Treatment of Thyrotoxicosis
David S. Cooper
The ideal treatment of thyrotoxicosis would be directed at its cause. This is possible in only a few patients; e.g., those with exogenous thyrotoxicosis or thyrotropin (TSH)-secreting pituitary adenomas. In patients with thyrotoxicosis due to its more common causes, especially Graves’ disease, an autonomously functioning thyroid adenoma (toxic adenoma), and multinodular goiter, the fundamental causes are not known. Therapy is therefore directed at inhibiting thyroidal thyroxine (T4) and triiodothyronine (T3) synthesis and release or destroying thyroid tissue. Ancillary treatment involves ameliorating the effects of T4 and T3 on peripheral tissues. There are several means of accomplishing these goals, and their efficacy depends to some extent on the cause of the thyrotoxicosis. Because this is so, an attempt should be made to determine the cause; this usually can be achieved by history and physical examination, aided by selected tests such as measurement of thyroid radioiodine uptake and thyroid radionuclide imaging (see Chapters 12 and 31 and Chapters 17 through 23, in which the different causes of thyrotoxicosis are discussed).
This chapter considers the three major forms of treatment of thyrotoxicosis: Antithyroid drugs, radioactive iodine (radioiodine), and thyroidectomy. The emphasis is on the treatment of hyperthyroidism caused by Graves’ disease, because it is the most common cause of thyrotoxicosis, and it is the disorder in which the relative merits of different treatments are most vigorously debated. When appropriate, the treatment of some of the other causes of thyrotoxicosis and of special categories of patients (e.g., children, pregnant women, and patients with thyrotoxic storm) is mentioned. Information about treatment of some of the less common causes of the disorder can be found in the chapters addressing those disorders.
Thyrotoxicosis Caused By Graves’ Disease
Graves’ disease is an autoimmune disease characterized by thyrotoxicosis caused by thyroid hyperplasia and hypersecretion of hormones from the thyroid gland, and, less commonly, infiltrative ophthalmopathy and localized myxedema (1). The thyroid hypersecretion and hyperplasia are caused by TSH receptor–stimulating antibodies (TSHR-Abs), which are antibodies against the TSH receptor on the cell membrane of thyroid follicular cells that mimic the effects of TSH (see Chapter 18A).
Autoimmune diseases tend to wax and wane over time, and Graves’ disease is no exception. Although spontaneous remissions occur in patients who are not treated, Graves’ disease – or rather the thyrotoxicosis that results from it – is virtually always treated because spontaneous remissions in untreated patients are uncommon and because the resulting thyrotoxicosis can have deleterious effects on multiple organ systems. In addition, there are several safe and effective therapies from which to choose, although each has certain drawbacks. Which therapy is best is a matter of debate, and opinions vary from country to country and from continent to continent (2,3). There is no “best” treatment, and the choice depends on several factors. Among the most important are the physician’s experience and the patient’s preferences. In some situations (e.g., in pregnant women and elderly patients), the therapeutic choices are more limited.
The chief therapeutic objective is to alleviate the patient’s thyrotoxicosis. Antithyroid drugs act by decreasing thyroid hormone production. Whether the remissions that sometimes occur during or after antithyroid drug therapy are spontaneous, are due to amelioration of thyrotoxicosis, or are due to drug effects on the immune system is a matter of debate and is discussed later in detail. In contrast, surgery and radioiodine reduce the mass of thyroid tissue, but are not thought to alter the underlying Graves’ disease, except possibly by removing intrathyroidal lymphocytes, a source of TSHR-Ab. Hypothyroidism usually follows the latter two treatments, but also may occur during or after drug therapy (4,5), possibly because of autoimmune destruction of the thyroid gland (6,7). Thus, the end result may be the same, regardless of the form of therapy.
Drug Therapy of Thyrotoxicosis Caused By Graves’ Disease
Antithyroid Drugs
Antithyroid drugs have been a mainstay of treatment of patients with Graves’ thyrotoxicosis for almost 70 years (8,9). They can be given to patients with other forms of thyrotoxicosis (e.g., toxic nodular goiter), but they are not usually the primary mode of therapy for these conditions. These drugs inhibit the synthesis of T4 and T3, leading to gradual reduction in their serum concentrations. After several weeks or a few months, the dosage usually can be reduced; in some patients the drug can be discontinued, and the patient may remain euthyroid for months or years. A remission of Graves’ disease, usually defined as being euthyroid for at least 1 year after treatment was stopped, occurs in about one-half of the patients. Thereafter, some patients have recurrent thyrotoxicosis (10), but others never do (11) (Fig. 32.1).
![]() Figure 32.2 The structure of thiourea and two antithyroid drugs, propylthiouracil and methimazole, used clinically. |
The antithyroid drugs to be considered here are heterocyclic compounds known as thionamides that contain a thioureylene group (Fig. 32.2). Three drugs of this type are available: Methimazole [1-methyl 2-mercaptoimidazole;
MMI (Tapazole)], carbimazole (1-methyl-2-thio-3 carbethoxy-imidazole), and propylthiouracil (6-propyl-2-thiouracil; PTU). MMI and PTU are used in the United States and South America, MMI in Europe and Japan, and carbimazole mainly in the United Kingdom. Carbimazole is rapidly metabolized to MMI (12) and has no properties not shared by MMI; therefore, these two drugs can be considered as one.
MMI (Tapazole)], carbimazole (1-methyl-2-thio-3 carbethoxy-imidazole), and propylthiouracil (6-propyl-2-thiouracil; PTU). MMI and PTU are used in the United States and South America, MMI in Europe and Japan, and carbimazole mainly in the United Kingdom. Carbimazole is rapidly metabolized to MMI (12) and has no properties not shared by MMI; therefore, these two drugs can be considered as one.
