and inversions; and changes in expression patterns of genes as well as epigenetic changes also play critical roles in tumor susceptibility, tumor initiation, and progression. Understanding all of the important genetic and genomic changes in each cancer type will help in accurate diagnosis and prognosis and increase the ability to stratify the patient populations to help assess the most optimal treatments for each patient. The use of such genetic and genomic information to determine treatment decisions is referred to as personalized medicine. Knowledge about the genetic and genomic changes that accompany cellular transformation and the events that are critical for initiation and maintenance of the cancerous state is increasing at a rapid pace. Because most cancers are clonal in origin and because it is possible to obtain an adequate amount of tumor material from tumor biopsies or resections, it is now possible to examine and document the genetic and genomic changes in tumor cells very accurately. As a result, understanding of the genetic and genomic changes in cancer is significantly greater than many other human diseases. This knowledge allows implementation of the principles of personalized medicine into clinical management of cancer patients. This chapter will consider examples of how cancer genetics and genomics are affecting the ability to manage cancer patients.
CANCER PREDISPOSITION
There is a large body of evidence that indicates that certain families have a higher incidence of a particular cancer. Epidemiological studies reveal that family members descendant from individuals who developed cancer, especially at a younger age, have a higher risk of developing cancer. This was followed by studies of families where the predisposition to develop cancer was found to be inherited. Another line of evidence that reveals the genetic basis for cancer came from studies of twins. Monozygotic twins have the same genetic composition, while dizygotic twins have a 50% probability of sharing an identical copy of any gene. The fact that there is a higher concordance of cancer incidence in monozygotic twins but not in dizygotic twins provides additional critical evidence for the genetic basis of cancer.1 Studies during the past half century have established not only the familial predisposition of cancer but also to identified several genes that are involved in cancer predisposition. That knowledge, in turn, helps to explain the genetic processes and mechanisms that lead to cancer and to develop tests to identify individuals at risk for certain types of cancers.
There are several examples of gene mutations that are responsible for cancer predisposition. Cancers that show a familial predisposition include childhood retinoblastoma, colorectal cancer, early onset breast cancer, and several types of renal cancer, among many others. One cancer type where there is a large amount of information about familial predisposition is colorectal cancer (CRC).2 CRC is one of the most common cancers, and it is estimated that as many 875,000 new cases of CRC are diagnosed every year in the
world. The greater accessibility of the colon and rectum to detect or follow the cancer as well as the relative ease of obtaining biopsies facilitate study of this cancer.
world. The greater accessibility of the colon and rectum to detect or follow the cancer as well as the relative ease of obtaining biopsies facilitate study of this cancer.
CRC can be classified into familial cases and sporadic cases. Various estimates of the relative proportions of these two categories of CRC have been made and in some estimates the familial cases represent 10% of all CRCs, while other estimates place this number to be 25% or more.3 Several genes that are involved in familial cases have been identified and are discussed in the sections that follow.
FAMILIAL ADENOMATOUS POLYPOSIS
Individuals with familiar adenomatous polyposis (FAP) are born normally and develop hundred to thousands of benign colonic polyps during their early adulthood. Unless these tumors are detected and treated (usually by surgery), one or more of them may develop into adenocarcinomas that can metastasize. Family studies revealed that this predisposition was inherited in an autosomal dominant fashion, and individuals who have inherited the susceptibility allele almost always exhibit the phenotype (near 100% penetrance). Linkage analysis revealed that the gene for FAP is located on human chromosome 5. Positional cloning has enabled the cloning of the gene that was designated as adenomatous polyposis coli (APC).4,5 APC is a classic tumor suppressor gene, and inactivation or modification of both copies of the gene is necessary for the initiation of CRC development.
APC plays an important role not only in the relatively rare cases of FAP but also in a majority of sporadic CRC. Most of the sporadic colorectal tumors are also the result of the inactivation of both copies of the APC gene, but unlike FAP, where one copy is already mutated in the germline, in sporadic cases both copies are sequentially mutated in somatic colonic epithelial cells.6 This observation explains the earlier onset of tumors and the abundance of tumors in FAP patients.
Since FAP is inherited in an autosomal dominant fashion and since individuals with FAP are born with a mutation in the APC gene, when an individual with FAP is identified it is recognized that all of the immediate family members are at risk to carry the mutant allele. The siblings of the affected individual are at 50% risk, and other relatives would also be at risk depending on the nature of familial relationship. Because the diagnosis of FAP is unambiguous, genetic testing is not always conducted. It is most desirable to sequence the germline DNA of the affected individual to identify the mutation, inform the relatives of their risk, and recommend the testing for the specific mutation as appropriate.
