Anticoagulant and Procoagulant Proteins in the Animal Kingdom
Angelina Wei Ling Tan
Shiyang Kwong
R. Manjunatha Kini
Blood circulation has a crucial role in the survival of vertebrate animals, including man. It transports oxygen and nutrients to every tissue and organ and carries waste products and carbon dioxide to be removed via the liver, kidneys, and lungs. It also has a vital role in defense against a wide range of pathogens by recruiting leukocytes and other immune cells to the appropriate site in case of a pathogen attack. Thus, any problem with the hemostatic system can lead to death or severe debility, and therefore, it is normally tightly regulated by a complex system of proteins and cells that prevent blood loss from injuries or major blockages by pathologic thrombi and emboli.
Various animals have developed mechanisms to target the hemostatic system and exploit this vulnerability for their own ends. In some species (e.g., snakes), such mechanisms are used to immobilize and kill the prey/victim, and these are also used as defensive strategies against attacks by larger animals. In others (e.g., leeches, or insects such as mosquitoes and ticks), they are used to provide a continuous supply of blood as food. In all these animals, the mechanisms have evolved to perfection over millions of years, in parallel with the development of their hosts, to allow their survival. Since many of the components present in venom and saliva target critical hemostatic pathways, they have been of great interest in basic research into the mechanisms of these pathways, as well as in potential therapeutic applications to modify disease. Over the last three to four decades, due to the efforts of scientists from various backgrounds including biology, protein chemistry, molecular biology, pharmacology, hematology, and structural biology, significant progress has been made in understanding the structure-function relationships, as well as the mechanism of action of a number of exogenous factors from various animals, that affect blood coagulation. These exogenous factors have contributed significantly to the development of research tools as well as providing new therapeutic agents. In this chapter, we describe anticoagulant and procoagulant proteins that have been reported from the animal kingdom. Their mode of inhibition or activation of the blood coagulation cascade together with their structure-function relationships is also discussed.
ANTICOAGULANTS
Anticoagulants may inhibit the blood coagulation cascade through either direct or indirect mechanisms. In nature, animals make use of anticoagulants to their advantage, to kill their prey or to feed off their hosts. As these anticoagulants evolved independently and for different purposes, they differ greatly in function and structure.
Anticoagulants from Snake Venoms
Snakes feed on a variety of animals, including snails, fishes, frogs, toads, lizards, chickens, mice, rats, and even other snakes. As they lack the ability to tear apart the flesh of their prey, they resort to swallowing them whole. Hence, the venoms of snakes have evolved as a complex mixture of pharmacologically active proteins and peptides that have the ability to incapacitate, immobilize, and even digest their prey. These molecules include neurotoxins, cardiotoxins, hemotoxins, and cytotoxins, which target crucial physiologic systems of the prey as well as a number of hydrolytic enzymes including phospholipases, proteinases, and nucleotidases. Hence, exposure to these toxins during a snake bite often leads to symptoms ranging from tissue damage and paralysis to hypotension and hemolysis. Over the years, numerous toxins that contain anticoagulant properties have been isolated and characterized. These proteins and glycoproteins have molecular masses ranging from 6 to 90 kDa and inhibit blood coagulation through a variety of mechanisms. They can be classified under enzymatic or nonenzymatic anticoagulant proteins. As not all of the mechanisms of these anticoagulant proteins are entirely well understood, further research of these proteins is essential to delineate their mechanism and structure-function relationships.
