div class=”ChapterContextInformation”>
4. The Thyroid Gland
4.1 Anatomy, Histology, and Physiology
The thyroid gland is the largest single organ specialized for endocrine hormone production. Its function is to secrete an appropriate amount of the thyroid hormones, primarily 3,5,3′,5′-1-tetraiodothyronine (thyroxine, T4; the thyroid is the only source of T4.) and a lesser quantity of 3,5,3′-1-triiodothyronine (T3; the thyroid secretes 20% of circulating T3; the remainder is generated in extraglandular tissues by the conversion of T4 to T3 by deiodinases).
A midline isthmus lying horizontally just below the cricoid cartilage.
Two lateral lobes that extend upward over the lower half of the thyroid cartilage.
The thyroid gland has a rich blood supply. Microscopically, thyrocytes form follicles which contain the colloid and are surrounded by a single layer of thyroid epithelium. The follicular cells of the thyroid gland are specialized in their ability to synthesize the large hormonal precursor protein thyroglobulin (Tg), concentrate iodide intracellularly from the circulation, and express a receptor that binds thyroid-stimulating hormone (thyrotropin, TSH), which promotes thyrocyte’s growth and biosynthetic functions. Thyroglobulin (=large glycoprotein containing 140 tyrosyl residues), synthesized by the follicle cells, is extruded into the lumen of the follicle. The biosynthesis of T4, T3 occurs within Tg at the cell-colloid interface.
The parafollicular C cells are the source of calcitonin and give rise to medullary thyroid carcinoma when they undergo malignant transformation.
4.2 Thyroid Hormone Synthesis and Secretion
Iodide is the main component of thyroid hormones and a sufficient iodide intake is necessary to allow the synthesis of thyroid hormones.
- 1.
Active transport of iodide across the basement membrane into the thyroid cell (trapping of iodide)
Iodide is transported across thyrocytes basal membrane by the NIS (Na+/I− symporter). Membrane-bound NIS, which derives its energy from a Na+-K+ ATPase, allows the human thyroid gland to maintain a concentration of free iodide 30–40 times higher than that in plasma. NIS action is stimulated physiologically by TSH and pathophysiologically by the TSH receptor-stimulating antibody of Graves’ disease.
Large amounts of iodide suppress both NIS activity and NIS gene expression, representing mechanisms of iodine autoregulation. The perchlorate ion (ClO4−) competes with iodide for NIS; perchlorate has been used to treat hyperthyroidism and has the potential to be an environmental inhibitor of thyroid function. NIS can also concentrate pertechnetate (TcO4−) into thyroid cells, facilitating use of the radionuclide sodium pertechnetate (99mTcO4−) for visualization of the thyroid gland and quantification of its trapping activity.
- 2.
Oxidation of iodide and iodination of tyrosyl residues in thyroglobulin to form iodotyrosine (MIT, DIT) (iodine organification)
Within the thyroid cell, at the apical-colloid interface, iodide is rapidly oxidized by locally produced hydrogen peroxide in a reaction catalyzed by TPO; the resulting active iodide intermediate is bound to tyrosyl residues in Tg. The coupling of iodotyrosyl residues in Tg is also catalyzed by TPO and form iodotyrosines [monoiodotyrosine (MIT) and diiodotyrosine (DIT)].
- 3.
Coupling of iodotyrosine molecules within Tg to form iodothyronines (T3,T4)
Within the Tg molecule, two molecules of DIT couple to form T4, and MIT and DIT molecules couple to form T3; this process is also mediated by TPO.
Note: Thiocarbamide drugs (particularly propylthiouracil, methimazole, carbimazole) are potent inhibitors of TPO and will block thyroid hormone synthesis.
- 4.
Proteolysis of thyroglobulin and thyroid hormone secretion
At the thyrocyte’s apical membrane, colloid is engulfed in vesicles by pinocytosis and absorbed into the cell. This releases T4 and T3, as well as inactive iodotyrosines. The biologically active thyroid hormones T4 and T3 enter the circulation; DIT and MIT are deiodinated and their iodide conserved. Thyroid hormone secretion is stimulated by TSH and inhibited by excess iodide and lithium.