LYNCH SYNDROME
Another predisposition to CRC is Lynch syndrome (LS), named after Henry Lynch who first described this syndrome.7 LS is also inherited as an autosomal dominant disorder, and individuals who inherit a disease allele develop CRC at an earlier age than sporadic CRC but later than FAP patients. The manifestation of colorectal neoplasms in individuals with this syndrome is less severe than in FAP patients, with the development of a few tumors later in life.
It was noted that the LS tumors have a unique feature in that they show genetic instability as revealed by expansion or contraction of the length of microsatellites.8,9,10 Based on these observations these tumors are classified as microsatellite instable (MSI+). This knowledge of microsatellite instability plays an important role in discovering the genes important for LS. The first gene that was responsible for a subset of LS cases was found to be a human gene that has homology to a bacterial gene that is necessary for repairing single nucleotide mismatches and small insertions or deletions that result from errors in DNA replication.11,12 This gene was designated Mut S homolog 2 (MSH2). MSH2 encodes a protein that is required for recognition and repair of DNA mismatches. It was later discovered that mutations in other genes that encode members of the mismatch repair complex also cause LS. In addition to MSH2 the genes that encode other members of this complex that are now known to be involved are Mut L homolog 1 (MLH1), Mut S homolog 6 (MSH6), postmeiotic segregation 2 (PMS2), and Mut L homolog 3 (MLH3). Mutations in all of these genes are now implicated in colon cancer susceptibility.11,13,14,15,16,17,18
Relatives of LS patients are also at increased risk to carry the mutant gene and, therefore, for CRC. As is the case for FAP it would be desirable to establish the specific mutation that causes LS and test the immediate relatives to establish or rule out the presence of the specific mutation in their germline. The presence or absence of mismatch repair proteins, especially MLH1, can also be detected by immunological methods, and individuals whose tumors do not have a detectable level of MLH1 are excellent candidates for testing of their germline; if a germline mutation in the gene is detected, informing that patient’s immediate relatives and testing them for the specific mutation may be warranted.
The precise incidence of LS in the population has been difficult to assess. Because individuals with LS develop fewer tumors and later in life than those with FAP, they are more difficult to distinguish from sporadic cases, and, therefore, testing for LS has not become routine. According
to some studies the incidence of LS among patients with colorectal neoplasms is as high as 3%.19 These results suggest that examination of all colorectal tumors for its MSI status and testing for mismatch repair gene mutations in individuals whose tumors are MSI+ may help identify individual at risk with a greater efficiency.20,21
to some studies the incidence of LS among patients with colorectal neoplasms is as high as 3%.19 These results suggest that examination of all colorectal tumors for its MSI status and testing for mismatch repair gene mutations in individuals whose tumors are MSI+ may help identify individual at risk with a greater efficiency.20,21
OTHER POLYPOSIS SYNDROMES
There are other syndromes that are relatively rare that predispose individuals to CRC risk. These include Peutz-Jeghers syndrome, juvenile polyposis, and Cowden’s disease. Genes that are involved in these syndromes have been identified and include LKB1, SMAD4, BMPR1A, and PTEN. Although all of the syndromes mentioned above are inherited in a dominant fashion, mutations in MYH, a homolog of an excision repair gene in Escherichia coli, cause a tumor predisposition but in a recessive fashion (de la Chapelle22 gives a detailed review).
ASSOCIATION STUDIES
Most of the genetic mutations in genes described above result in CRC with high penetrance. This raises the question if there are other genes where mutations or variants cause a predisposition to CRC but with lower penetrance. Studies of sibling pairs that are concordant or discordant for CRC as well as association studies have identified regions of the genome or single nucleotide polymorphisms (SNP) that may be important in CRC susceptibility. One such polymorphism is that located on human chromosome 8. In an initial study Zanke et al.23 examined a large cohort of individuals who had large bowel cancer and an equal number of controls for associations with genes or variants in the genome. In this study they identified SNPs at 8q24, which shows significant association with susceptibility to colon cancer. This region was also shown to be responsible for susceptibility to several other cancers.24,25 Additional follow-up studies confirmed and extended these observations, implicating other regions of the genome in susceptibility to colon cancer among “sporadic” cancer cases.26 Like several other SNP variants that have been shown to be associated with complex diseases, the SNPs at 8q24 also lie in a region that is not known to harbor any genes. However, Pomerantz et al.27 were able to show that this variant is functionally important in regulating the expression of the cellular oncogene c-myc that is located a few hundred kilobases away from the variant. This group also showed that variants at 8q24 that are known to be involved in other solid tumors also act through their action on the myc oncogene.28 These results suggest that it might be possible to identify individuals within the general population that show susceptibility to several different solid tumors. Identification of such susceptible individuals may, in turn, help in more careful monitoring or other interventions, which may lead to prevention of the cancers.