Anticoagulant Enzymes
Phospholipases A2
Phospholipases A2 (PLA2) enzymes are esterolytic enzymes that specifically hydrolyze the sn-2 acyl bond of phospholipids, releasing lysophospholipids and fatty acids. Snake venoms are a rich source of these PLA2 enzymes, and hundreds of them have been previously purified and characterized.1, 2 Approximately 13 kDa in size, they usually consist of 116 to 124 amino acid residues and have 6 to 7 disulphide bonds. Typically, the structure of PLA2 enzymes comprises a core of three α-helices, a calcium-binding loop, and a β-wing composed of antiparallel β-sheets (FIGURE 113.1). Although they share this common scaffold with nontoxic mammalian enzymes, most snake venom PLA2 enzymes are toxic and induce diverse pharmacologic effects, including cardiotoxic, myotoxic, neurotoxic, hemolytic, convulsive, anticoagulant, antiplatelet, and edema-inducing and tissue-damaging effects.3, 4 Based on their anticoagulant properties, PLA2 enzymes have been classified into strongly, weakly, or nonanticoagulant enzymes.5, 6 Strongly anticoagulant PLA2 enzymes inhibit blood coagulation below 2 µg/mL, whereas weakly anticoagulant enzymes inhibit between 3 and 10 µg/mL. Nonanticoagulant enzymes do not display anticoagulant activities even at 15 µg/mL. As many coagulation complexes
depend on phospholipids for their formation and function, it was thought that PLA2 enzymes exhibit their anticoagulant function primarily through their ability to hydrolyze phospholipids. However, the results from the experiments to study the role of phospholipid hydrolysis in the anticoagulant effects of PLA2 enzymes were contradictory. For example, several studies indicated that the enzymatic activity of PLA2 enzymes is essential for their anticoagulant activities. (a) The modification of active site His48 by p-bromophenacyl bromide led to the loss of both enzymatic and anticoagulant activities. (b) The replacement of hydrolyzable phospholipids by nonhydrolyzable phospholipids led to loss of anticoagulant activities. (c) Longer times of incubation of PLA2 enzymes with plasma led to increased phospholipid hydrolysis and clotting times. On the other hand, several other studies suggested that the enzymatic activity of PLA2 enzymes is not essential for their anticoagulant activities. (a) The catalytic efficiency of anticoagulant PLA2 enzymes did not correlate with their anticoagulant potency. (b) There is a lack of correlation between plasma lipid hydrolysis and anticoagulant effects of the PLA2 enzymes. (c) The addition of antibodies to PLA2-hydrolyzed phospholipids reversed the anticoagulant effects. (d) Chemical modifications have shown that the loss of enzymatic activity led to little effect on anticoagulant effects, and vice versa (for details, see review7). The controversies in the field were essentially due to two critical but wrong assumptions, namely, the anticoagulant mechanism of different PLA2 enzymes is the same and the phospholipid requirements of different coagulation complexes are alike.
depend on phospholipids for their formation and function, it was thought that PLA2 enzymes exhibit their anticoagulant function primarily through their ability to hydrolyze phospholipids. However, the results from the experiments to study the role of phospholipid hydrolysis in the anticoagulant effects of PLA2 enzymes were contradictory. For example, several studies indicated that the enzymatic activity of PLA2 enzymes is essential for their anticoagulant activities. (a) The modification of active site His48 by p-bromophenacyl bromide led to the loss of both enzymatic and anticoagulant activities. (b) The replacement of hydrolyzable phospholipids by nonhydrolyzable phospholipids led to loss of anticoagulant activities. (c) Longer times of incubation of PLA2 enzymes with plasma led to increased phospholipid hydrolysis and clotting times. On the other hand, several other studies suggested that the enzymatic activity of PLA2 enzymes is not essential for their anticoagulant activities. (a) The catalytic efficiency of anticoagulant PLA2 enzymes did not correlate with their anticoagulant potency. (b) There is a lack of correlation between plasma lipid hydrolysis and anticoagulant effects of the PLA2 enzymes. (c) The addition of antibodies to PLA2-hydrolyzed phospholipids reversed the anticoagulant effects. (d) Chemical modifications have shown that the loss of enzymatic activity led to little effect on anticoagulant effects, and vice versa (for details, see review7). The controversies in the field were essentially due to two critical but wrong assumptions, namely, the anticoagulant mechanism of different PLA2 enzymes is the same and the phospholipid requirements of different coagulation complexes are alike.