4.3 Thyroid Hormone Transport
In the blood, T4 and T3 are almost entirely bound to plasma proteins. There are three major thyroid hormone transport proteins: thyroxine-binding globulin (TBG); transthyretin, formerly called thyroxine-binding prealbumin (TBPA); and albumin and only 0.04% of T4 and 0.4% of T3 are “free.”
Only the free or unbound hormone is available to tissues and it is the free fraction that is responsible for hormonal activity. The metabolic state correlates more closely with the free than the total hormone concentration in the plasma. The levels of free hormones are normal in states where there are primary or secondary changes in plasma binding proteins, because TSH release is controlled by the free thyroid hormone level and adjusts to normalize it irrespectively of how much hormone is bound by the plasma proteins. The concentration of free hormones does not necessarily vary directly with that of the total hormones, for example, while the total T4 level rises in pregnancy, the free T4 level remains normal.
4.4 Regulation of Thyroid Function

The hypothalamic–pituitary–thyroid axis (iodide, I)
4.5 The Molecular Actions of the Thyroid Hormones
The Effects of the Thyroid Hormones
Increase in basal metabolic rate (calorigenic effect)
Promotion of cell division
Regulation of growth, differentiation, and development of tissues and organs, bone maturation
Brain maturation (important role in the myelination process) during intrauterine life and the first years of life; development of neuroendocrine system and of intelligence
Cardiovascular effects: chronotropic and inotropic ⊕ effects, by increasing expression of β-adrenergic receptors; ↓ in vascular resistance
Enhance the motility of the digestive tract
Growth of the long bones; teeth eruption, growth and maturation (← GH effect)
↑ of bone turnover, ↑ of bone resorption, and, to a lesser extent, bone formation
Carbohydrate metabolism: ↑ liver gluconeogenesis, glycogenolysis, and intestinal absorption of glucose
Protein metabolism: biphasic effect (physiologic conditions: anabolic effect; stress conditions: catabolic effect)
Lipid metabolism: fat mobilization, liver synthesis of lipids, stimulation of tissue lipolysis; ↑ liver synthesis of triglycerides (TGs); ↑ synthesis of LDL receptors→ ↑ LDL-cholesterol (LDL-c) clearance
4.6 Thyroid Investigations
4.6.1 Tests of the Thyroid Function
4.6.1.1 Basic Tests
T3, T4, FT3, FT4
Highly specific and sensitive chemiluminescent and radioimmunoassays are used to measure serum T4 and T3 concentrations. Free hormone concentrations usually correlate better with the metabolic state (and have a greater accuracy in reflecting the thyroid function) than do total hormone concentrations because they are unaffected by changes in binding protein concentration or affinity.
TSH
Serum TSH concentration is used as first line in the diagnosis of primary hypothyroidism and hyperthyroidism! Because thyroid dysfunction usually arises from primary disorders of the thyroid gland, serum TSH measurement is the most widely employed test to determine whether thyroid dysfunction exists.
Note: The test may be misleading in patients with secondary thyroid dysfunction due to hypothalamic/pituitary disease.
Interpretation of Tests (Table 4.1)
Thyroid hormone concentrations in various thyroid abnormalities
Condition | TSH | Free T4 | Free T3 |
---|---|---|---|
Primary hyperthyroidism | Undetectable | ↑↑ | ↑ |
T3 toxicosis | Undetectable | Normal | ↑↑ |
Subclinical hyperthyroidism | ↓ | Normal | Normal |
Secondary hyperthyroidism (TSH-oma) | ↑ or normal | ↑ | ↑ |
Thyroid hormone resistance | ↑ or normal | ↑ | ↑ |
Primary hypothyroidism | ↑ | ↓ | ↓ or normal |
Subclinical hypothyroidism | ↑ | Normal | Normal |
Secondary hypothyroidism | ↓ or normal | ↓ | ↓ or normal |
4.6.1.2 Dynamic Tests
Check TSH at 0′ then give protirelin (TRH) 200–400 μg IV and check TSH 60 min later; a normal response: TSH at 60 min increases 2× the basal values.
