In vivo animal models have proven very useful to the understanding of basic biologic pathways of the immune system, a prerequisite for the development of innovate therapies. This article addresses currently available models for defined human monogenetic defects of neutrophil granulocytes, including murine, zebrafish, and larger mammalian species. Strengths and weaknesses of each system are summarized, and clinical investigators may thus be inspired to develop further lines of research to improve diagnosis and therapy by use of the appropriate animal model system.
Key points
- •
Human granulocyte diseases are mostly monogenic diseases affecting neutrophils, not eosinophils and basophils.
- •
Most monogenic neutrophil diseases are recessive or X-linked and can be classified as chronic granulomatous disease (CGD), leukocyte adhesion deficiency (LAD), or severe congenital neutropenia (SCN). Each of these can be caused by mutations in different genes.
- •
For CGD and LAD, mice bred to lack the orthologous gene mutated in each human form usually have a phenotype similar to that of the human patients. In contrast, for SCN, there is a striking discrepancy between the phenotypes of model mice and human patients.
- •
Not all animal models are mouse models. For some neutrophil diseases, there are naturally occurring models in dogs or cows. The construction of zebrafish models is an emerging trend in neutrophil diseases.
- •
Opportunities to characterize new monogenic forms of SCN, to generate new mouse models by random mutagenesis, to engineer new zebrafish models, and to use animal models in the exploration of new treatments should occupy researchers studying animal models of neutrophil diseases for many years to come.
Introduction
Granulocytes are immune cells that contain within them punctate granules, visible under the light microscope. There are 3 categories of granulocytes: basophils, neutrophils, and eosinophils, that are distinguished by whether they can be usefully stained by basic (high pH), neutral (medium pH), or acidic (low pH) stains. Eosinophils may have several roles in host defense and have been most widely studied in asthma. Both eosinophils and basophils have roles in promoting the Th2 responses needed to defend against parasitic infections. However, a search of OMIM (Online Mendelian Inheritance in Man) shows no monogenic disorders primarily attributed to eosinophil or basophil dysfunction. By laboratory assays, humans have been found with a partial or total lack of peroxidase in eosinophil granules, but they have mild anemia or no phenotype at all. Ohnmacht and colleagues generated mice lacking basophils, which will enable targeted studies of human diseases in which basophils may be important. The immune function of neutrophils and their roles in disease are much better characterized than those of basophils and eosinophils, so neutrophils and their diseases are focused on entirely in this article.
Neutrophils are first responders at sites of infection and part of the innate immune system. Neutrophils can fight an infection by diverse weapons: phagocytosis of the infecting microbe, poisoning the microbe with toxic peptides stored in the granules, and wrapping the microbe in neutrophil extracellular traps. More generally, neutrophils enhance inflammation and inflammatory signals that eventually call in macrophages, T cells, and B cells for a more long-lasting attack on the infection. Consistent with the paradigm that the innate immune system is more primitive than the adaptive immune system, all jawed vertebrates are thought to have neutrophils or neutrophil-like cells called heterophils, which can kill microbes by phagocytosis. Even some invertebrates, such as flies, have neutrophil-like cells. One reason eosinophils are thought be important for parasitic infections is that parasites, such as helminths, are too large to be killed by phagocytosis.
Not only are neutrophils conserved across jawed vertebrates, but so are most of the known genes mutated in human neutrophil diseases. This evolutionary perspective suggests that it might be possible to model each human neutrophil disease of known monogenic cause by knocking out the corresponding gene in a mouse and characterizing the mouse phenotype. One could also hope to discover new human diseases by first studying abnormal mice that turn out to have a neutrophil disease. In a few cases, fish have been deliberately used instead of mice; also included are naturally occurring models of 2 diseases in dogs and 1 disease in cows.