The origin of antithyroid drugs dates back to the early 1940s, with the serendipitous observations of two groups working independently at the Johns Hopkins Medical School. Richter and Clisby, who were studying taste preferences in laboratory animals, noted that the bitter substance phenylthiocarbamide caused goiter in rats (13). The MacKenzies, who were studying the gut flora of guinea pigs, recognized that the nonabsorbable antibiotic sulfaguanidine also caused goiter (14). They (15) and Astwood (16) subsequently determined that the cause of the goiter was stimulation of the thyroid by the pituitary gland, consequent to pharmacologic inhibition of thyroid hormone production. Within 18 months after the observations that sulfaguanidine and thiourea caused goiter, Astwood proposed that goitrogens could be used to treat thyrotoxicosis, screened many potentially useful compounds using a bioassay system, and conducted clinical studies with thiourea and thiouracil. Indeed, he coined the term “antithyroid drug” (17).
Mechanism of Action
The antithyroid drugs have intrathyroidal and extrathyroidal actions. The chief intrathyroidal actions are inhibition of iodine oxidation and organification (the iodination of tyrosine residues in thyroglobulin), inhibition of iodotyrosine coupling, possible alteration of the structure of thyroglobulin, and possible inhibition of thyroglobulin biosynthesis. The main extrathyroidal action is inhibition of conversion of T4 to T3 (by PTU, but not MMI). The drugs’ immunosuppressive actions, if they exist, could be intrathyroidal or extrathyroidal.
Intrathyroidal Actions
Antithyroid drugs are actively transported into the thyroid gland (18,19,20), by a mechanism that is similar but probably not identical to the iodide transport system (21). They do not inhibit iodide transport or block the release of stored T4 and T3. Their most important actions are to interfere with thyroid peroxidase–mediated iodide oxidation, organification of iodine, and iodotyrosine coupling. With respect to the organification of iodine, the drugs compete with tyrosyl residues in thyroglobulin for oxidized iodine (22,23). As a result, the active iodine species is diverted away from tyrosyl residues in thyroglobulin, so that fewer tyrosyl residues are iodinated. The drugs themselves ultimately are oxidized and degraded. Antithyroid drugs also interfere with the peroxidase-catalyzed coupling process by which iodotyrosyl residues are coupled to form T4 and T3; the drug concentrations required to inhibit coupling are less than those required to inhibit iodine organification (24).
Extrathyroidal Actions
Propylthiouracil, but not MMI, decreases the conversion of T4 to T3 in peripheral tissues (and the thyroid) by inhibiting the activity of type 1 T4-deiodinase (see Chapter 7.) The mechanism is uncertain but may involve competition between the drug and cofactors for the reaction, which include reduced sulfhydryl groups (25). In addition, PTU may bind covalently to the enzyme via a selenosulfide bond (26), thereby inactivating it (27). MMI is not an inhibitor of this reaction, but other imidazole derivatives do inhibit T4 conversion to T3 in vitro (28). The clinical importance of the ability of PTU to block this conversion is discussed later.
Effects on the Immune System
Methimazole and PTU may have immunosuppressive as well as antithyroid actions (29,30,31). The central question is whether the effects are caused by the action of the drugs on the immune system, or whether the abatement of autoimmune phenomena is simply the result of the decline in thyroid secretion induced by the drugs. In studies in vitro, the observed activity can be directly ascribed to the drug, but in studies in vivo the distinction between direct and indirect effects is ambiguous.
Despite some negative results (32), numerous in vitro studies have documented an effect of antithyroid drugs on various arms of the immune system. The drugs inhibit lymphocyte transformation (33), and they may have other inhibitory (34,35,36) [as well as stimulatory (34,35,36)] effects on lymphocyte, monocyte (34), and neutrophil (34,37) function and on formation of soluble mediators such as interleukin-2 (IL-2) (38). The formation of free radicals, which may be important in T-cell responsiveness and in complement-mediated thyroid-cell injury, may be inhibited by MMI (39). In addition, the drugs may reduce expression of major histocompatibility complex (MHC) class II (HLA-DR) molecules on thyroid cells, which may be important for the initiation or maintenance of Graves’ disease (40). Antithyroid drugs may reduce HLA-DR expression directly (41) or by inhibiting secretion of interferon-γ, which induces class II molecules (42). MMI reduces MHC class I messenger RNA concentrations in cultured thyroid cells (FRTL-5 cells) (43). Finally, antithyroid drugs may influence the immune system by inducing expression of Fas ligand (FasL) on thyroid cells, which could lead to activation of Fas on lymphocytes and consequently Fas-induced apoptosis of these cells (44).
There is also strong in vivo evidence, albeit circumstantial, for an immunologic effect of antithyroid drugs. The thyroid glands of patients with hyperthyroidism who were treated with an antithyroid drug before thyroidectomy were depleted of lymphocytes, as compared with patients who had received only the beta-adrenergic receptor antagonist propranolol (45). In addition, the serum concentrations of TSHR-Ab, whether measured by bioassay or receptor assay, and other antithyroid antibodies decline during antithyroid drug therapy (46,47). The effects appear to be specific for thyroid-related antibodies, because the serum concentrations of antiparietal cell antibodies did not change in patients with coexisting autoimmune gastritis (48). Serum concentrations of the immunomodulator intercellular adhesion molecule-1 (ICAM-1) (49), and of some cytokines and soluble cytokine receptors, also decrease in response to antithyroid drug therapy, including those of IL-1β (50), soluble IL-2 receptors (51,52), and soluble IL-6 receptors (53). A study of thyroid aspirates from treated versus untreated patients suggested that thyrocyte HLA-DR expression is reduced by thionamide therapy (54). Furthermore, antithyroid drug treatment results in changes in cell-mediated immunity in patients with Graves’ disease. For example, an increase (normalization) in suppressor T-cell number during treatment was found in several (50,55,56), but not all (57) studies, and helper T-cell (50) and natural killer cell activity decreased (58). Also, MMI decreases the number of activated T cells within the thyroid itself, as compared with the pretreatment number (50).