However, its main use is in the differential diagnosis of elevated TSH in the setting of elevated thyroid hormone levels ⇒ in the differential diagnosis of resistance to thyroid hormone and a TSH-secreting pituitary adenoma.
4.6.2 Antibody Screen
High titers of antithyroid peroxidase (anti-TPO) antibodies and/or antithyroglobulin antibodies are found in patients with autoimmune thyroid disease (Hashimoto’s thyroiditis, Graves’s disease, and sometimes euthyroid individuals).
4.6.3 Thyroid Ultrasonography
The standard transducer for neck imaging is a multifrequency, linear array probe in the 10–15 MHz range which is ideal for examining the superficial structures of the neck-thyroid and lymph node areas.
Thyroid ultrasonography provides an accurate indication of thyroid size (the thyroid volume-upper normal limit <16 ml in women and <18 ml in men) and it can reveal the structure of the thyroid gland: for example, an enlarged/or not, hypoechoic gland suggests either Graves’ disease or subacute thyroiditis, Hashimoto’s thyroiditis.
It is useful for differentiating cystic nodules from solid ones but cannot be used to distinguish between benign and malignant disease.
Certain features of nodules are more common in malignant neoplasm (e.g., hypoechogenicity, microcalcifications, irregular margins, central vascularity, incomplete halo; see also later), and other features are typical of benign lesions (e.g., iso- or hyperechogenicity, a spongiform appearance and cystic change), but none of these features can be considered definitive.
Sequential scanning can be employed to assess changes in the size of thyroid and thyroid nodules over time.
Sonography can be used to guide fine-needle aspiration of thyroid nodules.
4.6.4 Scintiscanning
The Main Uses
To provide information about the size and shape of the thyroid gland and the distribution of tracer activity within the gland → to define areas of ↑ or ↓ function within the thyroid that occasionally helps in cases of uncertainty as to the cause of the thyrotoxicosis (see Table 4.2).
To distinguish between Graves’ disease and a thyroiditis (autoimmune or viral—de Quervain’s thyroiditis).
To detect retrosternal goiter.
To detect ectopic thyroid tissue.
To assess if a thyroid nodule is “hot” (functioning) or “cold.”
Radionuclide scanning (scintigram) in thyroid disease
Condition | Scan appearance |
---|---|
Graves’ hyperthyroidism Thyroiditis (e.g., de Quervain’s) Toxic nodule Thyrotoxicosis factitia Toxic multinodular goiter | • Enlarged gland with homogeneous radionucleotide uptake • Low or absent uptake • A solitary area of high uptake • Depressed thyroid uptake • Multiple functioning nodules (areas of high uptake) in the gland or occasionally an irregular, patchy distribution of radioactive iodine |
RAIU (24 h)—directly proportional with TSH levels
—indirectly proportional with iodine pool
Note: RAIU does not determine the hormonal functional status of thyroid gland, even though it can be useful to differentiate among the causes of thyrotoxicosis particularly when used in conjunction with imaging.
FT4 and FT3 (+TSH) measurements reflect the THYROID FUNCTION.
4.6.5 Thyroid Biopsy
Fine-needle aspiration biopsy (FNAB) of a thyroid nodule is the best method for differentiating benign from malignant thyroid nodules.
4.7 The Nontoxic Diffuse Goiter and the Nodular Thyroid Disease
Nontoxic goiter (i.e., goiter not associated with hyperthyroidism) that can be diffuse or nodular and
Toxic multinodular goiter.
Nontoxic goiter may be defined as any thyroid enlargement that is characterized by uniform or selective (i.e., restricted to one or more areas) growth of thyroid tissue, is not associated with overt hyperthyroidism or hypothyroidism, and does not result from inflammation or neoplasia.
