6 The thyroid gland develops from the floor of the pharynx at four weeks gestation in the form of a diverticulum which travels caudally leaving the thyroglossal tract in the neck. The latter normally disappears but cystic remnants may remain and form a thyroglossal cyst. The diverticulum becomes bi‐lobed and fuses with the ventral aspect of the fourth pharyngeal pouch. By 10 weeks gestation, the gland has a butterfly‐shaped structure with its two lobes connected by the isthmus. The genetic mechanisms controlling thyroid organogenesis are incompletely understood but four transcription factors NKX2‐1 (TTF1), FOXE1 (TTF2), PAX8, and HHEX are known to be involved from 20 days post‐fertilization onwards. Integrity of the thyroid stimulating hormone receptor (TSHR) is also known to affect thyroid development since bi‐allelic loss‐of‐function mutations results in thyroid hypoplasia. The main function of the thyroid gland is to synthesize thyroxine (T4) and triiodothyronine (T3). The hypothalamus secretes thyrotropin‐releasing hormone (TRH), which stimulates the anterior pituitary to secrete thyroid stimulating hormone (TSH). TSH acts on the thyroid follicular cell by binding to a specific receptor (TSHR) as shown in Figure 6.1. The occupied receptor activates the G stimulatory protein, which then stimulates the thyroid metabolism via the adenylate cyclase, calcium, and phospholipase C pathways. Figure 6.1 Schematic representation of TSH receptor, TSHR, and intracellular pathways. Gs‐protein, G stimulatory protein; PIP3, phosphatidyl inositol triphosphate. Figure 6.2 shows the main steps in T4 synthesis. Dietary iodine is actively transported into the follicular cells by the sodium iodide symporter (NIS) and from the cells to the colloid by Pendrin and other apical transporters. Iodine is oxidized to iodide by thyroid peroxidase (TPO). The hydrogen peroxide (H2O2) required for this process is generated by the dual oxidase 2 enzymes (DUOX2 and DUOXA2). Tyrosyl residues on the thyroglobulin molecule are then iodinated to form monoiodotyrosine (MIT) and diiodotyrosine (DIT). MIT and DIT are coupled to form T3 and T4. Iodide is recycled from uncoupled MIT and DIT by iodotyrosine dehalogenase. Inactivating mutations at any of these steps result in dyshormonogenesis. The thyroid gland is the sole producer of T4, essentially a prohormone, which is mostly deiodinated to the bioactive T3 in peripheral tissues. Figure 6.2 Schematic representation of thyroxine (T4) and triiodothyronine (T3) synthesis. TPO, thyroid peroxidase; H2O2, hydrogen peroxide; Tg, thyroglobulin; MIT, monoiodotyrosine, DIT, diiodotyrosine; TBG, thyroxine binding globulin; TBPA, thyroid binding pre‐albumin; Alb, albumin; rT3, reverse T3. ↑ refers to the sodium iodide symporter (NIS). After T4 is secreted by the thyroid gland, it is metabolized by the tissue enzymes deiodinase type I, II and III. Type II deiodinase catalyzes T4 to T3 conversion by outer ring deiodination (ORD) (Figure 6.3). Type III deiodinase converts T4 to the inactive reverse T3 (rT3) by inner ring deiodination (IRD) while type I deiodinase catalyzes both ORD and IRD. Seventy per cent of circulating T4 and 50% of circulating T3 are bound to thyroxine‐binding globulin (TBG), the remainder to other proteins, primarily albumin. Only 0.03% of circulating T4 and 0.3% of T3 are unbound (i.e. free to act on target cells). Total T4 and T3 concentrations therefore reflect TBG concentration, so that measurement of free thyroid hormones gives a more accurate assessment of thyroid function. Figure 6.3 Conversion of thyroxine (T4) to tri‐iodothyronine (T3) by outer ring deiodination (ORD) and to reverse T3 (rT3) by inner ring deiodination (IRD). The thyroid hormones have profound effects on growth, neurological development, metabolism, and cardiovascular function. T4 and T3 bind to α1, β1, and β2 receptors in the target tissues, for example, heart and skeletal muscle (α1), brain, liver, and kidney (β1), hypothalamus and pituitary (β2). Binding of thyroid hormones to their receptors results in stimulation of target cells with an increase in oxygen consumption, altered protein carbohydrate and lipid metabolism, and potentiation of the action of catecholamines. During the first trimester the foetus is largely dependent on small amounts of maternal T4 and T3 that cross the placenta. Levels of TSH start to rise from the second trimester onwards. T4 and T3 concentrations are low in foetal plasma since T4 and T3 are predominantly inactivated, but they are present in relatively high concentrations in target tissues, such as the brain. Some maternal T4 crosses the placenta in late gestation, which explains why cord serum T4 from athyreotic newborns is 20–50% of normal. Iodine freely crosses the placenta so that the intellectual disability seen in the offspring of iodine‐deficient mothers can be readily prevented by prenatal iodine administration. At birth, there is an acute release of TSH. This postnatal TSH surge, which is presumably of physiological benefit, results in high T4 and T3 levels (Figure 6.4). The ratio of T4 and T3 to rT3 rises, corresponding to preferential ORD of T4 and decreased thyroid hormone inactivation. Levels of T4 and T3 are high by 7 days, falling thereafter so that by 14 days of age concentrations are similar to those found in infancy and childhood. Figure 6.4 Changes in serum TSH and T4 concentrations in full term and premature infants during the first five days of life. Source: From Fisher and Klein 1981, copyright 1981 Massachusetts Medical Society. All rights reserved. The preterm infant, especially below 34 weeks gestation, shows the foetal pattern of low plasma T3 and T4 with high rT3. Factors contributing to this pattern include immaturity of the hypothalamic–pituitary axis, premature interruption of the small but significant maternal contribution to circulating thyroid hormone levels, persistence of the foetal tendency towards inactivation of T4 and T3 and a negative iodine balance. It is uncertain whether or not the preterm thyroid state should be regarded as physiological, or pathological and requiring intervention. The situation is complicated by the knowledge that concentrations of thyroid hormones in the tissues may be substantially different to those found in the plasma. The issue of low T4 levels in preterm infants is discussed further below. Table 6.1 shows the normal values for the commonly measured thyroid function tests (TFTs). Free T4 (FT4) or T4 and TSH measurements are the mainstay of assessment in hypothyroidism, with TSH the most sensitive indicator of primary disease. In primary hyperthyroidism, TSH is suppressed and there is preferential conversion of T4 to T3, so that measurement of both hormones is helpful in the management of thyrotoxicosis. TBG measurement is redundant if FT4 is measured. Measurement of antibodies to TPO or to thyroglobulin (Tg) may be used to confirm autoimmune thyroiditis. Stimulatory TSH receptor antibodies are positive in Graves’ disease. Tg is a 660 kDa protein synthesized exclusively in the thyroid gland so that the presence of thyroid tissue can be inferred if it is detectable in the