Despite the evidence for an immunomodulatory effect of antithyroid drugs, several caveats are necessary. With regard to the in vitro data, the effective doses have varied from 10-4 to 10-5 mol/L, whereas intrathyroidal concentrations in vivo are unlikely to exceed 5 × 10-5 mol/L (59), thus casting some doubt on the pharmacologic relevance of the observed effects. The changes in serum autoantibody concentrations and in T-cell subsets do not occur in all patients, and the changes that do occur are variable. The reasons for this are unclear, but they must relate to the question of whether remissions of Graves’ disease are spontaneous, or whether they are induced by the antithyroid drug (see later discussion). Finally, any changes in immune response markers that may be induced by antithyroid drugs inevitably occur when thyroid secretion is declining and thyrotoxicosis is improving (29). Thus, if the thyrotoxic state was responsible for perpetuation of the altered immunity, then its correction should reduce the alterations. Therapy for Graves’ thyrotoxicosis with potassium perchlorate leads to a decline in serum TSHR-Ab concentrations in a manner similar to that which occurs during antithyroid drug treatment (60), but since perchlorate also may have immunosuppressive effects (61), the matter remains unresolved.
Despite these reservations, additional in vivo data indicate an immunosuppressive effect of antithyroid drugs. First, administration of MMI causes serologic and histologic attenuation of experimental autoimmune thyroiditis in rats (62,63,64). Second, in one study of euthyroid patients with chronic autoimmune (Hashimoto’s) thyroiditis, administration of carbimazole caused a decline in serum antithyroid peroxidase antibody concentrations (47), a result not confirmed, however, by two other studies (65,66). Methimazole therapy, but not glucocorticoids, given prior to radioiodine therapy blunted the increase in TSHR-Ab usually seen after therapy (67,68) (see later discussion), suggesting that an organ-specific effect, rather than generalized immunosuppression, is of primary importance (68). In another study, patients treated with either PTU or carbimazole had identical decrements in serum thyroid hormone concentrations, but the carbimazole-treated patients had greater decreases in serum TSHR-Ab concentrations and increases in the number of suppressor T cells, suggesting, indirectly, an effect on the immune system independent of thyroid function (69).
To summarize, antithyroid drugs can inhibit immune function in vitro, but the concentrations of drug required may be higher than are attained within the thyroid gland during treatment. Changes in serum concentrations of antithyroid antibodies and TSHR-Ab and in T-cell subsets occur in patients receiving chronic antithyroid drug therapy, but changes in thyroid function occur concomitantly, making it impossible to distinguish cause and effect satisfactorily.
Table 32.1 Selected Pharmacologic Features of Antithyroid Drugs | ||||||||||||||||||||||||||||||
---|---|---|---|---|---|---|---|---|---|---|---|---|---|---|---|---|---|---|---|---|---|---|---|---|---|---|---|---|---|---|
|
Clinical Pharmacology of the Antithyroid Drugs
Methimazole
MMI is almost completely absorbed from the gastrointestinal tract (70,71). Peak serum concentrations occur 1 to 2 hours after ingestion and are in the range of 300 ng/mL (2.6 mmol/L)
after a 15 mg oral dose (Table 32.1) (71). The serum concentrations are dose related and correlate with effects on iodine organification (72). Carbimazole is rapidly converted to MMI in serum: 10 mg of carbimazole yields about 6 mg MMI (12). The serum half-life of MMI is 6 to 8 hours, but little is bound to serum proteins (71,73). The serum half-life is similar in patients with thyrotoxicosis (71,73), but it may be shorter in patients who do not respond to the drug (74). Drug clearance is unchanged in patients with renal disease (70) but is slowed in those with hepatic disease (73).
after a 15 mg oral dose (Table 32.1) (71). The serum concentrations are dose related and correlate with effects on iodine organification (72). Carbimazole is rapidly converted to MMI in serum: 10 mg of carbimazole yields about 6 mg MMI (12). The serum half-life of MMI is 6 to 8 hours, but little is bound to serum proteins (71,73). The serum half-life is similar in patients with thyrotoxicosis (71,73), but it may be shorter in patients who do not respond to the drug (74). Drug clearance is unchanged in patients with renal disease (70) but is slowed in those with hepatic disease (73).
Intrathyroidal MMI concentrations are about 500 to 2,000 ng/g (about 5 × 10-5 mol/L) (59,75). The intrathyroidal turnover of MMI is slow, the concentrations 17 to 20 hours after ingestion being similar to those 3 to 6 hours after ingestion (75), which may account for the longer duration of action of MMI as compared with PTU. The effects of MMI dissipated within 24 hours in one study (76), but other studies suggest a longer duration of action (77). Little MMI is excreted in the urine, and neither the products of metabolism nor their fate is known (78). Because it is not protein bound (73) and is lipid soluble, MMI freely crosses membranes [e.g., placenta (79) and breast epithelium (73)]. Given its relatively long serum (and intrathyroidal) half-life and its long duration of action, MMI is effective when given as a single daily dose (80,81,82,83).