A thyroid nodule is defined as a discrete lesion, within the thyroid gland, due to an abnormal, focal growth of thyroid cells.
WHO Staging of Goiter
Grade 1 = small goiter; it can’t be observed with the head in a normal position (only with the head in hyperextension), but it can be palpated.
Grade 2 = moderate goiter; physical examination: visible with the head in normal position, palpable; it does not extend beyond the external branch of sternocleidomastoid muscle.
Grade 3 = large goiter: one or both lobes extend beyond the external branch of sternocleidomastoid muscle; it is visible from 10 m distance; ± pressure symptoms in the neck.
Grade 4 = huge goiter; extend inferiorly to present as substernal goiter and superiorly toward the mandible; it totally deforms the neck and it can be “seen” from behind; it is accompanied by important pressure symptoms in the neck.
4.7.1 Etiology of Goiter
- 1.
Autoimmune thyroid disease (Hashimoto’s thyroiditis)
- 2.
Endemic (iodine deficiency, dietary origins)
- 3.
Sporadic
- 4.
Congenital causes of goiter: inadequate hormone synthesis due to inherited defect in thyroidal enzymes necessary for T4, T3 biosynthesis (dyshormonogenesis) (e.g., defects in the expression or function of TPO, defects in thyroglobulin synthesis) or due to Pendred syndrome
- 5.
Subacute thyroiditis
- 6.
Neoplasm, benign or malignant
- 7.
Drug-induced (antithyroid drugs, lithium, amiodarone, TKI, checkpoint inhibitors)
4.7.2 Pathogenesis
It is far from being understood. TSH has long been considered the major stimulus for thyroid growth in response to any factor impairing thyroid hormone synthesis. However, serum TSH concentration is normal in most patients with nontoxic goiter. Indeed, as the later researches suggest, any factor that impairs intrathyroidal iodine levels may lead to gradual development of goiter in response to normal concentrations of TSH. Moreover, it appears that a complex network of both TSH-dependent and TSH-independent pathways directs thyroid follicular cell growth and function and plays a role in the goitrogenic process.
The role of genetic factors. Some goiters are due to mutations in genes involved in thyroid growth and/or thyroid function, as suggested by the clustering of goiters within families.
Iodine Deficiency (Endemic Goiter) and Congenital Causes of Goiter
Optimal iodine requirements for adults: 150–300 μg/day. In endemic goiter areas, the daily intake (and urinary excretion) of iodine falls <50 μg/day and the gland is unable to maintain adequate hormonal secretion, and thyroid hypertrophy (goiter) and sometimes hypothyroidism result. It is in these areas that 90% of populations has goiter and 5–15% of infants are born with myxedematous or neurological changes of cretinism. The variability in the extent of goiter in these areas may be related to the presence of other, unidentified goitrogens (e.g., goitrogens in foodstuff or as endemic substances or pollutants).
Indeed, in addition to iodine deficiency and genetic susceptibility, the exposure to a variety of environmental factors has been linked to goiter generation. Thus, endocrine disruptors, including perchlorate, thiocyanate, and nitrate; isoflavones; and organochlorines as well as drugs, smoking, selenium deficiency, insulin resistance, oral contraceptives, parity, and alcohol have been suggested to be involved in goiter development.
The development of nontoxic goiter in patients with severe iodine deficiency (or with dyshormonogenesis) involves impaired thyroid hormones synthesis and, secondarily, increases in TSH secretion which leads to diffuse thyroid hyperplasia ± focal or nodular hyperplasia.
Over an extended period of time a diffuse, nontoxic goiter may progress to multinodular nontoxic goiter and to toxic multinodular goiter (with hyperthyroidism).
Note: The mechanism for the development of autonomous growth and function of thyroid nodules may involve some activating somatic mutations in the TSH rec and α subunit of the guanyl nucleotide stimulatory protein (Gs) in the cell membrane which result in thyroid cell proliferation and hyperfunction in the absence of TSH, which is suppressed.