Classification of Neutrophil Diseases
Most human monogenic neutrophil diseases can be classified according to the type of malfunction. Conditions in which neutrophil defense is inadequate because the number of circulating neutrophils are too low, as measured by the absolute neutrophil count (ANC), are called neutropenia. Chronic benign and ethnic neutropenias are not discussed, but the focus instead is on neutropenias that may start at birth and that are associated with severe bacterial infections, called severe congenital neutropenia (SCN). Diseases in which neutrophils fail to migrate properly to sites of infection are called leukocyte adhesion deficiency (LAD). Diseases in which neutrophils fail to carry out the oxidative burst function are called chronic granulomatous disease (CGD). Based on these layman’s descriptions, one might imagine that SCN would be easiest to explain at a molecular level and CGD would be the hardest to explain at a molecular level; the causes of neutropenia would be most conserved across species and the causes of CGD least conserved across species. Surprisingly, the opposite is true for both measures of complexity. Therefore, relevant animal models are presented in the following order: CGD, then LAD, then SCN, from best understood to least understood. One rare disease, due a mutation in RAC2 , is at the boundary of CGD and LAD and that disease is described in the LAD section. Two monogenic diseases that do not fit into this 3-part classification and some complex neutrophil diseases are described in a fourth section.
Over 20 years of animal case studies are summarized to address the basic evolutionary question: For which genes does conservation of the gene imply conservation of the phenotype when the gene is mutated? From the way the question is posed, one should expect that the phenotype is conserved for some genes, but not for other genes. Clinicians may wish to know when the phenotype is conserved because for those genes and only for those genes, animal models should be useful to understand the cellular mechanism of the disease and to test treatments.
Introduction
Granulocytes are immune cells that contain within them punctate granules, visible under the light microscope. There are 3 categories of granulocytes: basophils, neutrophils, and eosinophils, that are distinguished by whether they can be usefully stained by basic (high pH), neutral (medium pH), or acidic (low pH) stains. Eosinophils may have several roles in host defense and have been most widely studied in asthma. Both eosinophils and basophils have roles in promoting the Th2 responses needed to defend against parasitic infections. However, a search of OMIM (Online Mendelian Inheritance in Man) shows no monogenic disorders primarily attributed to eosinophil or basophil dysfunction. By laboratory assays, humans have been found with a partial or total lack of peroxidase in eosinophil granules, but they have mild anemia or no phenotype at all. Ohnmacht and colleagues generated mice lacking basophils, which will enable targeted studies of human diseases in which basophils may be important. The immune function of neutrophils and their roles in disease are much better characterized than those of basophils and eosinophils, so neutrophils and their diseases are focused on entirely in this article.
Neutrophils are first responders at sites of infection and part of the innate immune system. Neutrophils can fight an infection by diverse weapons: phagocytosis of the infecting microbe, poisoning the microbe with toxic peptides stored in the granules, and wrapping the microbe in neutrophil extracellular traps. More generally, neutrophils enhance inflammation and inflammatory signals that eventually call in macrophages, T cells, and B cells for a more long-lasting attack on the infection. Consistent with the paradigm that the innate immune system is more primitive than the adaptive immune system, all jawed vertebrates are thought to have neutrophils or neutrophil-like cells called heterophils, which can kill microbes by phagocytosis. Even some invertebrates, such as flies, have neutrophil-like cells. One reason eosinophils are thought be important for parasitic infections is that parasites, such as helminths, are too large to be killed by phagocytosis.
Not only are neutrophils conserved across jawed vertebrates, but so are most of the known genes mutated in human neutrophil diseases. This evolutionary perspective suggests that it might be possible to model each human neutrophil disease of known monogenic cause by knocking out the corresponding gene in a mouse and characterizing the mouse phenotype. One could also hope to discover new human diseases by first studying abnormal mice that turn out to have a neutrophil disease. In a few cases, fish have been deliberately used instead of mice; also included are naturally occurring models of 2 diseases in dogs and 1 disease in cows.