Although the potency of MMI is commonly regarded as being about 10 times that of PTU, it is almost surely greater, and it may be up to 50 times more potent (72). Indeed, thyrotoxicosis can be controlled in most patients with doses of MMI that are less, e.g., 10 to 15 mg daily, than those traditionally thought to be necessary (82,83). The difference in potency between MMI and PTU is probably not due to an actual difference at the biochemical level, but rather to differences in uptake into and metabolism within the thyroid gland, because in vitro MMI is not a significantly more potent inhibitor of thyroid peroxidase-catalyzed reactions (23). One study found an increase in prednisolone clearance in patients taking MMI, possibly related to hepatic enzyme induction (84). Therefore, patients requiring glucocorticoid therapy for Graves’ ophthalmopathy may need higher doses if they are also taking MMI.
Propylthiouracil
Orally administered PTU is almost completely absorbed. Peak serum concentrations occur about 1 hour after ingestion and are dose dependent, with peak concentrations of about 3 mg/mL (18 mmol/L) after a 150 mg oral dose (85) (Table 32.1). Serum PTU concentrations correlate with the drug’s effects on iodine oxidation and organification and with inhibition of T4-deiodinase activity (85). There is little information about intrathyroidal concentrations, which are most relevant to efficacy and duration of action (20). The serum half-life of PTU is in the range of 1 to 2 hours, and it is not altered in patients with thyrotoxicosis (85) or hepatic (86) or renal failure (87) in children (88) or in elderly patients (89). PTU is strongly (80% to 90%) protein-bound (90), largely to serum albumin (91), and is ionized at physiologic pH (92). This has implications for PTU therapy in pregnant and lactating women (see later discussion), because free (i.e., unbound) drug concentrations are low and ionized drug may not freely cross membranes. Most of an ingested dose of PTU is excreted in the urine, after conjugation with glucuronide in the liver (20).
The duration of action of PTU is about 12 to 24 hours (77,93). This rate probably depends on several factors, including the rates at which the drug is concentrated and degraded within the thyroid. Clearly, the duration of action is longer than the serum half-life. Although PTU can sometimes be given satisfactorily as a single daily dose (94), it usually is given every 6 to 8 hours (95), at least when therapy is initiated. With time, the frequency and total daily dose often can be decreased (93).
Clinical Considerations in the Use of Antithyroid Drugs
The thionamide antithyroid drugs are chiefly used for the long-term treatment of patients with thyrotoxicosis caused by Graves’ disease, with the expectation – or at least the hope – that a remission of Graves’ disease will occur. In surveys of thyroidologists in the United States over a decade ago, radioiodine, not an antithyroid drug, was the preferred treatment for most patients, the exceptions being children, adolescents, and young adults (2,3). In contrast, an antithyroid drug is the treatment of choice in much of the rest of the world, including Europe, Japan, and South America (2).
The clinical factors that influence the choice of therapy and the likelihood of remission are discussed later. Antithyroid drugs rather than surgery are preferred for pregnant women, discussed separately, and for children and adolescents (also discussed later). They are often given before surgery and
sometimes before radioiodine therapy, and they are standard therapy for neonates with Graves’ disease, which is a transient condition.
sometimes before radioiodine therapy, and they are standard therapy for neonates with Graves’ disease, which is a transient condition.
Both MMI and PTU are very (at least 90%) effective in controlling thyrotoxicosis due to Graves’ disease, and to some extent the choice between the two drugs has been a matter of personal preference. Recently, however, concerns about PTU-related hepatotoxicity have led the American Thyroid Association (96) and the FDA (97) to recommend that MMI be used instead of PTU as first line therapy, unless the patient has a minor allergy to MMI, and refuses alternate therapy with radioiodine or surgery. The situation in pregnancy is discussed later. While PTU inhibits extrathyroidal T4 conversion to T3, and although serum T3 concentrations do initially decline more rapidly after the initiation of PTU therapy, there is no evidence that the more rapid decline is clinically important, except possibly in patients with severe or life-threatening thyrotoxicosis (thyrotoxic storm) (98,99). In fact, MMI therapy results in more rapid normalization of serum T4 and T3 concentrations than does PTU therapy (100), and more effective long-term control of T3 levels in severe thyrotoxicosis (101) [Generic MMI is available in either 5, 10, or 20 mg tablets (Tapazole® is only available as 5 and 10 mg tablets)], and PTU in 50 mg tablets, a difference in formulation that means that fewer tablets of MMI need be given each day. The usual starting dose of MMI has been 20 to 30 mg daily, often in divided doses, but once-daily dosing – and lower doses – are adequate for most patients (102). In a prospective multicenter trial 10 mg daily was nearly as effective as 40 mg daily (103); serum T4 and T3 concentrations were normal in 6 weeks in 85% of the patients given 10 mg daily and 92% of those given 40 mg daily. Patients living in areas of relative iodine deficiency had a more rapid response, an effect noted previously (104). There is little additional benefit of even higher doses (105). The usual starting dose of PTU is 100 mg three times daily.
One study found that baseline thyroid function is an important predictor of the required starting dosage. If the initial serum T4 concentration was above 20 μg/dL (260 nmol/L), a daily carbimazole dose of 20 mg (equal to about 15 mg MMI) was inadequate for many patients; in contrast, if the initial serum T4 concentration was lower, a starting dose of 40 mg/day (equivalent to 30 mg MMI), caused hypothyroidism in a substantial number of patients (Fig. 32.3) (106). Clearly, the dose should be increased if thyroid secretion does not decrease within 4 to 6 weeks. Doses of PTU as high as 2,000 mg daily have been given to patients thought to be resistant to the drug, but in most instances the problem was poor compliance (107). In seriously ill patients who do not respond to high doses of antithyroid drugs, the addition of glucocorticoid therapy may provide additional benefit (108).