The most common cause of thyroid enlargement in developed countries is chronic thyroiditis (Hashimoto’s thyroiditis).
Gene mutations can produce a benign lesion, such as adenoma, or a malignant one, such as carcinoma.
4.7.3 Clinical Presentation
Thyroid enlargement: diffuse or multinodular.
Pressure symptoms: large, huge goiter which may displace or compress adjacent structures such as trachea, esophagus, and neck vessels can be rarely associated with symptoms and signs, including dyspnea, inspiratory stridor, dysphagia, choking sensation, and neck tightness or discomfort.
Facial flushing and dilatation of cervical veins on lifting the arms over the head (positive Pemberton sign ← obstruction to jugular venous flow).
Hoarseness: invasion or compression of the recurrent laryngeal nerve, causing hoarseness, rarely occurs, though, when present, it suggests advanced thyroid carcinoma.
The vast majority of patients are euthyroid; endogenous subclinical thyrotoxicosis caused by autonomously nodules may develop.
4.7.4 Investigations
The ultrasonographic evaluation is the optimal means of evaluating the thyroid gland.
Clinical Utility of Neck Ultrasound
Thyroid gland: size, volume, and characteristics
Nodules: number and characteristics of each nodule: diameters, shape, echogenicity, composition, limits, presence of calcifications, vascularization; differentiates solid from cystic nodules
Lymph node compartments
Follow-up: numbers and diameters of nodules
Guidance for FNAB
Scintigraphy (isotope scanning) reveals a patchy uptake, frequently with focal areas of increased uptake corresponding to “hot” nodules and areas of decreased uptake corresponding to “cold” nodules.
Most thyroid carcinomas are inefficient in trapping and organifying iodine and appear on scans as areas of diminished isotope uptake, referred to as a cold nodule. Unfortunately, most benign nodules also do not concentrate iodine. (!!!10% of surgically removed cold nodules are malignant). This confirms the limited utility of thyroid scintigraphy in identifying cancerous nodules. On the contrary, a hot (functioning) nodule on a radioisotope scan with markedly suppressed or absent uptake in the remainder of the gland suggests a toxic adenoma and makes malignancy less likely. These lesions are typically associated with a suppressed serum TSH level.
Normal or
↑ (high iodine uptake- in endemic regions)
depending on the iodide pool and
(=Iodine avidity, not hyperthyroidism!!!) TSH levels
↓
Hormonal determinations: TSH, FT4 are usually normal
Thyroid autoantibodies: TPO Ab, Tg Ab-high in autoimmune thyroid disease
Neck and mediastinal CT/MRI: In the presence of intrathoracic goiter to define the relationship with surrounding structures
Laryngoscopy: Vocal cord paralysis
FNAB of a thyroid nodule: In malignity suspicion, it has been proved to be the best method for differentiation of benign from malignant thyroid disease; it may have therapeutically utility (e.g., draining a cyst)
Differential diagnosis ⇒ The major problem in differential diagnosis: to rule out cancer
4.7.5 Treatment
- 1.
With the exception of those due to neoplasm, nontoxic goiters simply need observation. They can be monitored by clinical examination and evaluated periodically with ultrasound measurements.
- 2.
Medical treatment → in diffuse goiter and multinodular goiter (after excluding malignity):
- (a)
IODIDE (JODID® 100–200 μg/day) is usually administered in diffuse goiter and in young patients.
- (b)
Levothyroxine 25–50 μg/day in nontoxic multinodular goiter and diffuse goiter (=suppressive therapy).
For more than a century, thyroid hormone supplementation (termed suppressive therapy) was employed to reduce the size of nontoxic goiters. Patients with nodular thyroid disease appear to be less responsive to suppressive therapy than those with diffuse nontoxic goiters.