Classification of Neutrophil Diseases
Most human monogenic neutrophil diseases can be classified according to the type of malfunction. Conditions in which neutrophil defense is inadequate because the number of circulating neutrophils are too low, as measured by the absolute neutrophil count (ANC), are called neutropenia. Chronic benign and ethnic neutropenias are not discussed, but the focus instead is on neutropenias that may start at birth and that are associated with severe bacterial infections, called severe congenital neutropenia (SCN). Diseases in which neutrophils fail to migrate properly to sites of infection are called leukocyte adhesion deficiency (LAD). Diseases in which neutrophils fail to carry out the oxidative burst function are called chronic granulomatous disease (CGD). Based on these layman’s descriptions, one might imagine that SCN would be easiest to explain at a molecular level and CGD would be the hardest to explain at a molecular level; the causes of neutropenia would be most conserved across species and the causes of CGD least conserved across species. Surprisingly, the opposite is true for both measures of complexity. Therefore, relevant animal models are presented in the following order: CGD, then LAD, then SCN, from best understood to least understood. One rare disease, due a mutation in RAC2 , is at the boundary of CGD and LAD and that disease is described in the LAD section. Two monogenic diseases that do not fit into this 3-part classification and some complex neutrophil diseases are described in a fourth section.
Over 20 years of animal case studies are summarized to address the basic evolutionary question: For which genes does conservation of the gene imply conservation of the phenotype when the gene is mutated? From the way the question is posed, one should expect that the phenotype is conserved for some genes, but not for other genes. Clinicians may wish to know when the phenotype is conserved because for those genes and only for those genes, animal models should be useful to understand the cellular mechanism of the disease and to test treatments.
Chronic granulomatous disease
CGDs are primary immunodeficiencies characterized by recurrent infections and inflammatory lesions called granulomas. The granulomas can occur on the skin or in the liver, lymphatic system, spleen, gastrointestinal tract, or genital tract. Mechanistically, CGD occurs because of a defect in the respiratory burst oxidase activity in neutrophils. More specifically, the nicotinamide adenine dinucleotide phosphate (NADPH) oxidase complex does not produce adequate amounts of superoxide and therefore killing of microbes is impaired.
There are 5 phenotypically comparable CGDs caused by defects in any of 5 proteins that participate in the NADPH oxidase complex. These proteins are traditionally denoted according to their approximate molecular weights: p22, p40, p47, p67, gp91; the “g” in gp91 indicates that this protein is glycosylated; often, a superscript “phox” is added to all 5 protein names, but the authors omit that. Defects in a sixth participating protein RAC2 lead to a phenotype that overlaps CGD, but because RAC2 has other functions, that phenotype is more severe and is better classified as a leukocyte adhesion deficiency. The gene names are shown in Table 1 .
Protein | Gene | Human Mutation Reference | Mouse Model Reference |
---|---|---|---|
p22 | CYBA | ||
p40 | NCF4 | ||
p47 | NCF1 | ||
p67 | NCF2 | ||
gp91 | CYBB |
There are documented human patients with null mutations for 4 of the 5 types. For p40 deficiency, the only documented human patient has compound heterozygous mutations, one of which may not be null. Most human CGD patients in North America and Europe are men with a mutation in CYBB, encoding gp91, because CYBB is on the X chromosome. The other forms of CGD are autosomal recessive and affect men and women equally.
Mice with definitely or likely functional null mutations exist for all 5 types. Knockout mice for p40, p47, and gp91 were deliberately engineered. Mice with a homozygous mutation in p67 were generated via a cross of known mouse strains. Mice with a null substitution mutation in p22 were created by N-ethyl-N-nitrourea (ENU) mutagenesis.