serum. Thyroglobulin is of value in diagnosing the cause of congenital hypothyroidism (see section ‘Congenital hypothyroidism’) and in the monitoring of patients following thyroidectomy and 131I ablation for thyroid cancer. In pituitary and hypothalamic diseases, TSH levels will be inappropriately low for the low FT4 (or T4) level. TRH, the response to which helps distinguish hypothalamic from primary and pituitary hypothyroidism, is no longer routinely available for testing. Magnetic resonance imaging (MRI) may show anterior pituitary hypoplasia or ectopic posterior pituitary in children with central congenital hypothyroidism. Table 6.1 Suggested reference ranges for common thyroid function tests, after values from Royal Hospital for Children, and Glasgow Royal Infirmary, Glasgow, UK. Thyroglobulin values are from 29 iodine‐sufficient term infants from Czech Republic, analysed at Glasgow Royal Infirmary (Neumann et al. 2018). NB. Reference ranges for TSH receptor antibody vary widely according to the assay used. T3, triiodothyronine; T4, thyroxine; TSH, thyroid stimulating hormone; TPO, thyroid peroxidase. Hypothyroidism is defined as a state in which the hypothalamic–pituitary–thyroid axis is failing, or is in danger of failing, to produce sufficient T4. Classification is according to: Hypothyroxinaemia is defined as FT4 levels below the reference range in the absence of an elevated TSH. This biochemical profile is encountered in the preterm infant; severe non‐thyroidal illness (‘sick euthyroid syndrome’, in which T3 is typically low initially but FT4 may eventually decrease as well); malnutrition, anorexia nervosa, diabetic ketoacidosis, and in association with certain drugs. During recovery, thyroid hormone levels spontaneously return to normal but TSH often overshoots transiently. Hyperthyrotropinaemia is a purely descriptive term which refers to a mild elevation of TSH (e.g. 7–15 mU/L) in the context of normal FT4 (or T4) concentrations. Hyperthyroidism refers to over‐production of T4 and/or T3, and is almost invariably primary with TSH suppression. Goitre refers to thyroid gland enlargement. It is best inspected with the neck slightly extended, asking the child to swallow to make the gland more obvious; best palpated when standing behind the child; and described clinically in terms of size, texture (e.g. smooth or nodular), consistency, and symmetry. Goitres may be sub‐classified according to thyroid function: hypothyroid, hyperthyroid, or euthyroid. Preterm babies are susceptible to transient hypothyroxinaemia (TH), the incidence of which increases with decreasing gestational age and increasing neonatal morbidity. The hypothyroxinaemia may be secondary to immaturity of the hypothalamic–pituitary axis and the T4 level may be normal for the infant’s gestational age. TSH levels are within the reference range. TFTs should be repeated after two weeks and kept under surveillance. Treatment is only indicated if the T4 level remains low with an elevated TSH. There is a recognized correlation between the severity of hypothyroxinaemia and neonatal outcome. However, causality has never been established and to date there is no evidence that thyroid hormone replacement therapy improves outcomes in this population. A Cochrane review does not support the use of thyroid hormone supplementation in preterm infants to reduce neonatal mortality, neonatal morbidity or improve neurodevelopmental outcomes (Osborn and Hunt 2007). A United Kingdom randomized controlled trial of L‐T4 supplementation in babies below 28 weeks’ gestation showed no beneficial effect on brain growth in treated infants (Ng et al. 2013). At present supplementation of preterm babies with thyroid hormones cannot be recommended but there is a need for further research. This non‐specific label should only be used once mild compensated primary congenital hypothyroidism (e.g. due to thyroid ectopia) has been excluded by thyroid imaging. Neonatal hyperthyrotropinaemia may reflect the physiological TSH surge or be caused by delayed maturation of the hypothalamic–pituitary–thyroid axis. It is more common in preterm infants. No treatment is required but the infant should be kept under surveillance until the TSH normalizes. Transient TSH elevation, with or without a low FT4 (or T4), accounts for roughly 25% of cases referred by the screening laboratory if TSH is measured on day 5. It is strongly associated with sick and/or preterm newborns and those suffering from congenital malformations (Table 6.2). More rarely, it is caused by transplacental transfer of maternal medication, transplacental transfer of maternal TSH receptor blocking antibodies, or iodine deficiency or excess. If TSH elevation is >10 mU/L, then treatment should be started (see section ‘Congenital hypothyroidism’). Table 6.2 Causes of permanent and transient, syndromic and non‐syndromic neonatal hypothyroidism. Source: Adapted from Donaldson and Jones (2013), and reproduced with kind permission. Congenital hypothyroidism is one of the most common disorders in paediatric endocrinology with a prevalence of 1 in 6700 live births in countries where newborn screening is not performed, increasing to 1 in 2000–3000 live births when screening is carried out (Grosse and Van Vliet 2011). It is the most common cause of preventable learning difficulties. Worldwide, iodine deficiency is the dominant cause. In areas with severe iodine deficiency, congenital hypothyroidism is endemic and leads to learning difficulties, short stature, deafness, and neurological abnormalities. This accounts for approximately 70% of permanent congenital hypothyroidism. The most common form is thyroid ectopia when caudal migration is incomplete, resulting in an ectopic (usually sublingual) gland which is always hypoplastic. Athyreosis, where no thyroid tissue is detectable on imaging is the second commonest cause. It may be subdivided into apparent athyreosis, in which thyroglobulin is detectable, and true athyreosis when it is not (Gagné et al. 1998). Hypoplasia in situ is less common than ectopia and athyreosis. It may result from a TSH receptor mutation which, if severe, causes severe hypoplasia or even apparent athyreosis; and from a PAX8 mutation. Inherited or de novo gene mutations affecting the transcription factors NKX2‐1 and FOXE1 genes, sometimes in association with syndromes (see Table 6.2), are responsible for probably <2% of cases of thyroid dysgenesis. Hemiagenesis is not a cause of congenital hypothyroidism since the contralateral lobe compensates. Autosomal recessive defects in thyroid hormone synthesis account for 15–20% of congenital hypothyroidism in the United Kingdom but occur more commonly in parts of the world where the prevalence of consanguinity is high (e.g. the Middle East, North Africa). The most common genes affected are TPO and thyroglobulin. Pendred’s syndrome – sensorineural deafness with or without dyshormonogenetic goitre – is due to a mutation in the Pendrin gene. DUOX2 mutations result in congenital hypothyroidism which may be transient in nature. Mutations in the iodotyrosine dehalogenase gene result in iodine wasting with a goitre, similar to that