Note: Pharmacologic doses of iodides (in the forms of amiodarone, radiocontrast agents) may induce either hyperthyroidism in patients with autonomous thyroid nodules or hypothyroidism.
- (c)
Treating the hyperthyroidism when a multinodular goiter becomes toxic with one or more hyperfunctioning nodules with antithyroid drugs/surgery/radioiodine.
- (a)
- 3.
Surgical treatment:
For goiter that continues to grow despite TSH suppression with Levothyroxine.
For goiters that produce obstructive symptoms, with substernal extension.
- 4.
131I therapy for nontoxic goiter → to reduce the size of a massive goiter in elderly patients who are poor candidates for surgery or to treat goiter that recurs after resection.
- 5.
Preventive measures:
IODIDE for risk groups: pregnancy, lactation, and aged: 6–14 and 14–18
4.8 Thyroid Nodules and Thyroid Cancer
Thyroid nodules are extremely common, particularly among women. The prevalence of palpable nodule is estimated to be about 4% of the adult population. In contrast to the relatively low prevalence of palpable nodule, thyroid nodules are detected on thyroid ultrasound in up to 50% of healthy individuals. Although thyroid nodules are common, thyroid cancer is a relatively rare condition (less than 5–10% of nodules are cancerous) (Table 4.3), with an annual incidence of thyroid cancer ≈8.7 per 100,000 population.
4.8.1 Etiology of Benign Thyroid Nodules
- 1.
Focal thyroiditis
- 2.
Dominant nodule in a multinodular goiter
- 3.
Benign adenoma (Follicular, Hurthle cell)
- 4.
Thyroid, parathyroid, and thyroglossal cysts
- 5.
Agenesis of a thyroid lobe
- 6.
Postsurgical, postradioiodine remnant hyperplasia
- 7.
Rare: lipoma, hemangioma, and teratoma
The types and approximate frequency of malignant thyroid tumors
Papillary carcinoma | 80% |
Follicular carcinoma (including Hurthle cell carcinoma) | 10% |
Medullary carcinoma | 5% |
Undifferentiated (anaplastic) carcinoma | 3% |
Miscellaneous (lymphoma, fibrosarcoma, teratoma, and metastatic cancer) | 1% |
Because both papillary and follicular cancers arise from thyroid epithelial cells and sometimes respond to TSH and concentrate iodine, they are grouped together as differentiated thyroid cancers.
4.8.2 Clinical Evaluation
An asymptomatic thyroid mass may be discovered either by a clinician on routine neck palpation or by the patient during self-examination. History and clinical examination should concentrate on risk factors that predispose to benign or malignant disease.
A previous history of radiation, especially childhood head and neck irradiation.
A family history of papillary or medullary carcinoma (MEN 2 syndrome).
The development of hoarseness or dysphagia, history of recent thyroid growth.
Nodules are more likely to be malignant in patients <20 or >60 years.
Thyroid nodules are more common in ♀ but more likely to be malignant in ♂.
Physical findings suggestive of malignancy include a solitary firm or hard, nontender nodule/a dominant nodule in a multinodular goiter that is clearly different from the rest of the gland, fixation to adjacent tissue, and the presence of regional lymphadenopathy.
History of Hashimoto’s disease (↑ incidence of lymphoma).