To resolve the controversies and decipher the mechanism of anticoagulant activity, three anticoagulant PLA2 enzymes— CM-I, CM-II, and CM-IV from the venom of the black-necked spitting cobra (Naja nigricollis), were studied.8 Based on their anticoagulant properties, CM-I and CM-II are classified as weakly anticoagulant enzymes, whereas CM-IV, which is at least 100-fold more potent than CM-I and CM-II, is classified as strongly anticoagulant enzyme.9 As a first step, the coagulation complexes that are inhibited by these enzymes were identified using the “dissection approach” (see FIGURE 113.2), followed by their effects on reconstituted coagulation complexes. CM-IV was reported to inhibit the coagulation cascade at two different points—the extrinsic tenase complex as well as the prothrombinase complex.10 Further studies have identified that while it inhibits the extrinsic tenase complex through a combination of enzymatic and nonenzymatic mechanisms, the inhibition of the prothrombinase complex, however, is independent of phospholipid hydrolysis.11 This inhibition is achieved through competing with factor Va (FVa) in binding to factor Xa (FXa), hence blocking the formation of the prothrombinase complex. It forms a 1:1 complex with FXa, with a dissociation constant (Kd) of 500 nM.12 On the other hand, CM-I and CM-II inhibits only at a single point in the coagulation cascade, at the extrinsic tenase complex, solely through enzymatic hydrolysis of phospholipids. Unlike CM-IV, CM-I and CM-II display no effect toward the prothrombinase complex.13
As mentioned previously, although PLA2 enzymes possess similar folds and three-dimensional (3-D) structures, they display a vast array of biologic functions. This indicates that their structure-function relationships are subtle and complex. By a systematic analysis, the anticoagulation site was identified to be situated between residues 54 and 77 in strongly anticoagulant PLA2 enzymes.13 This predicted site was well supported by subsequent experiments.9, 14, 15, 16 This region is positively charged and is located on the surface of the molecule, thus accessible for interactions (FIGURE 113.1). This region contains four positively charged lysine residues that may play a role in intermolecular interactions.9, 16 In the case of protein CM-IV, a comparison of amino acid sequences with bovine FX, FV, prothrombin, and tissue factor (TF) revealed that half of CM-IV’s anticoagulant region was homologous with the light chain of FVa and the other half with a section of TF.11 These two sites are hypothesized to be responsible for targeting the prothrombinase and the extrinsic tenase complexes respectively.17
Metalloproteinases
Metalloproteinases are zinc-dependent endoproteolytic enzymes that cleave peptide bonds in the middle of the proteins. They form three different classes, P-I, P-II, and P-III, based on their domain structure and size.18, 19, 20 The snake venom metalloproteinases exert digestive (of prey), hemorrhagic, procoagulant, anticoagulant, and antiplatelet effects.18 Most snake venom metalloproteinases are α-fibrinogenases that selectively cleave the Aα chain of fibrinogen. They inhibit blood coagulation as they produce truncated fibrinogen, which is unable to form a fibrin clot as stable as the native fibrinogen. The structure-function relationships of these metalloproteinases with respect to their anticoagulant effects have not yet been studied.
Serine Proteases
Protein C activators are the only serine proteases that indirectly inhibit coagulation. Under normal physiologic conditions, protein C is activated by thrombin and thrombomodulin to activated protein C (APC). APC exerts its anticoagulant activity by degrading FV/FVa and FVIII/FVIIIa. It also stimulates fibrinolysis by interacting with plasminogen activator inhibitor.21 Protein C activators are approximately 36 to 40 kDa in molecular weight, and they activate protein C in the absence of Ca2+ with low salt concentrations.22, 23, 24 A protein C activator isolated from the venom of Agkistrodon contortrix contortrix activates protein C through thrombomodulin-independent mechanisms.22 The stretch of positively charged residues on its interfacial surface, together with three carbohydrate moieties surrounding the catalytic site, are pivotal in protein C recognition, binding, and activation.25
Nonenzymatic Anticoagulants
C-type Lectin-related Proteins