The reported phenotypes for gp91, p47, and p40 knockout mice generally correspond to the phenotypes of the most severe human patients, with 1 proviso. Mouse studies typically report on immune response to challenge by a small number of microbial species, whereas CGD patients must cope with a plethora of infectious prokaryotes. Granulocytes of gp91-deficient male mice produce no superoxide. Clearance of infection by Staphylococcus aureus and Aspergillus fumigatus (2 infections commonly seen in CGD patients) was delayed in comparison to gp91-sufficient control mice. When gp91-deficient mice were injected with the irritant chemical thioglycollate, there was increased neutrophil recruitment near the site of injection. p47-deficient mice produce no superoxide in neutrophils. Despite the lack of superoxide, the knockout neutrophils have some capability to kill S aureus in vitro, but it is reduced compared with p47-sufficent neutrophils. p47 knockout mice developed spontaneous infections and had significantly lower survival than heterozygous or wild-type mice. Histologic postmortem examination identified granulomas similar to those seen in CGD patients. p40 knockout mice have a deficiency in production of reactive oxygen species in response to TNF-alpha and their neutrophils have difficulty killing S aureus in vitro.
The mouse model for the p67 form of CGD was obtained and characterized as follows. A back-cross of the C57BL/6J strain (used in many laboratories) to the wild MOLF/Ei strain leads to variability in susceptibility to salmonella infections. This variation is consistent with recessive inheritance of a single locus and can be mapped to a region of mouse chromosome 1 containing the Ncf2 gene, encoding p67. Sequencing identified that the susceptible mice are homozygous for a R394W mutation derived from the MOLF/Ei strain. A human CGD patient with an analogous R395W mutation has been reported. A functional assay suggested that R394W leads to a deficiency in superoxide production, which is a hallmark of CGD.
The most interesting of the CGD model mice is the one for p22 deficiency, which has a Y121H homozygous substitution in the protein. These mutant mice have the expected immunodeficiency, which in this study was demonstrated by challenge with Burkholderia, another infection commonly seen in CGD patients. Surprisingly, the p22 mutant mice also have a balance disorder because of faulty gravity sensing in the inner ear, which has not been reported for human patients with the p22 form of CGD. The molecular explanation for the balance defect is that there are several oxidase-producing complexes that share the p22 subunit; these complexes use different homologs of the gp91 subunit to partner with p22. One of these complexes, called Nox3, is needed for the generation of otoconia, which are calcium carbonate crystals in the inner ear used for gravity sensing. In contrast to the balance problem, the mice have normal hearing. The Y121H substitution does not truncate the protein in principle, but in practice it leads to no (stable) protein being translated, although there is mRNA. Thus, it may be considered equivalent to a functional null for purposes of modeling.
Leukocyte adhesion deficiency
In this section, 3 diseases, often called “leukocyte adhesion deficiency,” and a fourth one, which is called here “RAC2 disease,” are discussed. The 3 LADs are usually distinguished by Roman numerals, I, II, III. There are mouse models for each of the 4 diseases, and additional nonmurine animal models for 2 of them. For RAC2 disease, there are 2 zebrafish models that may be more useful than the knockout mice. There is one other nonmouse model for LAD type I, some Holstein cattle have a disease called bovine leukocyte adhesion deficiency (BLAD). The animal models for LAD are summarized in Table 2 . LAD I, II, III have autosomal-recessive inheritance. RAC2 disease is in principle autosomal dominant because the observed human mutations are heterozygous, but the observed human mutation D57N occurred de novo in the affected children and animal models suggest that biallelic null mutations of RAC2 could lead to the same disease.
