Imaging system
Isotope
Radiotracer
Applied animal model
Reference
SPECT
99mTc
99mTc-BTAP-AnnexinV
Atrial thrombi
{Stratton, 1995 #4}
{Narula, 2001 #5}
99mTc-HYNIC-Annexin V
Jurkat T-cell lymphoblasts
{Blankenberg, 1999 #3}
{Blankenberg, 1999 #3} {Blankenberg, 2006 #2}
99mTc-HYNIC-Cys-Annexin V
Ischemia
{Fonge, 2008 #7}
99mTc-MA-G3-Annexin V
Turpentine-induced apoptosis
{Vanderheyden, 2006 #8}
99mTc-EC-Annexin V
Breast cancer
{Yang, 2001 #9}
{Kurihara, 2008#10}
99mTc-N2S2-Annexin V 118
Cyclophosphamide-induced apoptosis
{Tait, 2000 #11}
99mTc-(CO)3-Annexin V123
None
{Tait, 2012 #12}
99mTc-[C3((Bham)2]-Annexin V
Colorectal adenocarcinoma
{Ogawa, 2013 #13}
111ln
111ln DTPA-PEG-Annexin V
Breast cancer
{Wen, 2003 #18}
{Ke, 2004 #20}
PET
11C
11C-Annexin V
Doxorubicin-induced apoptosis, hypopharyngeal
{Cheng, 2012 #21}
{Cheng, 2013 #22}
18F
18F-SFB
Myocardial ischemia
{Grierson, 2004 #24}
{Torestsky, 2004 #26}
{Murakami, 2004 #27}
18F-FAN
Cycloheximide-induced apoptosis
{Yagle, 2005 #29}
18F-FBABM
None
{Li, 2008 #23}
64Cu-DTPA-Annexin V
{Cauchon, 2007 #31}
67,68 Ga-Annexin V
None
{Smith-Jones PM, 2003 #37}
{Bauwens, 2010 #38}
{Wangler, 2011 #36}
124I
124I-MBP-Annexin V
Fas-mediated apoptosis
{Dekker, 2005 #33}
{Dekker, 2005 #32}
MRI
Gd
Gd-DTP-Annexin V
Cardiovascular apoptosis
{Hiller, 2006 #39}
Gd-DTPA-BSA-Annexin V
Atherosclerotic lesion
{van Tilborg, 2006 #41}
{van Tilborg, 2010 #40}
CLIO
CLIO-Annexin V
None
{Schellenberger, 2002 #42}
{van Tilborg, 2009 #43}
{Oling, 2000 #44}
USPIO
USPIO-Annexin V
Fulminant hepatitis
(Yeh et al., unpublished data)
Optical imaging multiple modalities
Cy5.5-Annexin V
Lewis lung carcinoma
{Petrovsky, 2003 #45}
{Ntziachristos, 2004 #46}
Zn(2)+DPA-Annexin V
Prostate cancer or ionophore-induced apoptosis
{Smith, 2010 #48}
{Smith, 2011 #50}
MRI/Optical
Anx-CLIO-Cys5.5
Coronary artery occlusion
{Schellenberger, 2004 #51}
{Sosnovik, 2009 #52}
A5-pQD
Atherosclerotic lesion
{van Tilborg, 2006 #54}
{van Tilborg, 2010 #40}
SPECT/CT/Optical
111ln-Annexin V-CCPMs
Breast cancer
{Zhang, 2011 #56}
{Zhang, 2013 #57}
14.3 SPECT and PET Imaging
14.3.1 Single-Photon Emission Computed Tomography (SPECT) Imaging
Since the first apoptotic imaging reported in 1999 [4, 5], in vivo imaging of cell death with radiolabeled annexin V has been widely used in animal studies and clinical trials [6]. The unique advantages of radiotracers include their high sensitivity and the translational potential. Among various SPECT radionuclides, technetium-99 m (99mTc) is the most prominent isotope for the nuclear imaging because of its ideal nuclear properties and easy availability at low cost [7, 8].
Based on the previous study [9, 10], Blankenberg et al. reported an improved 99mTc-annexin V radioprobe using the bifunctional agent hydrazino nicotinamide (HYNIC) [5]. 99mTc-HYNIC-annexin V showed the greatest uptake in the kidneys, liver, and urinary bladder; however, it was devoid of any bowel excretion, resulting in excellent signal to background ratio in the abdominal region. With the modified procedure of preparation, 99mTc-HYNIC-annexin V could be synthesized efficiently with high yield. By far, 99mTc-HYNIC-annexin V has been extensively investigated in animal models [11–15]. Multiple clinical trials have confirmed the clinical utility of 99mTc-HYNIC-annexin V in determining the efficacy of chemotherapy in the patients of non-small cell lung cancer for the detection of apoptotic regions [16, 17].