C-type lectins are calcium-dependent carbohydrate-binding proteins that agglutinate red blood cells. They usually exist as homodimers. On the other hand, C-type lectin-related proteins are usually heterodimers or oligomeric complexes of heterodimers, and they do not have lectin-like activity.26, 27, 28 Anticoagulant C-type lectin-related proteins were one of the first few nonenzymatic proteins isolated and characterized from snake venoms.17 From the venoms of the hundred-pace pit viper (Deinagkistrodon acutus) and Stejneger’s bamboo viper (Trimeresurus stejnegeri), anticoagulant proteins that inhibit prothrombinase activation were isolated and purified.29, 30, 31, 32 Although they were shown to perform inhibition through nonenzymatic mechanism, details on their mechanism and structure-function relationships are still unclear. A specific FIX-binding protein
and FX-binding protein from the venoms of Protobothrops flavoviridis (formerly, Trimeresurus flavoviridis) and D. acutus, respectively, were isolated and characterized. Both are heterodimeric, with α- and β-chains, and both chains are structurally similar to C-type lectins. They were shown to bind and inhibit the coagulation factors FIX and FX respectively, with nanomolar and subnanomolar affinities. The dimeric interface created by domain swapping recognizes the Gla domain of FX and FIX.33
and FX-binding protein from the venoms of Protobothrops flavoviridis (formerly, Trimeresurus flavoviridis) and D. acutus, respectively, were isolated and characterized. Both are heterodimeric, with α- and β-chains, and both chains are structurally similar to C-type lectins. They were shown to bind and inhibit the coagulation factors FIX and FX respectively, with nanomolar and subnanomolar affinities. The dimeric interface created by domain swapping recognizes the Gla domain of FX and FIX.33
Another group of anticoagulant C-type lectin-related proteins interact specifically with thrombin/prothrombin. This includes bothrojaracin34, 35 from Bothrops jararaca and bothroalternin36 from Bothrops alternatus venoms. Bothrojaracin inhibits thrombin directly through both exosite 1 and exosite 2, which accounts for its high affinity toward thrombin with a Kd of 0.6 nM. However, it does not bind to the active site of thrombin. Due to its binding to thrombin’s exosite 1, it also inhibits FV activation by thrombin.37 In prothrombin, bothrojaracin binds to partially exposed anion-binding exosite (pro-exosite 1) with a Kd of 175 nM, thus inhibiting the activation of prothrombin to thrombin.38
Three-finger Toxins
Three-finger toxins are a family of nonenzymatic proteins that are commonly found in the venom of snakes.39 They range from 60 to 74 amino acid residues in length. Their structure is unique, with three β-stranded loops extending from a central core, which is held by four to five conserved disulphide bridges. This gives it an appearance of three extended fingers, hence its name. Despite the overall similarity in structure, three-finger toxins display a great diversity of biologic functions. Members of this family include α-neurotoxins,40, 41 κ-bungarotoxins,42 muscarinic toxins,43 fasciculins,44 calciseptine,45, 46 cardiotoxins (cytotoxins),47, 48 dendroaspins,49 and anticoagulant proteins.50, 51 An example of an anticoagulant three-finger toxin is the Hemextin AB complex, isolated from the ring-necked spitting cobra, Hemachatus haemachatus.51 This complex consists of two subunits, Hemextin A and Hemextin B, and inhibits the extrinsic tenase complex with a Ki of 50 nM. Interestingly, subunit Hemextin B by itself does not display any anticoagulant effects, but instead synergistically enhances the anticoagulant effects of Hemextin A by forming a complex with it.52
Anticoagulants from Hematophagous Animals
In hematophagous organisms, blood meals from their hosts are crucial for survival. This process is not trivial as the hosts defend themselves by a variety of responses at the feeding site, which involves platelet activation and aggregation, local vasoconstriction, blood coagulation, and raising an immune response. To overcome such host responses, blood-feeding organisms produce a pharmacopeia of molecules in their saliva, from anticoagulants and platelet aggregation inhibitors to vasodilators, anti-inflammatory, and immunomodulatory molecules. The blood-feeding habit is found in several thousands of species in nature, and in arthropods alone blood-feeding habit has evolved at least 20 times independently, resulting in a vast molecular diversity of antihemostatic molecules. Thus, it is not uncommon to observe functional diversity among closely related proteins and functional convergence among structurally unrelated proteins. The saliva of these animals provides an excellent source of novel anticoagulant proteins and peptides, which differ in structure and mechanism of action. Based on this mechanism of action of the anticoagulants, they can be broadly classified into thrombin inhibitors, FXa inhibitors (FXaIs), extrinsic tenase complex inhibitors, and intrinsic tenase complex inhibitors.