Disease | Human Gene | Model Organism | Mutation | Reference(s) |
---|---|---|---|---|
LAD I | ITGB2 (CD18) | Mouse | Hypomorphic | |
LAD I | ITGB2 (CD18) | Mouse | Null | |
LAD I | ITGB2 (CD18) | Cows | D128G | |
LAD II | SLC35C1 | Mice | Null | |
LAD III | FERMT3 (kindlin) | Mice | Null and null only in chimeras and neutrophils | |
RAC2 disease | RAC2 (D57N mutation) | Mice | Null | |
RAC2 disease | RAC2 (D57N mutation) | Zebrafish | Morpholino or D57N |
Leukocyte Adhesion Deficiency, Type I
The mildest, most common, and first described LAD is type I, due to biallelic mutations in the integrin gene ITGB2, which codes for the protein usually called CD18. The phenotype includes recurrent bacterial infections, periodontitis, delayed wound healing, and granulocytosis. At the molecular level, neither the integrin β1 subunit nor its 3 α subunit partners come to the cell surface as they should, impairing neutrophil adhesion and chemotaxis. Two mouse models were generated that reflect a range of human LAD I phenotypes, depending on the severity of the mutations in ITGB2 . A first attempt to make a knockout mouse led inadvertently to a mouse with a low level of Itgb2 expression. The phenotype of the mouse with the hypomorphic mutation includes the following: mild granulocytosis, poor neutrophil mobilization to a chemically induced peritonitis model, and delayed rejection of heart transplants. The knockout mice have a more severe phenotype including leukocytosis, skin infections, alopecia, defective neutrophil adhesion and migration, and an impaired respiratory burst (resembling CGD). Knockout mice inoculated with the bacterium Streptococcus pneumoniae have much more difficulty clearing the infection than wild-type controls and many of the mice die of infection.
Cows with BLAD have a homozygous D128G substitution in ITGB2. The clinical symptoms overlap those of the human disease: high neutrophil counts, recurrent bacterial infections, periodontitis, and oral ulcers. Leukocytes of other types seem to try to compensate for a lack of innate defense by boosting levels of IgG. Chemotaxis and phagocytosis of affected granulocytes are severely impaired due to poor adhesion, whereas adhesion-independent functions are not impaired. BLAD is of economic importance in the dairy industry because some bulls used often in mating turned out to be carriers of the BLAD-causing mutation.
Leukocyte Adhesion Deficiency, Type II
LAD II has a more severe phenotype than LAD I because it includes developmental delay and short stature. LAD II is caused by biallelic mutations in SLC35C1, encoding a protein involved in the transport of fucose, one of the sugars involved in posttranslational protein modification. This modification is surprising because there is no obvious connection between fucosylation and CD18 or other integrins. The use of fucose has been partly conserved and studied in a variety of eukaryotes and prokaryotes. Indeed, 1 of the 2 seminal human studies reasoned that fucosylation pathway genes are conserved between humans and worms and used a complementation strategy in Caenorhabditis elegans to find that biallelic mutations of SLC35C1 cause LAD II.
For LAD II, Hellbusch and colleagues engineered mice that are homozygously null for Slc35c1 , which encodes the ortholog of the protein mutated in human LAD II. Mice are born in 1/2/1 proportions, so there is no prenatal lethality. The knockout mice have leukocytosis, especially elevated neutrophils and eosinophils, and poor postnatal survival. The mouse weights are reduced. Leukocyte rolling flux fractions and velocity are significantly reduced, perfectly consistent with the human LAD II phenotype.
In addition to obtaining these phenotypic characterizations, Hellbusch and colleagues used the Slc35c1−/− mice to gain insight into the pathways of fucose transport. Aleuria aurantia lectin (AAL) is specific for 3 types of fucosylation and can be used in vitro to show that Slc35c1−/− mouse embryonic fibroblasts (MEFs) have a defect in a fucosylation pathway. It was known from previous work that there are 2 pathways to obtain guanosine diphosphate-fucose for fucosylation: a de novo synthesis pathway and a salvage pathway. Fucose is needed in the endoplasmic reticulum (ER) and in the Golgi; the set of fucosyltransferases in those 2 organelles are nonoverlapping. Slc35c1 transports guanosine diphosphate-fucose only into the Golgi, not the ER. Nevertheless, the mouse phenotype can be improved (better weight and survival) by the administration of fucose, which must somehow get transported to the Golgi. This statement suggests that there is an alternative mechanism of transport that is less efficient, but works better as the concentration of fucose increases. Administration of fucose to 1 human LAD II patient similarly improved the patient’s condition, but for a different patient, the fucose treatment was ineffective. Further mouse studies, perhaps using mice in which 2 fucose pathways are disrupted, could give insight into the function of Slc5cl and its dysfunction in LAD II.