of dietary iodine deficiency. Sodium iodine symporter gene defects are rare, and show lack of uptake on radioisotope scan. In contrast to infants with thyroid dysgenesis, in whom thyroid hypoplasia is found, thyroid imaging of infants with biosynthetic defects will show a normal or enlarged thyroid gland in situ, provided that the defect is distal to the TSH receptor. Other causes of congenital hypothyroidism are rare. TSH or TRH deficiency accounts for hypothyroidism in approximately 1 in 100 000 births and usually occurs in the context of malformations associated with multiple pituitary deficiencies, for example, septo‐optic dysplasia. Newborn screening for congenital hypothyroidism using dried capillary blood spots was developed in the 1970s, in response to the combination of difficulty in achieving an early clinical diagnosis and the severe neurodevelopmental consequences of a late diagnosis, especially beyond three months of age (Grosse and Van Vliet 2011). By the early 1980s, newborn screening was established in North America and most of Europe, but has yet to be implemented in many parts of the world, including the Maghreb countries of North Africa. It is important to recognize that no screening programme is perfect and that occasional errors – missed patients, mis‐labelling and loss of samples – are inevitable. Thus clinical vigilance is essential, whether newborn screening is in place or not. Screening samples are usually collected as capillary blood from the baby’s heel but cord blood sampling may be more feasible in resource‐limited settings. Guidelines from the European Society for Paediatric Endocrinology (ESPE) recommend that screening should take place 48–72 hours after birth (Léger et al. 2014). In the United Kingdom, screening is normally performed between days 4 and 7. In North America, babies are usually tested between 48 hours and four days, but may be tested before 48 hours of age if they are discharged from the hospital prior to that time. Early sampling may lead to false positive results and it is important that the TSH value is interpreted in the light of age‐appropriate reference ranges. Options include TSH measurement only (the most sensitive method for primary hypothyroidism but which will miss central hypothyroidism), T4 measurement only (which will miss compensated hypothyroidism) and measurement of both T4 and TSH (the ideal but most labour‐intensive and costly method). In most of the United Kingdom and North America, TSH‐only measurement is used. Since 2002 in Scotland, a capillary TSH of ≥25 mU/L (measured on whole blood) triggers immediate notification by the laboratory, which should be achieved within a set standard of 14 days (Mansour et al. 2017). When the capillary TSH value ranges between 8 and 24 mU/L, a second sample is requested, and the infant is referred if the repeat value is ≥8 mU/L. Values of <8 mU/L are reported as normal. The ESPE guidelines recommend repeat sampling at discharge in infants who were born preterm (gestation <37 weeks) or were ill at the time of initial sampling, neonates from multiple births in whom foetal blood mixing may have occurred, infants exposed to iodine, and recipients of blood transfusion, dopamine infusion, etc. The rationale for second testing is that TSH suppression might occur in sick and preterm infants, thus masking true congenital hypothyroidism. While Public Health England also advocates repeat screening at 28 days in preterm infants (NHS guidelines for Newborn Blood Spot Sampling 2016), this approach has not been adopted by all centres, some arguing that the delayed TSH rise in such infants is usually transient in nature. Devices are being developed for measuring TSH on capillary blood at the time and place of patient care (point‐of‐care testing). This approach hold promise for screening in areas with restricted resources. Awareness of the clinical symptoms and signs is required for the detection of infants missed on the screening programme and for the assessment of infants with high TSH values. Given the increased prevalence (5%) of non‐thyroidal malformations (particularly cardiac) in congenital hypothyroidism, and the association with dysmorphic syndromes (e.g. Down, Albright’s hereditary osteodystrophy), a thorough examination is indicated. If hypothyroidism is suspected on neonatal screening, then an urgent venous sample for FT4 (or T4) and TSH measurement should be taken to confirm the diagnosis. Thyroglobulin measurement is helpful in selected cases, being undetectable (<2 μg/L) in true athyreosis and mutations in the thyroglobulin gene, detectable in apparent athyreosis, often high‐normal or elevated (>400 μg/L) in thyroid ectopia, and usually markedly elevated (>1000 μg/L) in dyshormonogenesis (except if due to mutations in Tg). Transplacental transfer of TSH receptor blocking antibodies only accounts for ~1% of cases (Brown et al. 1996). Failure of one or both epiphyses to appear on a knee radiograph reflects the severity of intrauterine hypothyroidism and is advocated by some centres as part of the initial assessment. ESPE guidelines recommend thyroid imaging (Figure 6.5) by radioisotope scanning, ultrasound, or both. If athyreosis or ectopia are confirmed, the diagnosis of permanent congenital hypothyroidism is secure, the parents can be informed that lifelong thyroxine treatment will be required, and reassured that the risk of recurrence is low (<2%). The functional disorders with a thyroid gland in situ, entail a one in four risk in siblings and may be permanent or transient. Figure 6.5 Isotope (a and b) and ultrasound (c–e) images from infants with thyroid ectopia (a), thyroid hypoplasia due to a TSH receptor defect (d), and dyshormonogenesis (e). The isotope scans show lingual ectopia, a normally sited small gland, and avid uptake into an enlarged gland, respectively. The coronal ultrasound scans show non‐thyroidal tissue in the thyroid fossa of the infant with thyroid ectopia, reduced thyroid volume in the infant with thyroid hypoplasia, and a bulky gland in the infant with dyshormonogenesis. Note that the non‐thyroidal tissue has a hyperechoic and heterogeneous texture (arrowed), compared with that of the thyroid tissue shown in the two other infants (arrowed), and that it also wraps around the major vessels (broken arrows). T, trachea. While few centres dispute that thyroid imaging in the assessment of newborns with TSH elevation should now be accepted as part of good clinical practice, the optimal mode of imaging remains controversial. When an infant is found by the screening laboratory to have TSH elevation, she/he should be seen by the clinician within 24 hours. Most families will be deeply shocked by the discovery of an abnormal result on their apparently well baby, and the disclosure that the child may have a lifelong disorder. Initial counselling should therefore be brief and simple, reassuring the parents that the treatment of permanent congenital hypothyroidism is straightforward and the outlook excellent, provided that observance with medication is meticulous. In centres where same‐day venous TFTs are available, the need for treatment with levo‐thyroxine (L‐T4) can be judged from the venous FT4 and TSH values. Biochemical severity can be graded according to FT4 values of <5 pmol L (severe), 5–10 pmol/L (moderate), and 10–15 pmol L (mild) (Léger et al. 2014). L‐T4 should be started immediately, therefore, if TSH elevation is confirmed and FT4 is <15 pmol/L. When same‐day venous TFT’s are not available, L‐T4 should be started if capillary TSH is ≥40 mU/L since decompensated hypothyroidism is likely, but may be deferred for 48–72 hours if TSH is <40 mU/L and the infant clinically euthyroid (Pokrovska et al. 2016). Treatment should be started within 14 days of age in infants with venous TSH >20 mU/L and/or fT4 < 15 pmol/L. Well infants with normal fT4 and venous TSH 6–20 mU/L may be observed initially, and thyroid imaging should be performed. If, however, TSH elevation >10 mU/L persists, L‐T4 treatment followed by discontinuation of L‐T4 treatment and retesting at three years is a pragmatic and safe strategy. L‐T4 can be administered as crushed tablets, mixed with a few millilitres of water, breast or formula milk, and given via a spoon or syringe. The smallest tablet is 25 μg, but this can be halved. Liquid forms of thyroxine should only be used if pharmaceutically produced and licensed. The initial dose of T4 should be 10–15 μg/kg per day depending on the severity of the hypothyroidism, equating to 37.5–50 μg daily in most term infants. The aim of treatment is to normalize FT4 and TSH within two weeks if possible, given the evidence of a better neurodevelopmental outcome. During the early weeks of treatment, once the shock of the diagnosis has settled down, detailed and careful counselling is essential. Information leaflets and visual aids are very useful, as is contact with a similarly affected child and family or a support group. FT4 should be maintained in the upper half of the reference range for age (15–20 pmol/L), with TSH in the lower half (0.5–3 mU/L). Sometimes the initial fall in the TSH with treatment is delayed in which the thyroxine dose should be titrated against FT4, keeping this near the top of the reference range. Parents should be aware of symptoms of over‐treatment with thyroxine including crying, irritability, poor sleeping, and diarrhoea. L‐T4 dose can be estimated by assuming a full replacement dose (e.g. in athyreosis) of 100 μg (m2/day (3–4 μg/kg/day), calculating body surface area from the formula √ [length (cm) × weight (kg)/3600. L‐T4 requirement depends on the type and severity of hypothyroidism, being lower in children with ectopia, mild dyshormonogenesis and transient congenital hypothyroidism. A suggested dosage schedule, based on the requirement in the absence of thyroid tissue, is given in Table 6.3. L‐T4 dosage should be pre‐emptively increased to keep pace with body surface area or weight, rather than allowing the TSH levels to rise due to under‐treatment. Most children do well when free T4 is kept between 12 and 20 pmol/L and TSH between 0.5 and 5 mU/L. Table 6.3 Suggested schema for follow‐up of patients with congenital hypothyroidism. Thyroxine (L‐T4) dose is for athyreosis and equivalent to 100 μg/m2/day. a More frequent follow‐up in the case of problems with compliance/adherence. NB Soya milk formulas, iron medication, calcium, and fibre administered in close time proximity to L‐T4 can interfere with L‐T4 absorption. Disorders associated with malabsorption, e.g. coeliac disease, may affect T4 levels as may medications which increase T4 degradation, e.g. anticonvulsants. Such patients require more frequent follow‐up and higher doses of L‐T4. Table 6.3 gives guidance as to how frequently patients should be seen, with clinic attendance no less than every three months in the first year and yearly after three years. Follow‐up should be more frequent if there are concerns about compliance, if TFT’s are abnormal or if the dose has been altered. If the TSH is >5 mU/L and the T4 level is in the lower half of the normal range or below the normal range, then the dose should be raised by 12.5–25 μg per day and the TFTs should be repeated in four to six weeks. Conversely, the dose should be reduced by a similar amount if the TSH level is <0.5 mU/L. The prevalence of sensori‐neural hearing loss is increased in congenital hypothyroidism, even after allowing for Pendred’s syndrome. Thus audiology should be carried out at three years in all affected children, with repeat testing in patients who had severe hypothyroidism at birth. Retesting should normally take place after the age of three years, by which time neurodevelopment is largely complete so that potential short‐term exposure to hypothyroidism carries a low risk of harm. Re‐evaluation is unnecessary in infants already known to have an unequivocal cause of congenital hypothyroidism including athyreosis or ectopia, confirmed on scanning during infancy, genetically proven dyshormonogenesis (with the exception of DUOX2 deficiency which may cause transient hypothyroidism) and in children where venous TSH has been >10 mU/L after 12 months of age while on treatment – indicative of underdosage or poor compliance with thyroxine. However, retesting is required in children who were preterm and/or sick at birth; those with eutopic glands of indeterminate cause; patients in whom thyroid imaging was not performed in infancy; and when the dose of thyroxine has not needed increasing since early childhood. In children requiring re‐evaluation, the ESPE guidelines suggest decreasing the thyroxine dose by 30% and checking thyroid function two to three weeks later. If TSH is >10 mU/L, the diagnosis of congenital hypothyroidism is confirmed. Thyroid imaging may then be performed, to establish the aetiology. If thyroid function remains normal, the dose is decreased further before rechecking thyroid function after two to three weeks. If thyroid function is normal four weeks after stopping treatment, the child can be discharged with a diagnosis of resolved transient hypothyroidism. Neonatal screening programmes have revolutionized the outlook for babies with congenital hypothyroidism and the overall prognosis is now excellent. However, subtle neurocognitive deficits may occur in some children with severe intrauterine hypothyroidism despite adequate postnatal treatment. There is also concern that overtreatment with L‐T4 may cause deficit in attention and memory. Lower intelligence quotient (IQ) has been reported in children with serum T4 of <40 nmol/L prior to treatment, superimposed on the effect of a lower socioeconomic status. (Tillotson et al. 1994). Special attention to severely affected children form socially deprived families is therefore essential. Failure to comply with treatment, especially during the first three years of life, may impair normal brain development. The relationship between intellectual and education outcome, and the quality of postnatal treatment and follow‐up has been demonstrated in France (Léger et al. 2011). Work from Canada and Spain has shown that exposure to high thyroxine levels adversely affects attention (Rovet and Erlich 1995; Rovet and Alvarez 1996; Alvarez et al. 2010). However, although a Norwegian study found more