Thrombin Inhibitors
There are several classes of thrombin inhibitors isolated from hematophagous animals (FIGURE 113.3). Each class of thrombin inhibitors shows distinct protein folds, and hence, they have evolved through convergent evolution. They also inhibit thrombin by different mechanisms.
Hirudin
Hirudin, isolated from the medicinal leech, Hirudo medicinalis, is probably the most prominent and well-characterized thrombin inhibitor. Reported more than 100 years ago, hirudin is a 65-residue protein that is specific for thrombin, with a Ki of 22 fM.53 A sulphated tyrosine (Tyr64) on hirudin has been identified to play a significant role in interactions with thrombin, which, when removed, results in a binding to thrombin 10 times weaker.54 Hirudin’s first three N-terminal residues bind to the hydrophobic pocket of thrombin’s active site in a noncanonical form, through hydrogen bonds. The C-terminus on the other hand is rich in acidic residues and through specific electrostatic interactions binds to exosite-I of thrombin.55, 56, 57, 58, 59 Thus, via these two interactions, hirudin inhibits specifically thrombin as a tight-binding inhibitor. Apart from hirudin, there have also been many inhibitors from other species of leeches that belong to this family of proteins that were subsequently isolated.60, 61
Haemadin
Another molecule, haemadin, was also isolated from leeches. Found in Haemadipsa sylvestris, the Indian leech, haemadin is 57-amino acid residue in length, slightly smaller than hirudin.62 It is a slow and tight-binding inhibitor of thrombin, with a Ki of 210 fM. Although haemadin’s sequence similarity with hirudin is low, they share a common 3-D fold, where its globular N-terminal core is stabilized