BREAST CANCER
Susceptibility to early onset breast cancer has been extensively studied. It was recognized that certain families have a high incidence of breast and ovarian cancers. Careful examination of these families revealed that the predisposition to these cancers is inherited in an autosomal dominant fashion. Genetic linkage analysis revealed that, at least in some families, this trait is linked to markers on human chromosome 17.29 When positional cloning approaches became available, it was determined that mutations in a gene on this chromosome were found to be responsible for this predisposition.30,31 That gene was designated BRCA1 (BReast CAncer-1). In other families a second gene, BRCA2, located on chromosome 13, was found to be involved in the cancer predisposition. Women who inherit a mutation either in BRCA1 or BRCA2 are at high risk for development of breast or ovarian cancer. Mutations in these genes can be inherited or they could result from new mutational events. If an individual with mutations in either BRCA1 or BRCA2 has been identified, it would be important to assess if other members in their family are at risk. If inherited, since the mutations are dominant acting, each of the immediate relatives (siblings) would have a 50% risk of carrying the same mutation and therefore would also be at high risk for developing cancer. Individuals with known pathogenic mutations may elect prophylactic mastectomy and oophorectomy or careful surveillance to detect tumors at their earliest stage.
Testing individuals at high risk for breast cancer or colon cancer is a common practice. If an individual tests positive for a pathogenic mutation, it is prudent to test for the presence of the same mutation in that individual’s immediate relatives and manage them based on the results.
It is estimated that only a fraction of women that carry a pathogenic mutation in BRCA1 or BRCA2 are detected. Detection of these mutations well in advance of the time at which they would develop their first breast or ovarian tumor would have significant positive implications for their health. A relatively simple way of identifying individuals at risk is through the use of family history. Several family history tools are available, and one that was developed by the surgeon general of the United States is easily accessible and free of charge on the U.S. Department of Health and Human Services Web site (search for “My Family Health Portrait Tool”). Algorithms that can assess relative
risk have been developed, and depending on these risk predictions, appropriate individuals may be recommended to undergo genetic testing.
risk have been developed, and depending on these risk predictions, appropriate individuals may be recommended to undergo genetic testing.
EARLY DETECTION
It is well established that the long-term survival of patients, whose tumors were diagnosed at early stages, are significantly greater than those whose tumors are detected at a later stage. For example, the long-term survival of patients whose colonic tumors were detected at stage I is 95%, while it is only 5% when the tumors are detected at stage IV.
There are different methods for early detection of cancers. For colon cancer detection, colonoscopies are recommended for all individuals over the age 50. Palpation of the prostate is a routine procedure during annual medical examinations. Mammograms are useful in detecting at least a significant portion of breast tumors. Some of these methods are expensive and patient compliance is not adequately high. Alternative strategies for detection of cancer at early stages are in development.