Leukocyte Adhesion Deficiency, Type III
LAD III is a severe form of LAD that combines the immunodeficiency of LAD I with a severe bleeding disorder due to faulty platelet function. LAD III is caused by biallelic mutations in FERMT3 , which encodes the protein kindlin 3. The bleeding can be more problematic clinically than the immunodeficiency. Analogously, Fermt3 knockout mice die shortly after birth because of the platelet disorder, and the initial characterization of these mice does not consider granulocytes. To address this limitation, Moser and colleagues went on to generate Fermt3−/− fetal liver chimeras and neutrophils. Using these cells, they could show that the neutrophils have an adhesion deficiency. They suggest that the granulocytosis in LAD III (and by inference in LAD I) seems to be a compensation for the inability of the granulocytes to adhere and migrate to infected sites. They connected LAD III to LAD I at the molecular level by showing that kindlin 3 binds to integrin β 2 , and they did integrin mutagenesis experiments to show that the NXXF motif at positions 764–767 is needed for kindlin-integrin binding. Although LAD III is fatal if untreated, it can be cured with hematopoietic stem cell transplant (HSCT) in some cases because FERMT3 , unlike other kindlin-encoding genes, is expressed only in cells of the hematopoietic lineage. One difference between the human LAD III phenotype and the knockout mouse phenotype is that human patient erythrocytes have a normal shape, but knockout mouse erythrocytes have an aberrant shape.
RAC2 Disease
RAC2 disease is functionally related to LAD and has been reported in 2 human patients, both carrying de novo heterozygous D57N mutations. The phenotype includes recurrent infections, abscesses, impaired wound healing, and lack of pus. The protein is translated at half the normal level, but the D57N mutation has a dominant negative functional effect. In vitro, the patient neutrophils were deficient in polarization, chemotaxis, azurophilic (but not specific) granule release, and superoxide anion production. The first patient was treated successfully with HSCT via donation from a sibling. The HSCT process ablated the original bone marrow, so that posttransplant, the cells are 100% donor-derived. This stringency is important because D57N is a dominant-negative substitution.
RAC2 disease overlaps CGD phenotypically because of the role of RAC2 in the NADPH complex, explained earlier. The disease is more severe because RAC2 has roles in cell adhesion, cell migration, and other pathways. Clarifying RAC2 functions and how they are disrupted in RAC2 disease is difficult because, in mammals, there are 3 paralogous members of the Rac subfamily of Rho GTPases that overlap in functionality and differ primarily by tissue expression. Rac1 is ubiquitously expressed; Rac2 is expressed solely in cells of the hematopoietic lineage, and Rac3 is expressed mostly in the brain.
Roberts and colleagues made Rac2−/− mice and Gu and colleagues engineered various single-gene and double-gene knockout mice for the Rac genes. They showed that Rac2-deficient cells have a defect in adhesion to fibronectin. The mice do not suffer from opportunistic infections, but are susceptible to Pseudomonas aeruginosa infection, if challenged. Rac2−/− mice have leukocytosis mostly because of a threefold increase in the ANC. Superoxide production is impaired in vitro, but in vivo it seems that Rac1 can substitute for Rac2 in stimulating superoxide production ; in the superoxide aspect of the phenotype, Rac2 deficiency matches CGD rather than LAD. Neutrophil chemotaxis is impaired and Rac2-deficient neutrophils had impaired spreading on surfaces after integrin ligation, which explains the similarity of Rac2 deficiency to LAD I. Rac2-deficient cells also have a significant reduction in proliferation and a significant increase in apoptosis. Rac2-deficient cells have a defect in actin polymerization and they do not migrate in response to SDF1 signaling.