memory and behaviour problems in adults with congenital hypothyroidism compared with sibling controls, a link between high L‐T4 dosage in early childhood could not be confirmed (Oerbeck et al. 2005). Although it is difficult to separate the effects of congenital hypothyroidism itself from the effects of inadequate treatment and/or over‐treatment, the available evidence indicates that outcome can be optimized by: This condition can be difficult to recognize as its onset may be insidious and it has often been present for a number of years prior to diagnosis. Primary Acquired Hypothyroidism Secondary and Tertiary Although this is the most common worldwide cause of hypothyroidism, it more commonly results in a euthyroid goitre. Clinical iodine deficiency is rare in Europe, but an estimated 11 of 35 countries are iodine‐deficient (Lazarus 2014) and there is some evidence that iodine supplementation in mild‐to‐moderate deficiency improves cognition (Taylor et al. 2013). The condition is suspected in cases of goitre, a family history of iodine deficiency and from a knowledge of the regional iodine status. Measurement of urinary iodine concentration (UIC) is valuable for the assessment of iodine status in populations but of limited use in individual diagnosis. The World Health Organization (WHO) classifies iodine deficiency as mild, moderate, and severe if UIC is 50–99, 20–49, and < 20 μg/L. Iodine deficiency is treated with trace amounts of iodine and prevented by iodization of salt. This is practised widely in North America but inconsistently in Europe. This is the most common cause of acquired hypothyroidism in the Western world. It is more common in girls, particularly in adolescence, and there is a family history in approximately a one‐third of cases. Presentation may be with euthyroid goitre, goitre with compensated hypothyroidism, goitre or an atrophic gland with decompensated hypothyroidism or during screening because of the presence of another autoimmune condition. Autoantibodies are present in 95% of cases. Autoimmune thyroiditis may be associated with other autoimmune diseases, such as diabetes mellitus, coeliac disease, and Addison’s disease, as well as with skin disorders, such as alopecia areata and vitiligo. Hashimoto’s thyroiditis is common in Down syndrome, affecting 8.9% of Scottish children (Noble et al. 2000). Regular screening is indicated, one method being annual capillary blood spot TSH screening from one year of age onwards. If the TSH is raised, then venous TFT’s and a TPO antibody measurement should be performed. TSH screening will not detect Hashimoto’s disease if it goes through a hyperthyroidism phase (see p. 156) but this condition should be clinically obvious. Autoimmune thyroiditis is also increased in Turner syndrome (especially with isochromosome X), with a 6.6% prevalence in a Polish study (Gawlik et al. 2011). These include the hypothalamic–pituitary disorders in which other anterior pituitary hormone deficiencies will almost invariably be present. Dietary goitrogens, such as iodide, cabbage and soya beans, have also been reported to cause hypothyroidism. The clinician should be wary of diagnosing hypothyroidism in obese children. Obesity results in TSH resistance, causing mild hyperthyrotropinemia (5–7.5 mU/L) but with normal FT4. L‐T4 treatment is not indicated in this situation, and weight reduction will lead to normalization of TSH. Occasionally pseudohypoparathyroidism (see Chapter 10) may present as mildly raised TSH, for example, on newborn screening. The principal symptoms of acquired hypothyroidism are tiredness and weight gain, while the key signs are pallor, myxoedematous facies, and short stature relative to the mid‐parental height (Figure 6.6). If previous heights are available, it may be possible to pinpoint the start of the hypothyroidism. The goitre is usually diffuse and non‐tender with a firm texture but is nodular in some cases and is occasionally tender. Usually puberty is delayed, but, occasionally, with severe hypothyroidism cross‐stimulation of FSH receptors by extremely elevated TSH concentrations may lead to incomplete sexual precocity with enlarged ovaries on ultrasound scan in girls and testicular enlargement with low testosterone in boys. Paradoxically, in all but the most severe cases of acquired hypothyroidism, children do well at school, methodically doing their homework until it is completed. Figure 6.6 Girl with acquired hypothyroidism caused by Hashimoto’s disease (a) before and (b) after treatment. These will depend on the cause of the hypothyroidism. FT4 and TSH are required to confirm the diagnosis and TPO antibodies should be measured to determine the aetiology. In long‐standing hypothyroidism, bone maturation is delayed. A thyroid ultrasound should be done if a nodule is palpable or if there is clear asymmetry but is otherwise not essential. If there is no goitre and the autoantibody screen is negative, then an isotope or ultrasound scan should be performed to exclude a late presentation of thyroid dysgenesis. Inappropriately low or normal TSH values in the face of low FT4 suggests pituitary or hypothalamic disease which can be further investigated with further pituitary testing and MRI. Treatment is with T4 100 μg/m2 per day given as a single daily dose. Children with clinical hypothyroidism can start on full replacement but some clinicians prefer to prescribe a small dose of T4, 25–50 μg once daily. TFTs should be measured every two to four weeks and the dose adjusted in 25 μg steps as necessary until TFTs have normalized. Regardless of how treatment is initiated, the parents should be warned that treatment for severe hypothyroidism may be associated with short‐term adverse symptoms including irritability, poor concentration, emotional lability, and benign intracranial hypertension. School performance may also temporarily deteriorate. Catch‐up growth with increased height velocity will occur, often with initial weight loss. In those with compensated hypothyroidism, adverse symptoms are much less likely and treatment can be increased more quickly. There is controversy as to which euthyroid patients with compensated autoimmune thyroiditis should be treated since a spontaneous return to the euthyroid state is possible. When TSH is as high as 20 mU/L or more, it is wise to treat with L‐T4 irrespective of accompanying symptoms or FT4 levels. When TSH is 6–10 mU/L in a well patient with normal FT4, a watch‐and‐wait approach is advised. In patients who are well with TSH 10–20 mU/L, the choice lies between a period of close observation and elective L‐T4 treatment, and should be discussed with the family. There is some evidence that treatment will cause a reduction in thyroid volume in patients with goitre. Occasionally, treatment may be associated with the development of a slipped upper femoral epiphysis or Perthes’ disease, both of which cause leg pain and a limp. However, the former is more likely to be seen as a presenting feature of hypothyroidism. Families should be told that in the unlikely event of the child developing leg pain or a limp, they should attend the emergency department that day. Considerable