One approach to identify such markers was used by Faca et al.32 In this study, a murine model for pancreatic cancer was used as a starting point. These mice reliably develop pancreatic tumors during specific periods of their life. Plasma samples from these mice at different stages of tumor development were sampled and analyzed for their protein composition using proteomic approaches. Several proteins that were found to be overexpressed during early stages of cancer were examined in the blood from 30 newly diagnosed patients with pancreatic cancer and an appropriate set of 30 controls. This approach enabled them to identify a panel of five proteins that was able to discriminate pancreatic cancer cases from matched controls in blood specimens obtained as much as 12 months prior to the diagnosis of pancreatic cancer. Similar approaches enabled identification of protein markers that are important for ovarian and colon cancers.33,34
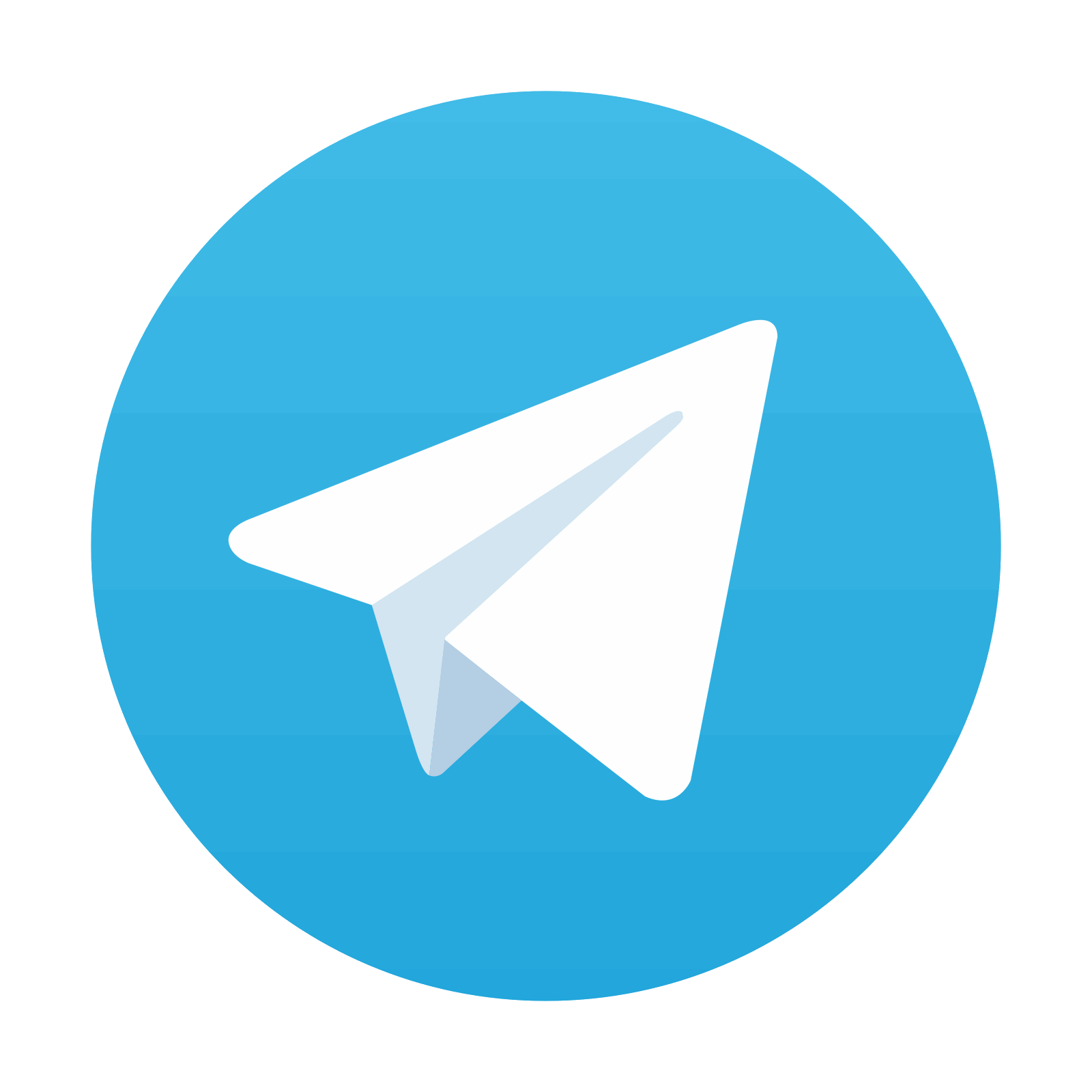
Stay updated, free articles. Join our Telegram channel

Full access? Get Clinical Tree
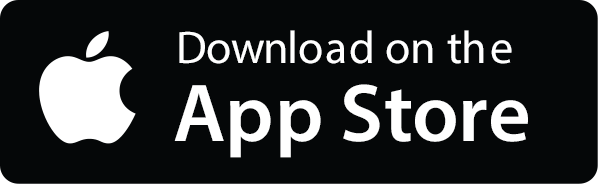
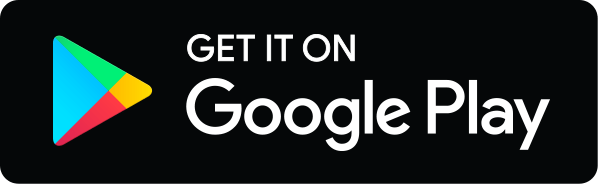