As thyroid secretion decreases during the first several weeks or months after antithyroid therapy is initiated, the dose of drug should be decreased, e.g., to 5 or even 2.5 mg MMI or 100 or 50 mg PTU daily, or hypothyroidism may supervene. Other factors that determine the speed of recovery include disease activity, the initial degree of thyroid hypersecretion, and the intrathyroidal stores of T4 and T3. The ability to reduce the dose without exacerbation of thyrotoxicosis reflects not only waning of disease activity, possibly reflected by a decline in TSHR-Ab production, but also because the goal of therapy changes, from relatively complete to partial inhibition of T4 and T3 synthesis.
If high doses of drug are required for control of thyrotoxicosis, remission is unlikely, and ablative therapy usually is selected. Some authors have argued that continuous high-dose antithyroid drug therapy is preferable to reducing the dose to maintain thyroid function within normal limits, because rates of remission may be higher as a result of greater putative immunosuppressive effects. However, high-dose therapy has not been widely used because this theory is unproven, it requires concomitant T4 therapy to prevent iatrogenic hypothyroidism, and because the frequency of serious side effects is higher with high doses of antithyroid drug (103,109).
There are many reasons to prefer MMI (110). First, the likelihood of compliance is higher because MMI can be given once daily and fewer tablets per day are needed; once-daily dosing of PTU is less effective (94,95). Second, patients treated with MMI become euthyroid sooner (100,101,111,112). The costs of MMI and PTU are comparable when doses of MMI in the 10 to 20 mg/day range are given; at higher doses, MMI may be more expensive than PTU. Finally, MMI is safer than PTU (113), at least in terms of the most important major side effects of these drugs, which are agranulocytosis and hepatotoxicity (see later discussion). In some special circumstances – pregnancy and thyrotoxic storm – PTU may be preferable, as discussed below. Indeed, sales of PTU in the United States have
declined sharply over the last decade, with a reciprocal rise in methimazole prescriptions (114).
declined sharply over the last decade, with a reciprocal rise in methimazole prescriptions (114).
Table 32.2 Side Effects of Antithyroid Drugs | ||
---|---|---|
|
Side Effects of Antithyroid Drugs
Antithyroid drugs have multiple potential side effects (Table 32.2). Most are considered to be allergic reactions. Fever, urticaria or other rashes, and arthralgia occur in 1% to 5% of patients (115,116), usually within the first several weeks or months after initiation of therapy, and are more common in patients treated with higher doses (103,116,117,118). In one study, serum aminotransferase concentrations increased slightly in one-third of patients within 2 months after starting PTU therapy (118). The results of baseline liver function tests, which are often abnormal in hyperthyroidism (119,120), were not predictive of this change in PTU-treated patients (118), and the high serum aminotransferase values resolved without discontinuation of therapy. Similar changes have not been reported for MMI. Serum bone alkaline phosphatase concentrations also may increase transiently during antithyroid drug therapy, and should not be confused with hepatobiliary dysfunction, but rather is a manifestation of an increase in bone formation (120). Periodic monitoring of liver function tests is generally not recommended because of its fulminant onset and because of the lack of evidence that monitoring prevents severe hepatotoxicity with other hepatotoxic drugs (96).
The more serious and rarer toxic reactions (major side effects) are agranulocytosis, aplastic anemia, hepatitis [with PTU (121)], and cholestasis [with MMI (121)], polyarthritis (122), and a lupus-like syndrome or vasculitis (123,124), all of which, with the possible exception of agranulocytosis, are more common in patients treated with PTU. Agranulocytosis, the most feared problem, probably occurs with equal frequency with both drugs (about 0.2% to 0.5%), although one report suggested that it was more often seen with PTU (125); the other severe reactions are less common.
Agranulocytosis
The cause of MMI- or PTU-induced agranulocytosis is not known, but it may be an immunologic phenomenon. Some patients have evidence of lymphocyte sensitization (126), an association with certain HLA class II haplotypes has been reported (127), and some patients have antibodies to granulocytes and granulocytic progenitor cells (128). The frequency of agranulocytosis is dose-related, especially with methimazole (115,129), and may be more common with PTU usage (125). Elderly patients may be more susceptible to agranulocytosis (115,130).
Agranulocytosis, which is often defined as a granulocyte count less than 250 cells/mm3 (0.25 × 109/L), usually develops so suddenly that routine monitoring of the leukocyte count has been thought to be of little value. However, in one study granulocytopenia [granulocyte count less than 500/mm3 (0.5 × 109/L)] was detected by routine monitoring of leukocyte counts before agranulocytosis occurred, suggesting that the onset may be gradual (130). In the patients who had granulocytopenia, prompt discontinuation of therapy led to an increase in leukocyte count. If this observation is confirmed, periodic leukocyte counts would be reasonable, at least during the first few months of therapy, when most reported cases of agranulocytosis have occurred. However, due to the low frequency of this side effect, routine monitoring is not recommended (96). Patients with agranulocytosis typically present with fever and evidence of infection, usually of the oropharynx, and sepsis with gram-negative organisms can occur (131). All patients should be warned of the possible symptoms, and given written instructions that the drug should be discontinued and a physician contacted immediately if they have any symptoms of infection. Agranulocytosis must be distinguished from the transient, mild granulocytopenia [granulocyte count <1,500/mm3 (1.5 × 109/L)] that occurs in up to 10% of antithyroid drug–treated patients, as well as that occasionally present in patients with thyrotoxicosis before therapy or in normal black subjects. As a practical matter, complete leukocyte counts should be obtained before initiation of therapy to establish a baseline. Although monitoring is not recommended, if a subsequent count is <1,500/mm3 (1.5 × 109/L), the drug should be discontinued. If the drug is not discontinued, the leukocyte count should be repeated weekly until it is stable or increasing. It may be possible to distinguish those patients with granulocytopenia during therapy who will recover rapidly from those who are likely to have agranulocytosis. In one study, 25 of 28 patients (89%) with moderate granulocytopenia [granulocyte count 500 to 1,000/mm3 [0.5 to 1.0 × 109/L)] and 4 of 6 patients (67%) with more severe granulocytopenia [granulocyte count 100 to 500/mm3 [0.1 to 0.5 × 109/L)] had a normal granulocyte count 4 hours after a single injection of 75 mg of granulocyte colony stimulating factor (G-CSF) and subsequently recovered fully, whereas those patients who did not have a normal granulocyte count after G-CSF injection had progressive decreases in granulocyte counts (132). Thus, testing with G-CSF may allow recognition of those patients who will recover from those who will require additional care.