surveillance and reassurance may be required by some families during the first few months following diagnosis. Thereafter, clinic visits and TFTs should take place 6–12‐monthly. The TSH level is a sensitive marker of under‐ or over‐replacement. In the case of non‐compliance, FT4 will be normal if L‐T4 was taken on the day of the clinic visit but the TSH level will be raised. The prognosis for autoimmune thyroiditis is very good and the outlook partly depends on whether the child will develop other autoimmune diseases. Most patients need treatment for life, but spontaneous remission may occur and should be suspected if L‐T4 dose requirement is low. Complete catch‐up growth following treatment can be expected in mild to moderate cases but not always in severely affected children with prolonged hypothyroidism, especially when treatment is initiated after puberty has started. Parents of children with severe hypothyroidism should be warned that it may take several months before their child is completely back to normal. This is caused by the development of antibodies which bind to the TSH receptor and cause inappropriate, unregulated stimulation of T4 production. TPO antibodies are usually also present. The condition is uncommon, with an incidence of 0.8 per 100 000 children per year, but often more severe than in adults when it does occur. Paediatric onset is usually in the second decade. It is six times more common in girls and in up to 60% of cases there is a family history of thyroid disease. It occurs more commonly in children with other autoimmune disorders, including diabetes mellitus, and there may also be a family history of non‐thyroidal autoimmune disease. Graves’ ophthalmopathy, rarely severe in children, usually takes the form of eye prominence or frank proptosis (exophthalmos) (Figure 6.7). It results from infiltration of the orbit and surrounding structures with lymphocytes, mucopolysaccharides, and oedema. Figure 6.7 Girl with Graves’ disease. Autoimmune thyroiditis usually causes hypothyroidism but may cause thyrotoxicosis (‘Hashitoxicosis’) in a small proportion of patients. TPO antibodies are almost always present, TSH receptor antibodies negative, and eye signs absent. An absolute distinction between the two conditions may not always be possible, some believing that they represent different ends of a spectrum. However, separating the two disorders was shown to be clinically helpful in a Scottish study of 66 patients with thyrotoxicosis. Of 13 patients with Hashimoto’s disease, 10 showed spontaneous remission compared with 10 of 53 patients with Graves’ disease (Kourime et al. 2017). Thus, while medical treatment of Hashimoto’s is as for Graves’ disease, neither radioactive iodine nor surgery are usually indicated. The onset of both Graves’ and Hashimoto’s disease is usually insidious but may be acute. Thyroid crisis or storm is a form of thyrotoxicosis characterized by an acute onset which may be precipitated by surgery, infections, drug withdrawal/non‐compliance, and radioactive iodine treatment. The patient develops hyperthermia, severe tachycardia, and restlessness and may become delirious, comatose, or die. It is rare in childhood. The diagnosis is usually obvious and is confirmed by finding elevated FT4, FT3, or T3 with TSH suppression. Rarely, FT4 is normal but FT3 or T3 levels are elevated, so‐called ‘T3 toxicosis’. TSH receptor antibodies are elevated in Graves’ disease and TPO antibodies are usually positive in both Graves’ and Hashimoto’s. This can be divided into first line treatment – medical and second line treatment – either with radioactive iodine or surgery. Symptoms of anxiety, palpitations, and tremor can be distressing, and oral Propranolol treatment 0.5–1mg/kg/day in divided doses makes the child more comfortable. This should be reduced and stopped when FT4 has normalized and symptoms have resolved. In children with asthma, the selective β‐blocker Atenolol may be given. Neither should be used in patients with heart failure. Antithyroid drugs (ATDs) are given as Methimazole (MMI) or Carbimazole which is the pro‐drug of MMI into which it is metabolized. Carbimazole is used in the United Kingdom and is available in 5 and 20 mg tablets. It may be started at 5 mg daily, increasing by 5 mg every two days in two or three divided doses and building up to 0.75 mg/kg/day, maximum dose 30 mg daily, over a two‐week period. The rationale of this slow and cautious approach is to minimize the chance of side effects, including nausea. The patient is seen weekly at this stage and the opportunity taken to counsel the family carefully, particularly when the TSH receptor antibody results are available. After four to eight weeks, FT4 and FT3/T3 will fall to within the reference range but the TSH will remain suppressed for several more weeks. The rapidity of the response is usually proportional to the size of the gland. When the FT4 normalizes at ~ 15 pmol/L the β‐blocker may be reduced and stopped, and one of two ATD strategies put into place. When FT4 is ≤15 pmol/L, the dose of Carbimazole is reduced to 0.5 mg/kg/day, giving this as a single daily dose. Dose is titrated further according to the FT4 and FT3/T3 levels to maintain the hormone levels in the centre of the reference range. It is important not to wait for the TSH to normalize before reducing the Carbimazole dose and to titrate against the FT4 levels. Because adverse effects from Carbimazole and MMI are more common in patients receiving high doses, the aim is to use the lowest dose necessary to maintain a euthyroid state. When FT4 and FT3/T3 are stable on the reduced dose of Carbimazole, the patient is seen monthly and, when good control is achieved, every three months. TSH receptor antibody titre may be repeated annually to give an idea of disease activity. An alternative is not to reduce Carbimazole when FT4 normalizes but instead to introduce L‐T4 in the dose of 50–100 μg daily to counter the hypothyroidism which will occur on 0.75 mg/kg/day of Carbimazole. Carbimazole and FT4 are given as single daily doses. The American Thyroid Association (ATA) guidelines favour dose titration in adults since the block and replace regimen requires a higher ATD dose with more risk of adverse effects and no proof that remission rates are better (ATD guidelines 2016). Also, it is easier to monitor disease activity and hence the imminence of remission, with dose titration. Advocates of block and replace claim smoother control with avoidance of hypo‐ or hyperthyroidism, and the need for less frequent monitoring. Side effects of Carbimazole and MMI include rashes, painful joints (most often wrists and ankles), neutropenia, and liver dysfunction. Neutropenia occurs in approximately 0.3% of patients, usually within the first three months. A slightly greater percentage may develop mild to moderate leucopenia. Patients should be asked to report symptoms of infection, especially sore throat, mouth ulcers, fever, and bruising. In such instances, treatment should be stopped and an urgent full blood count performed. Whenever possible, written information should be provided to back up the verbal advice. Stopping