Recently, Deng and colleagues developed zebrafish models for human RAC2 disease. First, they prepared a morpholino with impaired Rac2 expression; second, they engineered a knock-in of the human D57N mutation. Both fish models show similar aberrant phenotypes, strengthening the evidence that D57N causes disease by a dominant-negative mechanism rather than haploinsufficiency. Either expression of Rac2D57N or the morpholino model causes a failure in wound healing because of impaired neutrophil chemotaxis. In both models, neutrophils fail to respond to a P aeruginosa infection. Both models show a defect in polarization, which can be visualized because of the transparent nature of the zebrafish. Both models show neutrophilia without increased neutrophil production, consistent with the human phenotype. Even more interesting, the Rac2D57N mutant was used to study a neutropenia syndrome, called warts, hypogammaglobulinemia, infections, and myelokathexis (WHIM) syndrome, as described in the next section.
Severe congenital neutropenia
In SCN there is a primary immunodeficiency because the number of neutrophils in circulation is too low to fight infections adequately. In humans, an ANC less than 500/μl, when not infected or less than granulocyte colony stimulating factor (G-CSF) treatment, is considered neutropenic; some authors use a lower threshold of 200 when adding the adjective “severe.” Recombinant G-CSF boosts the ANC in most SCN patients and some untreated SCN patients mount a partial response to infections leading to temporarily higher ANC. The receptor for G-CSF is encoded by the gene CSF3R , and as one might expect, knocking out the corresponding gene in mice leads to neutropenia ( Table 3 ). Therefore, it is surprising that no SCN patients have been reported with biallelic inactivating mutations of CSF3R . Instead, some