In addition to prompt discontinuation of the antithyroid drug, treatment of agranulocytosis typically involves the
administration of broad-spectrum antibiotics and appropriate supportive measures; hospitalization should be avoided if possible, but is essential if the patient is febrile. The granulocyte count usually begins to increase within several days, but may not be normal for 10 to 14 days. G-CSF therapy has proven variably effective. Retrospective data suggest modest efficacy (133) in shortening the recovery time, but in a randomized trial of 24 patients, the combination of G-CSF (100 to 250 mg) and antibiotic therapy did not shorten the duration of agranulocytosis, as compared with antibiotic therapy alone (134). However, G-CSF may accelerate recovery in patients in whom the ratio of granulocytes to erythrocytes in the bone marrow is 0.5 or higher (135). Glucocorticoid therapy is probably ineffective (135). Other very rare hematologic side effects include aplastic anemia (136), as well as hypoprothrombinemia (137,138)
administration of broad-spectrum antibiotics and appropriate supportive measures; hospitalization should be avoided if possible, but is essential if the patient is febrile. The granulocyte count usually begins to increase within several days, but may not be normal for 10 to 14 days. G-CSF therapy has proven variably effective. Retrospective data suggest modest efficacy (133) in shortening the recovery time, but in a randomized trial of 24 patients, the combination of G-CSF (100 to 250 mg) and antibiotic therapy did not shorten the duration of agranulocytosis, as compared with antibiotic therapy alone (134). However, G-CSF may accelerate recovery in patients in whom the ratio of granulocytes to erythrocytes in the bone marrow is 0.5 or higher (135). Glucocorticoid therapy is probably ineffective (135). Other very rare hematologic side effects include aplastic anemia (136), as well as hypoprothrombinemia (137,138)
Hepatotoxicity
Fulminant PTU-induced hepatitis is rare (estimated to occur in 1:10,000 PTU-exposed patients, and may be more common in children (139); deaths have been reported, and other patients have required liver transplantation (139,140). A review of the literature found that the average PTU dose associated with liver failure was 300 mg/day, and the median time to develop severe hepatotoxicity after starting PTU was 120 days, with a range of 1 to 450 days (139).
MMI also can cause liver disease, usually cholestatic hepatitis; it may be severe and slow to resolve after the drug is stopped (121,141,142). Patients should be warned about the potential for hepatotoxicity, and to discontinue the drug if they have malaise, jaundice, or dark urine. PTU should be discontinued immediately if transaminases are >two- to threefold above normal and fail to improve on testing 1 week later. If transaminase levels worsen, immediate referral to a gastroenterologist or hepatologist is recommended.
Vasculitis
Patients with the lupus-like syndrome or vasculitis associated with PTU (and rarely MMI) may have fever, arthralgias, skin involvement (e.g., purpura, leg ulcers, bullae, erythema nodosum), serositis, glomerulonephritis, or pulmonary hemorrhage (124), and often have high serum concentrations of anti-neutrophil cytoplasmic antibodies (ANCA) (123,124,143). Reports of ANCA-related vasculitis, mostly with PTU, are predominantly from Asian countries; some patients are ANCA-positive before treatment is started (144,145). Between 30% and 60% of patients develop ANCA with PTU therapy, but do not have clinical vasculitis (145,146). In most cases, the antibody is myeloperoxidase-ANCA (MPO-ANCA) (144,146,147). In a small prospective study in which patients with Graves’ disease were negative for ANCA at baseline, asymptomatic ANCA positivity developed in 32% of patients treated with PTU after a mean of 12 months of therapy (148), and tended to resolve after cessation of treatment (148). The clinical significance of asymptomatic ANCA positivity is not known. In patients with symptomatic drug-induced lupus or vasculitis, treatment with glucocorticoids is usually successful, although in severe cases other immunosuppressants such as cyclophosphamide may be required (124).
Other Considerations
Like the minor side effects, these major side effects usually occur within the first several weeks or months after the initiation of therapy, when drug dosage is higher. However, they can occur during prolonged treatment (109) and may be more common when the drug is resumed than when it was first given (40). In the case of minor drug-related side effects such as fever or rash, the reaction may subside in several days despite continuation of therapy, with or without a short course of antihistamine therapy. If the minor side effect persists, the other antithyroid drug could be substituted, with an approximate 50% possibility that the side effect will subside. Substitution should not be attempted in the case of agranulocytosis because cross-reactivity has been reported. There are reports of methimazole being used safely in patients with PTU-induced hepatotoxicity, presumably because the two drugs cause different types of hepatic injury (149).
Patients with major side effects in whom antithyroid drug therapy is discontinued usually become thyrotoxic soon thereafter, if they were not thyrotoxic when the drug was discontinued. In most of them, antithyroid drug therapy is no longer an option. They should be treated with a beta-adrenergic antagonist drug and inorganic iodine, an iodinated oral cholecystographic agent if available, or lithium if the thyrotoxicosis is severe. If it is not, radioiodine therapy should be given as soon as practicable.