the medication nearly always leads to resolution of the problem after one to two weeks. In severe cases, granulocyte colony stimulating factor may be used. Routine measurement of full blood count is unhelpful and not advised. An itchy erythematous rash occurs in 2–5% of patients on Carbimazole and MMI. This side effect is more common with higher doses and these should rarely exceed 30 mg daily, an apparently higher dose requirement suggesting compliance problems. Carbimazole and MMI can cause liver problems, characterized by cholestatic dysfunction (rather than hepatocellular inflammation or liver failure) and, rarely, liver failure. Propylthiouracil (PTU) has also been used to treat thyrotoxicosis. Unfortunately, there is a 1 in 1000 risk of liver failure in children treated with PTU and a percentage of patients may require liver transplantation or die (Rivkees and Mattison 2009). In view of this, PTU should be restricted to the very few patients in whom Carbimazole or MMI have led to a toxic reaction and in whom both radioactive iodine and surgery are not considered an option. This is usually mild in children and adolescents compared with adults. Dry or painful eyes can be treated with hypromellose eye drops (‘artificial tears’), one drop to each eye up to four times a day. In the case of significant symptoms or impaired eye movements, early referral to an ophthalmologist is indicated but treatment with steroids or decompression is rarely required. While hyperthyroidism from Hashimoto’s thyroiditis can be expected to remit spontaneously, remission rates for Graves’ disease are lower and the family must be counselled that many years of Carbimazole or MMI may be required. Given the relative severity of Graves’ disease in children and the spectrum of severity, there is no logic (and no evidence) to support giving ATD treatment for a fixed period, for example, two or three years, and then stopping therapy to see if the patient has remitted spontaneously. Moreover, work from France and Japan suggests that the chance of spontaneous remission in Graves’ disease is enhanced by prolonged, low dose ATD therapy (Léger et al. 2012; Ohye et al. 2014). A logical approach, therefore, is to titrate the dose of ATD to keep serum FT4 between 10 and 20 pmol/L and TSH between 1 and 3 mU/L. In this way, disease activity can be monitored. When the dose requirement falls to, say, 5 mg daily or less of Carbimazole, it may be cautiously lowered further with a view to stopping treatment altogether. If, however, a high or moderate dose of ATD is required to keep the patient euthyroid, then stopping treatment is unlikely to result in remission and should not be attempted. Since the block and replace regimen conceals the patient’s hyperthyroid status, it is necessary to stop L‐T4 periodically to assess disease activity, and this should be done before attempting to reduce and stop ATD treatment. Monitoring of TSH‐receptor antibody may also reflect disease activity. Some patients and families will struggle with adherence to ATD therapy, resulting in a poorly controlled disease which can cause considerable problems, including educational disruption. In this situation second line treatment should be implemented sooner than later (see sections ‘Radioactive iodine’ and ‘Thyroid surgery’). However, the Scottish study of Kourime et al. showed that long‐term compliance with L‐T4 was often problematic in adult patients who had received ablative treatment with surgery or radioactive iodine in adolescence. This shows that second line treatment should not be undertaken lightly. It also underlines: (i) the importance of careful and realistic counselling of families from the time of diagnosis onwards; and (ii) the need to encourage a culture of regular and disciplined tablet‐taking, given that this will be necessary in the long term in roughly 50% of patients with Graves’ disease. The goal of radioactive iodine therapy is to give a sufficient dose to ablate thyroid tissue. This treatment induces lifelong hypothyroidism, rather than remission of the hyperthyroidism, in almost all cases. High dose ablative treatment minimizes the risk of cancer, and radioactive iodine treatment is now regarded as safe in children from five years onwards. Radioiodine therapy should be conducted in collaboration with a nuclear medicine specialist or an adult endocrinologist with a special interest in thyroid disorders. Treatment is administered orally as I‐131 (or 131I) which is a radioactive isotope of iodine. 131I given as the dose of 450 megabecquerel (MBq) = 12.2 millicurie (mCi) will almost always achieve hypothyroidism within six months. Teenage girls should undergo pregnancy testing prior to 131I administration. ATD medication should be stopped five days prior to treatment and symptoms of hyperthyroidism can be treated with β‐blockers if necessary. Analgesia may be needed to treat the neck pain of radiation thyroiditis and rarely nausea may occur. The patient should be closely monitored so that treatment with L‐T4 can pre‐empt the onset of severe hypothyroidism. In the rare cases when hyperthyroidism persists beyond six months, then patients can be retreated. In the minority of children with severe eye disease, radioactive iodine should be used with caution as it may exacerbate the ophthalmopathy. Steroid treatment for several weeks may be helpful. Alternatively, surgery may be required. The main advantage of this treatment is the rapid cure of the thyrotoxicosis. It is particularly useful in young children (under 10 and particularly under 5 years of age) in whom definitive treatment is required and in those with a very large thyroid gland. Euthyroid status must be induced prior to surgery. Ten to fourteen days prior to surgery, iodides (e.g. Lugol’s solution, see Table 6.4, 0.1–0.3 ml, three times a day [tds], orally) should be administered to decrease the vascularity of the gland and inhibit release of thyroid hormone. Table 6.4 Early detection and management of neonatal thyrotoxicosis. (after Léger 2017). Reproduced with kind permission of author and Karger publications. ATA guidelines stress the importance of thyroidectomy being carried out by a ‘high‐volume thyroid surgeon’ since this will reduce the risk of complications. These include recurrent laryngeal nerve palsy with a resultant hoarse voice, hypoparathyroidism causing hypocalcaemia, unsightly keloid scar formation, and haematoma. Most surgeons favour total or near‐total thyroidectomy which will render the patient hypothyroid. Sub‐total thyroidectomy carries a risk of recurrence but can be considered in patients with a history of particularly poor tablet‐taking in whom compliance with L‐T4 replacement is likely to be problematic. This rare condition is caused by the trans‐placental transfer of maternal TSH receptor antibodies which stimulate the foetal and neonatal thyroid. The higher the thyroid stimulating immunoglobulin level in pregnancy, the greater the risk that the infant will develop thyrotoxicosis. This disease may occur in infants of mothers with active hyperthyroidism who are on treatment and in those women previously treated with radioactive