somatic mutations have been reported in patients undergoing G-CSF treatment. That inactivating mutations of CSF3R should lead to neutropenia is supported by the surprising discovery of a family in which the phenotype of neutrophilia is associated with a heterozygous T617N mutation that leads to a constitutively active form of the receptor.
Gene | Human Mutations/Inheritance | Mouse Mutation | Reference for Mouse Model | Are Mice Neutropenic? |
---|---|---|---|---|
CSF3 | None? | Null | Yes | |
CSF3R | Somatic mutations only(?) | Null | Yes | |
LYST | Biallelic/recessive | Null? ( beige ) | No? | |
LYST | Biallelic/recessive | Skip exon 25 ( gray ) | No? | |
ELA2 | Heterozygous/dominant | Null | No, but neutrophil function is aberrant | |
ELA2 | Heterozygous mutations/dominant | V72M heterozygous knock-in | No | |
ELA2 | Heterozygous mutations/dominant | G193X heterozygous or homozygous knock-in | No, but SCN can be induced by a proteasome inhibitor | |
AP3B1 | Biallelic/recessive | Null ( pearl ) | No | |
AP3B1 | Biallelic/recessive | Null ( bullet gray ) | No | |
RAB27A | Biallelic/recessive | Null ( ashen ) | Yes, after LCMV infection | |
GFI1 | Heterozygous/dominant negative | Null | Yes | |
GFI1 | Heterozygous/dominant negative | N382S retrovirally induced in cultured cells | Granulopoiesis blocked, but no ANC measured because this model is in vitro only | |
SLC37A4 | Biallelic/recessive | Null | Yes | |
SLC37A4 | Biallelic/recessive | Null + cell transfer | Yes | |
G6PC3 | Biallelic/recessive | Null | Yes | |
HAX1 | Biallelic/recessive | Null | No | |
TAZ | X-linked | Knock down by RNAi | Some animals |
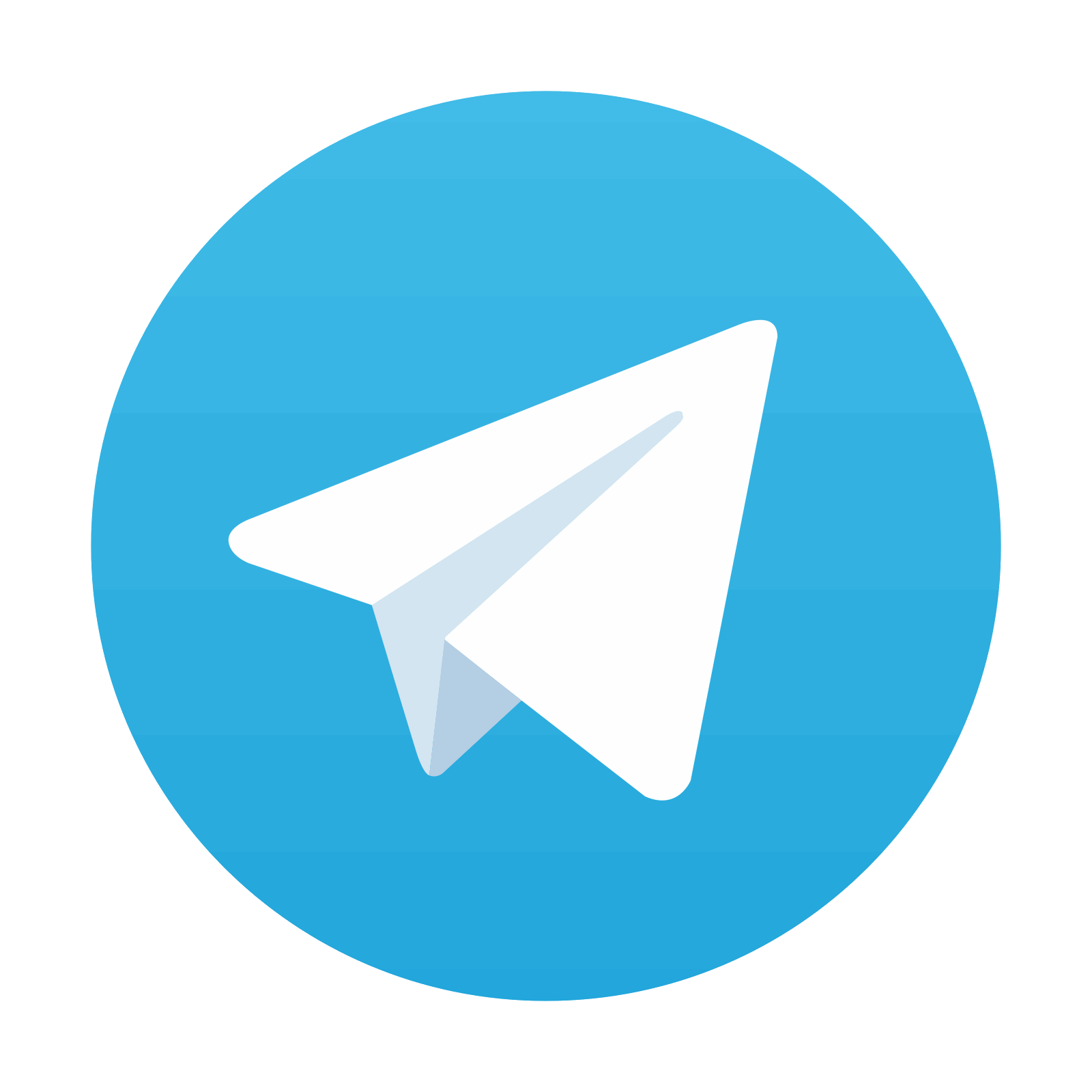
Stay updated, free articles. Join our Telegram channel

Full access? Get Clinical Tree
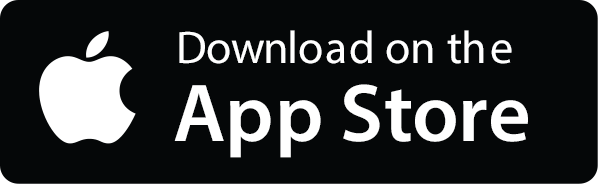
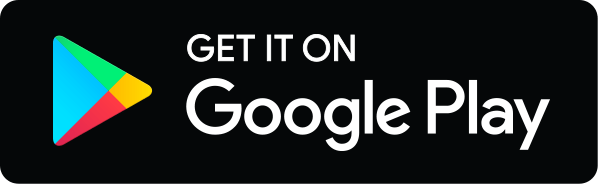