Other rare side effects of MMI are pancreatitis (150); hypoglycemia, caused by anti-insulin antibodies (the “insulin-autoimmune syndrome” or “Hirata disease”), typically in Japanese patients (151,152); and myalgia and high serum creatine kinase concentrations (153). MMI can cause a decreased sense of taste (115), whereas PTU may cause a bitter or metallic taste.
Follow-up of Patients Taking an Antithyroid Drug for Graves’ Disease
Once antithyroid drug therapy has been initiated, patients should be seen every 6 to 8 weeks until they are clinically and biochemically euthyroid. This usually occurs within 4 to 6 weeks with MMI, but it may take up to 12 weeks with PTU (100,111). As the thyrotoxicosis comes under control, the dose of antithyroid drug should be progressively reduced. Hypothyroidism, thyroid enlargement, or both may occur in patients if the dosage of drug is not decreased. Later, the frequency of follow-up visits can be decreased to every 2 to 3 months and then every 6 months.
The usual biochemical tests of thyroid function may be misleading early in the course of antithyroid drug therapy. TSH secretion is strongly inhibited by thyrotoxicosis, and therefore serum TSH concentrations may remain low for several months despite normalization of serum T4 and T3 concentrations. Some patients remain thyrotoxic with persistently high serum T3 concentrations, despite normal or even low serum T4 concentrations, indicating the need for an increase, rather than a decrease, in antithyroid drug dosage (154,155). This syndrome of so-called T3-predominant thyrotoxicosis is due to incomplete inhibition of thyroid hormone synthesis and may be associated with a low likelihood of remission (156). Although an enlarging thyroid gland may indicate that hypothyroidism has developed, it also may be indicative of persistent or increasing TSHR-Ab production and a low likelihood of remission.
Remissions and Antithyroid Drug Therapy
The primary goal of antithyroid drug therapy is to render the patient euthyroid. However, this form of treatment is usually chosen in anticipation that the patient will eventually have a remission of Graves’ disease, and therefore will not need destructive therapy. Unfortunately, the ability to predict which patients are likely to have a remission is poor (157), and there have been only a few large studies of the possible clinical, biochemical, and pharmacologic features that correlate with remission or relapse (158,159,160). In the United States, overall, the remission rate is in the 20% to 30% range, but may be as high as 80% in subgroups of patients with milder disease. One way of organizing the often-conflicting information concerning the factors that might be related to remission is to distinguish the clinical or patient-related factors from the drug-related factors, e.g., type or dose of drug or duration of therapy. The goal is to identify those patients in whom remission is unlikely, so that they are not given treatment destined to fail.
Pretreatment Clinical Factors Relating to Remission of Graves’ Disease
Certain pretreatment clinical characteristics seem to be associated with a low likelihood of long-term remission (i.e., the patient remains euthyroid for at least 1 year after antithyroid drug therapy is discontinued). They include a large goiter and more severe biochemical thyrotoxicosis, in both adults (Fig. 32.4) (158,159,160,161) and children (162). Another may be a high ratio of T3 to T4 in serum [T3 (ng/dL): T4 (μg/dL) >20) before (and during) therapy (156), but this is disputed (163). High baseline serum concentrations of TSHR-Ab may (159,164) or may not (158) be predictive of eventual relapse, but an undetectable serum TSHR-Ab concentration at the time of diagnosis is associated with a very high (90%) rate of remission (165). Patients with allergic diseases (e.g., atopy, allergic rhinitis, asthma), and high serum IgE concentrations may be less likely to have a remission (166).
Factors not consistently related to the likelihood of remission are the sex of the patient (167), smoking (168,169), the presence of ophthalmopathy (170), or the duration of symptoms before the initiation of therapy. The remission rate may be higher in older patients, possibly due to milder thyrotoxicosis (171), but no relationship with age was found in another study (158). One study found that certain personality traits (e.g., hypochondriasis, depression, paranoia), as well as a higher prevalence of “daily hassles,” were more common in those who relapsed versus those who remained euthyroid (172). Unfortunately, the low predictive value of any clinical finding makes it difficult to know a priori which patients are likely to have a remission and which are not.
Therapy Factors Relating to Remission of Graves’ Disease
Duration of Therapy
Although most patients are treated with an antithyroid drug for 1 to 2 years before it is discontinued (2), some patients have been reported to remit after only weeks or months of therapy (173,174). Longer courses of therapy intuitively seem preferable, and there are convincing retrospective data (175), particularly in children (176), that the longer the drug is given, the more likely the patient is to have a remission. However, prospective randomized studies on this point are conflicting (Fig. 32.5). In a study of 114 patients, 62% of those treated with MMI for 6 months relapsed, as compared with 42% of those treated for 18 months (177). However, in two other similarly designed studies, the relapse rates were similar among patients treated for 12 months and 24 months (178), and among patients treated for 18 and 42 months (179). Furthermore, among 100 patients given a “block-replace” regimen of
carbimazole plus T4, the 1-year relapse rates were 41% in those treated for 6 months and 35% in those treated for 1 year (180). In a prospective study of pediatric Graves’ disease, longer duration of therapy (up to 24 months) was also associated with the likelihood of remission (181). Given these results, treatment for 12 to 24 months seems reasonable, at which time treatment should be discontinued and the patient followed periodically.
carbimazole plus T4, the 1-year relapse rates were 41% in those treated for 6 months and 35% in those treated for 1 year (180). In a prospective study of pediatric Graves’ disease, longer duration of therapy (up to 24 months) was also associated with the likelihood of remission (181). Given these results, treatment for 12 to 24 months seems reasonable, at which time treatment should be discontinued and the patient followed periodically.