by three disulphide bridges with an extended C-terminal tail.63 Like hirudin, the first three N-terminal residues bind to active site of thrombin in a noncanonical form. However, haemadin’s acidic C-terminus binds to thrombin’s exosite-II, instead of exosite-I as in the case of hirudin63, 64 (see FIGURE 113.4).
Kunitz-type Thrombin Inhibitors
The Kunitz-type inhibitors are a family of proteins belonging to the serine protease inhibitors.65 They have a characteristic reactive-site loop, which binds and runs antiparallel to the enzyme active site residues. This type of inhibitors is commonly found in ticks. However, based on their sequences, there appears to be two different subclasses of these inhibitors, which originate from the two different families of tick—Ixodidae (hard ticks) and Argasidae (soft ticks). From the hard ticks, inhibitors that have been isolated include amblin from Amblyomma hebraeum,66 boophilin from Boophilus microplus,67 and haemalin from Haemaphysalis longicornis.68 With two tandem Kunitz domains, these proteins generally have a lower affinity for thrombin as compared to those from the soft ticks (see below), with a Ki of 20 nM for amblin66 and 1.8 nM for boophilin.67 On the other hand, Kunitz-type inhibitors from soft ticks include ornithodorin from Ornithodoros moubata,69 savignin from Ornithodoros savignyi,70, 71 and monobin from Argas monolakensis.72 They are slow, tight-binding, and competitive inhibitors of thrombin, with a Ki of 4.89 pM for savignin70 and 7 pM for monobin.72
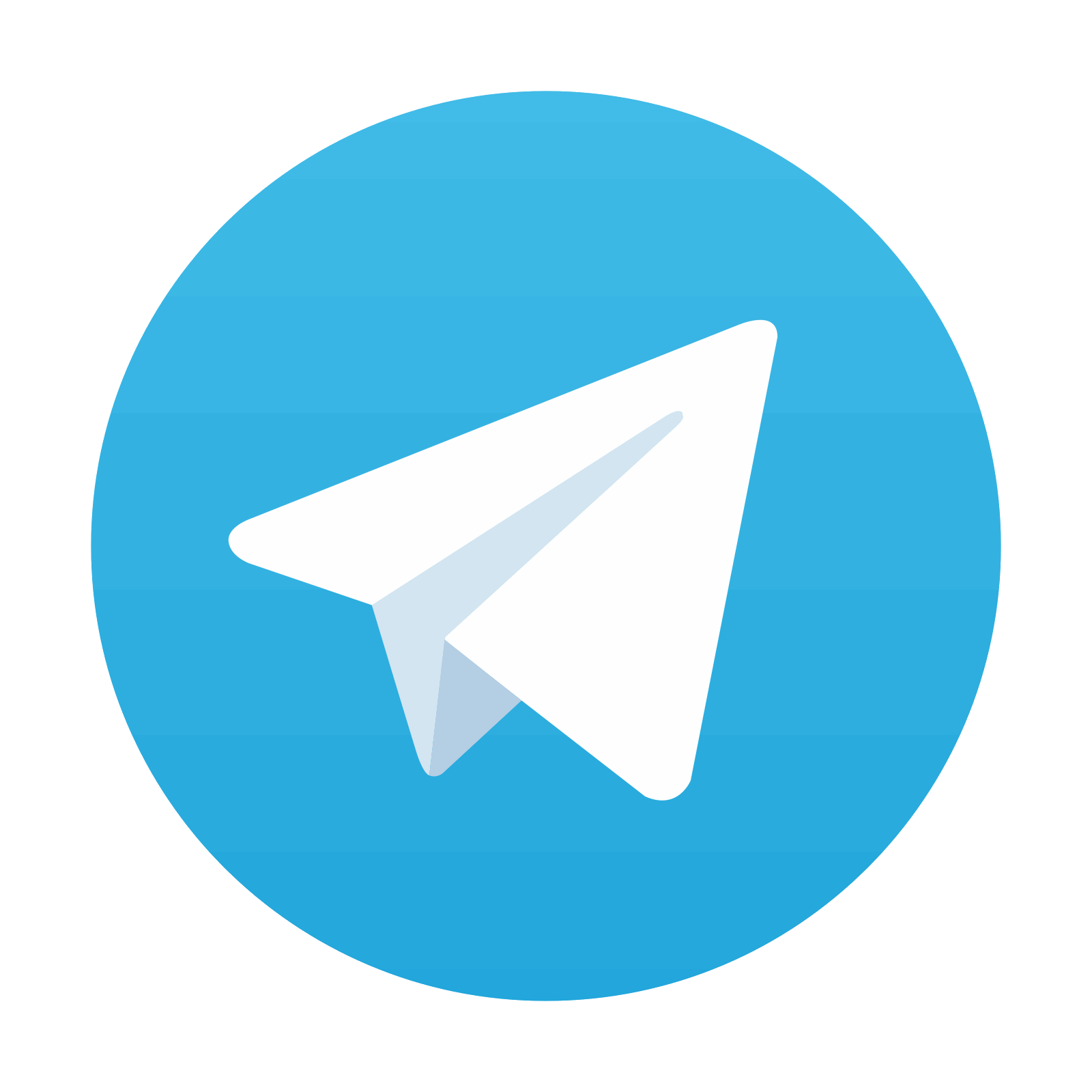
Stay updated, free articles. Join our Telegram channel

Full access? Get Clinical Tree
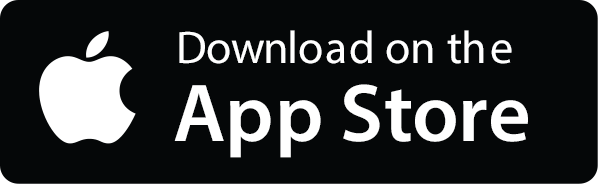
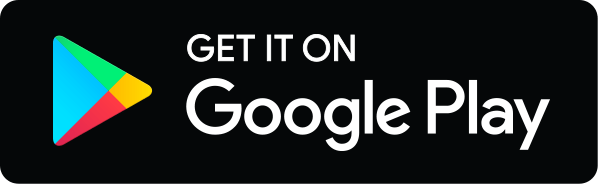