In 2000, Tait et al. reported 99mTc-HYNIC-cys-annexin V117, which revealed site-specific labeling; however, it showed similar apoptosis avidity when compared to the previous version of 99mTc-HYNIC-annexin V [18]. Similar radiolabeled annexin V probes such as 99mTc(CO)3-HIS-cys-AnxV [19, 20] and 99mTc-His10-annexin V [21] demonstrated improved sensitivity for detecting dead or dying cells.
Yang et al. reported the use of 99mTc-EC-annexin using ethylenedicysteine (EC) as a chelator to assess the level of apoptosis of tumor cell [26]. The preclinical data of breast cancer patients showed the total effective dose equivalent for 99mTc-EC-annexin V of 6.80–7.89 mSv could be reasonable and allow it for clinical use and it could be a predictor for evaluating the treatment-related apoptosis after induction of chemotherapy [27].
Recently, 99mTc-C3(BHam)2-annexin V was developed using a bis(hydroxamide) derivative [C3(BHam)2] as a bifunctional chelating agent [28]. In vivo evaluation of 99mTc-C3(BHam)2-annexin V showed decreased uptake and retention in nonspecific tissues and much lower kidney accumulation of radioactivity when compared to 99mTc-HYNIC-annexin V. Their findings also indicated that 99mTc-C3(BHam)2-annexin V could be a potential candidate as a predictor for response to chemotherapy.
14.3.2 Positron Emission Tomography (PET) Imaging
The major advantages of PET imaging over SPECT are its much higher sensitivity, spatial resolution, and quantitative imaging; therefore, annexin V has been radiolabeled with fluorine 18 (18F) and many other isotopes for positron emission tomography.
18F-labeled annexin V with N-succinimidy-4-18F-fluorobenzoate (18F-SFB) has been investigated by several groups [29, 30] [31]. These studies of 18F-SFB annexin V demonstrated comparable apoptotic imaging feasibility to 99mTc-labeled annexin V and a fast clearance [31]. Moreover, 18F-SFB annexin V showed a significant higher accumulation in the mice treated with doxorubicin when compared to the control group [30].
Annexin V can also be labeled with thiol-reactive agents such as N-substituted maleimides, and iodoacetamide can be used to modify proteins at cysteines at specific sites [32].18F-N-[2-(4-fluorobenzamido)ethyl]maleimide (18F-FBEM) was used to label thiol-containing proteins as a novel site-specific labeling prosthetic group [33, 34]. Compared to the previous generation of 18F-SFB-labeled annexin V, the novel 18F-FBEM-cys-annexin V showed faster renal and a lesser extent of hepatobiliary excretion in normal mice and more sensitivity of site-specific detection in the rats of hepatic apoptosis model [35].
14.4 MRI Imaging
One of the main differences between magnetic resonance imaging (MRI) scan and other imaging modalities like PET is that MRI scan which reveals high spatial resolution allows scientists to navigate through the entire living organism, down to the cellular level. Several annexin V-based contrast agents have been developed. However, due to the fundamentally low sensitivity of MRI, how to deliver sufficient contrast agents safely and acquire sufficient imaging signals in vivo is definitely the concern.
14.4.1 T-Positive Images: Gadolinium-Labeled Annexin V
To access the redistribution of phosphatidylserine in the event of apoptosis, annexin V was linked to gadolinium diethylenetriamine pentaacetate (Gd-DTPA)-coated liposomes [36]. A significant increase in signal intensity was visible in those regions containing cardiomyocytes in the early stage of apoptosis. The in vivo Gd-DTPA-annexin V MRI imaging provided a rapid targeting of apoptotic cells in the ischemic and reperfused myocardium. Moreover, van Tilborg and his colleagues reported Gd-DTPA-bis(stearylamide) (Gd-DTPA-BSA)-labeled annexin V, the multiple functions of lipid-based bimodal contrast agent, enables the detection of apoptotic cells with both MRI and optical techniques [37]. Gd-DTPA-BSA was covalently coupled multiple human recombinant annexin V to introduce specificity for apoptotic cells. The imaging results showed a significant increase of the relaxation rates of apoptotic cell pellets when compared to the untreated control cells, which may have applications for the in vivo detection of apoptosis. In 2010, the same group developed a small micellar annexin A5-functionalized nanoparticle for noninvasive MRI and fluorescent imaging of PS exposing cells in atherosclerotic lesions [38]. In vivo MRI images of the abdominal aorta in atherosclerotic ApoE(−/−) mice revealed enhanced uptake of the annexin A5-micelles as compared to control micelles, which was corroborated with ex vivo near-infrared fluorescence images of excised whole aortas.
14.4.2 T2-Negative Images: Iron Oxide-Labeled Annexin V
Compared to T1 agents, superparamagnetic iron oxide nanoparticle-based T2 agents are assumed to be the preferred MRI contrast agents for evaluating apoptosis due to their high sensitivity. Up to date, the common labeling approach for apoptotic imaging is based on cross-linked derivative of monocrystalline iron oxide (MION), also known as cross-linked iron oxide (CLIO) [39].