Drug Dose
The possibility that high-dose antithyroid drug therapy might increase the likelihood of remission is based on the suggestion that the drugs have immunosuppressive effects, as discussed earlier. One study did find that the remission rate was higher (75% vs. 42%) with high-dose therapy (mean MMI dose 60 mg/day or PTU dose 700 mg/day), as compared with lower doses, but the study was confounded by the fact that the high-dose therapy group also received T3 (182). In a subsequent study by the same group in which the patients given both high and low doses of antithyroid drug were given T3, the rates of remission were similar (183), and they were similar in other prospective randomized studies in which high and low doses were compared (160,184,185,186). Given the lack of evidence that high-dose therapy is more likely to be followed by remission and the higher likelihood of side effects (103,182,184,186), high-dose therapy cannot be recommended.
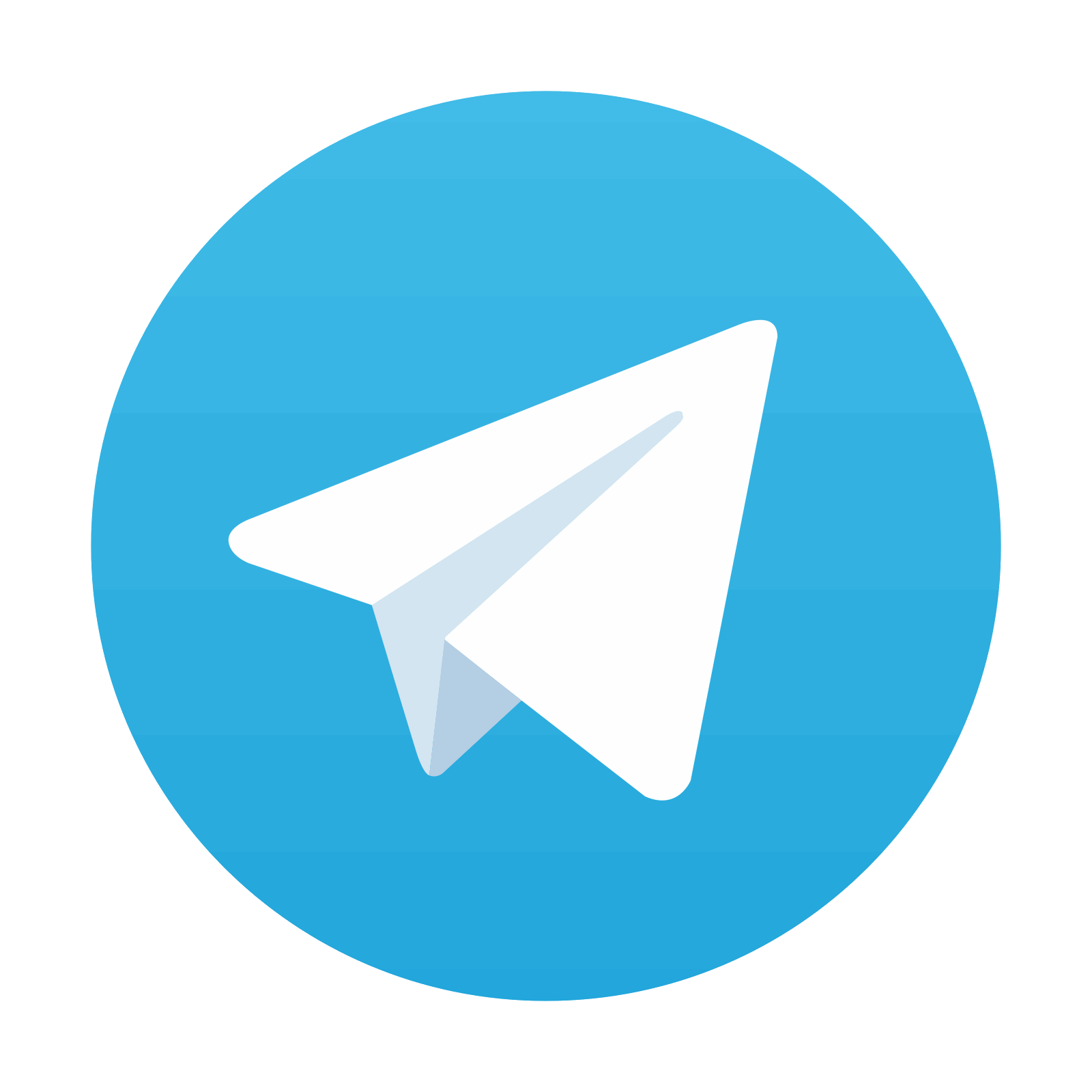
Stay updated, free articles. Join our Telegram channel

Full access? Get Clinical Tree
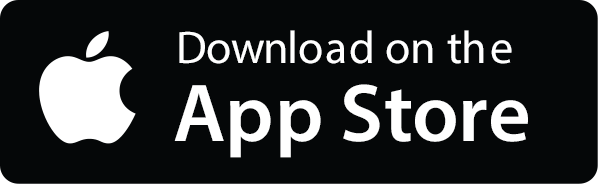
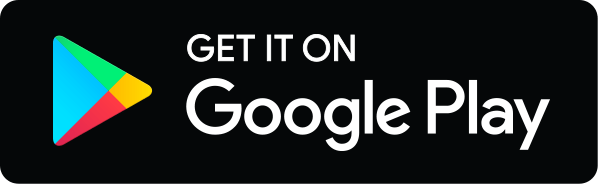