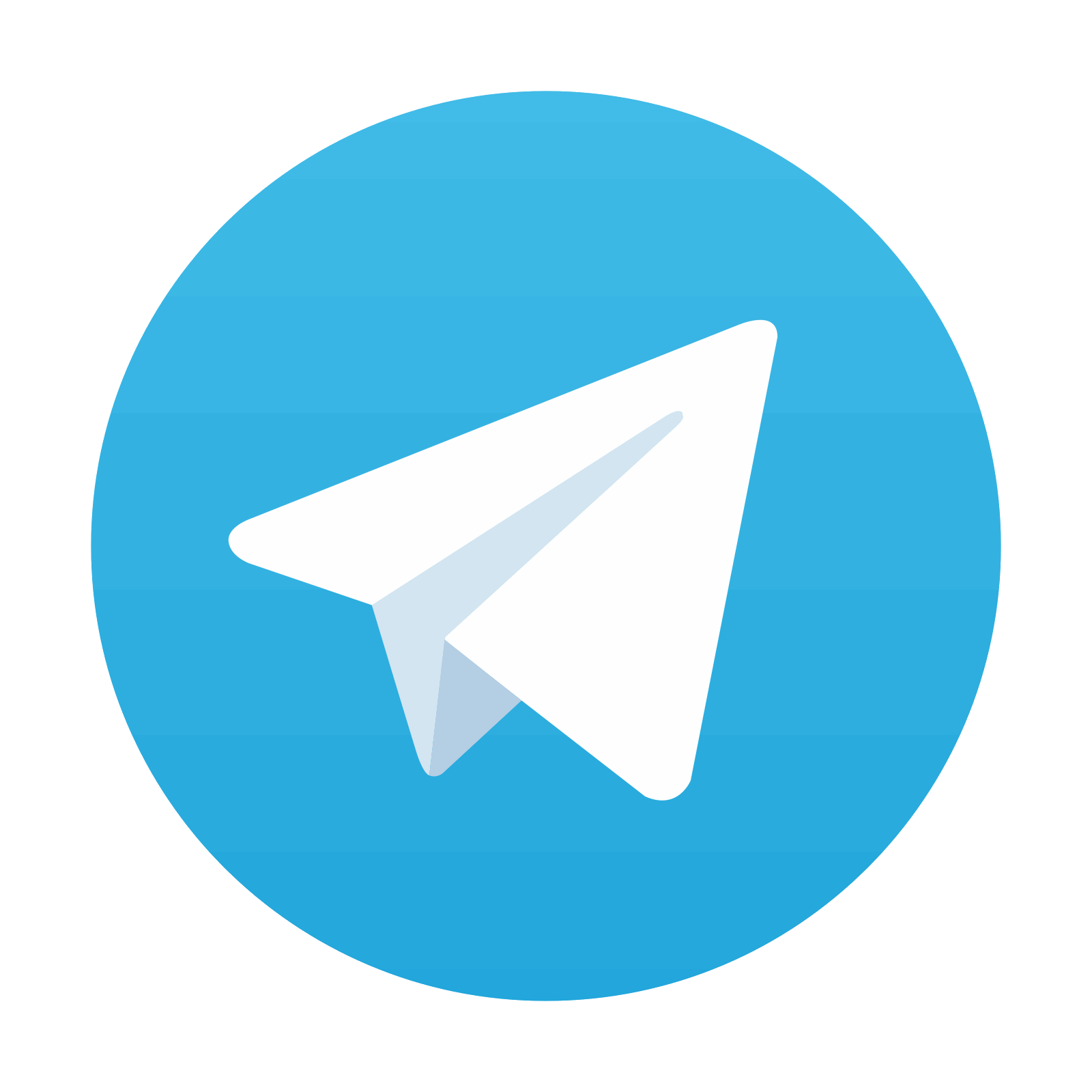
Stay updated, free articles. Join our Telegram channel

Full access? Get Clinical Tree
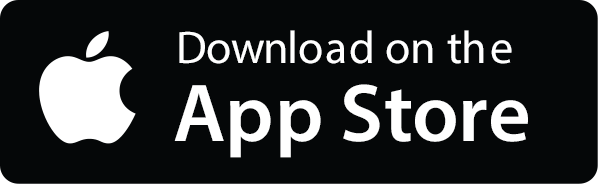
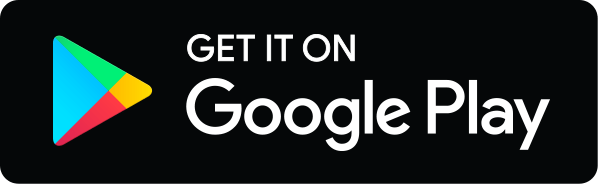