Annexin V-CLIO allowed the identification of cell suspensions containing apoptotic cells by MRI even at very low concentrations of magnetic substrate [40]. Van Tilborg et al. investigated the internalization of, when co-exposed to apoptotic stimuli, annexin A5 was shown to internalize into endocytic vesicles by using annexin A5-functionalized iron oxide particles [41].
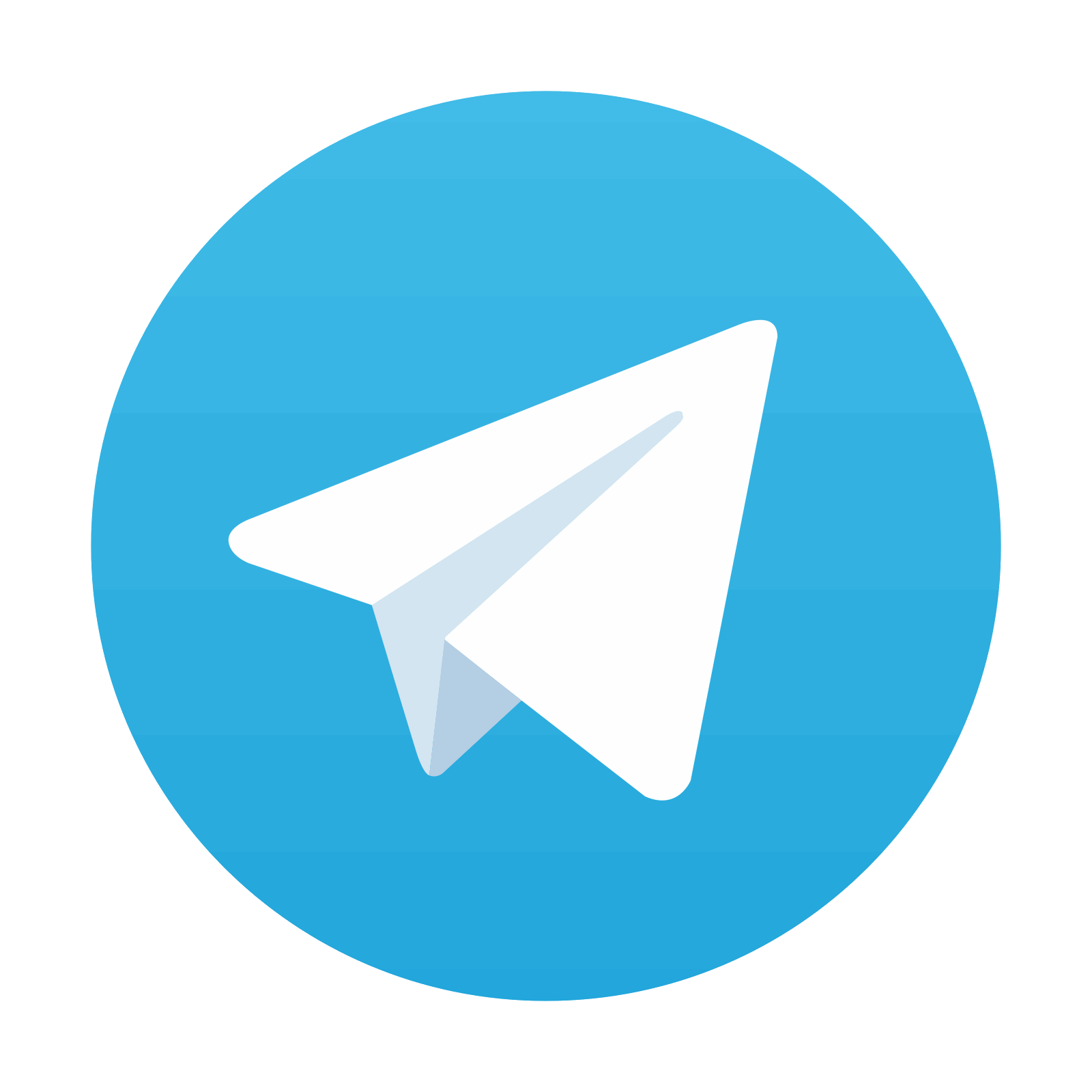
Stay updated, free articles. Join our Telegram channel

Full access? Get Clinical Tree
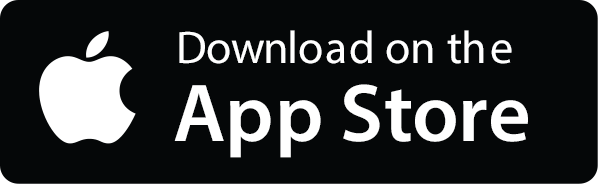
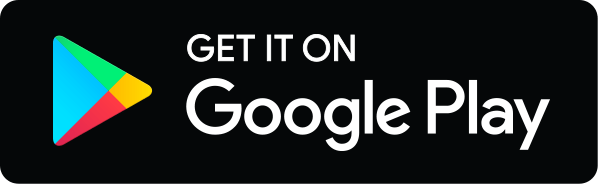