iodine ablation or thyroidectomy and are currently euthyroid on L‐T4 replacement but who still have high titres of circulating thyroid‐stimulating immunoglobulin. Only 2% of infants of mothers with Graves’ disease are affected but the condition can be life‐threatening. Foetal demise can occur but this should not occur in neonates if they are appropriately treated and monitored. The foetal and neonatal management of the condition is comprehensively covered in a mini‐review by Juliane Léger (2017). Table 6.4 shows how neonatal Graves’ disease can be predicted, and thyrotoxicosis pre‐empted. Early treatment will prevent severe symptoms occurring and since the half‐life of thyroid‐stimulating immunoglobulins is approximately 12 days and resolution of the disease corresponds to their degradation, the disorder is self‐limiting over a 3–12‐week period. When the risk of neonatal Graves’ disease has not been recognized, for example, if antenatal care has been inadequate or if the past history of maternal Graves’ disease has not been elicited or its significance appreciated, the baby may present with full‐blown features of the condition. These include goitre, tachycardia, arrhythmias, hypertension, cardiac failure, increased appetite, weight loss, increased stool frequency, irritability, and exophthalmos. In these severely affected babies, initial treatment with Lugol’s solution (5% iodine, 10% potassium iodide = 130 mg iodine/ml) blocks thyroxine production, exerting an effect within 48 hours. Sedatives may be required, for example, oral chloral hydrate 30 mg/kg given eight hourly as necessary. Prednisolone, which inhibits the conversion of T4 to T3 and inhibits thyroid hormone secretion, can be used in particularly severe cases in the dose of 2 mg/kg/day orally. Heart failure may require treatment with diuretics. Outcome is usually favourable but babies should be examined for evidence of craniosynostosis and thyroid function monitored for several weeks after discharge. Neurodevelopmental follow‐up is indicated in babies in whom severe foetal and neonatal hyperthyroidism has been documented. This rare disorder has been described in over 1000 people in the world. In approximately 75% of cases there is evidence of a family history. It results from a dominant mutation in the thyroid hormone receptor β (TRβ) gene so that tissues fail to respond to thyroid hormones. This results in the body trying to compensate by the thyroid gland secreting increased amounts of T4 and T3. Blood levels of these hormones are therefore high, although the TSH level is not suppressed. Because the abnormality in the thyroid hormone receptors may vary from one tissue or organ to another, the responsiveness of the cells to the excess thyroid hormones also varies. There may be retardation of growth and a delay in the way the bones mature. Some of the cells in the brain may be relatively unresponsive so that the person has learning difficulties and an attention deficit when concentrating, although the IQ is usually normal. Other tissues continue to respond to the increased amounts of thyroid hormone and this may manifest itself as hyperactivity and tachycardia. A goitre is nearly always present. Most people with this condition have few symptoms and treatment is not usually required. The diagnosis is important as it allows appropriate family counselling. Symptoms of thyrotoxicosis, particularly tachycardia, can be treated with a β‐blocker. Very rarely an autonomous nodule or nodules, due to an activating somatic TSH receptor mutation, may cause hyperthyroidism. McCune–Albright syndrome is associated with autonomous thyroid adenomas. The nodules, which are follicular adenomas, can be diagnosed clinically and by isotope scanning and are very rarely malignant. Treatment is generally surgical. TSH‐dependent hyperthyroidism is a very rare condition which is caused by a pituitary TSH‐secreting tumour (a ‘TSHoma’). Thyroid hormone levels are elevated and the TSH is normal or raised. Neuro‐radiological (MRI) evaluation is required. Rarely activating germline mutations of the TSH receptor can be responsible for familial and sporadic cases of nonimmune hyperthyroidism. These patients may present in the neonatal period or in childhood with a goitre and suppressed TSH levels. Hyperthyroidism with a multinodular goitre occasionally occurs in patients with the McCune–Albright syndrome due to an activating mutation of the α subunit of the G protein. Thyroid cancer is rare in childhood and adolescence, accounting for ≤3% of carcinomas. Its epidemiology and management have recently been reviewed (Vaisman et al. 2011; Ross et al. 2016). Table 6.5 shows the different histological types of thyroid neoplasia. Table 6.5 Classification of thyroid neoplasia. The following points should be borne in mind: This is usually with painless and diffuse enlargement of the thyroid gland. Alternatively, the family may have noticed one or more nodules. Depending on the extent of the spread of disease there may be accompanying hoarseness, dysphagia, cervical lymph node enlargement, and lung metastasis. These include exposure to low dose irradiation (e.g. as in the 1986 Chernobyl nuclear accident), head and neck irradiation (e.g. for Hodgkin’s disease); and a family history of medullary thyroid carcinoma (MTC) (see section ‘Medullary thyroid carcinoma’). Thyroid function is normal and thyroid antibodies usually negative. Ultrasound will show thyroid texture and confirm the presence of nodules. Radioisotope scan may show areas of absent uptake. Nodules which take up radioisotope are usually benign. If there is any doubt, the case should be discussed with a clinician with appropriate experience and training, who will be able to perform fine needle aspiration (FNA). If the clinical picture is suggestive of malignancy, or if FNA result is positive or suspicious, then surgery is indicated. Surgery consists of removal of the affected lobe followed by total thyroidectomy if frozen sections confirm malignancy. Postoperative T4 therapy should lead to complete suppression of TSH so as not to stimulate tumour regrowth. Radioactive iodine treatment is also given if there is evidence of metastatic disease or distant lymph node involvement. Follow‐up consists of regular clinical assessment and measurement of thyroid function to ensure TSH suppression. Thyroglobulin is also measured since it indicates the presence or absence of functioning thyroid tissue. Ultrasound is also useful in detecting neck recurrences. The prognosis in the papillary and follicular carcinomas is very good, even in those with metastases, and life expectancy is normal. MTC is an aggressive tumour arising from the parafollicular C cells and usually secretes calcitonin and occasionally other hormones, such as adrenocorticotrophic hormone (ACTH). It is related to de novo or dominantly inherited mutations in the RET proto‐oncogene which may be isolated or associated with other tumours in one of the multiple endocrine neoplasia (MEN) syndromes. The three phenotypes are: In children with a family history of MEN2A or MEN2B in whom genetic studies have shown that the child is affected, prophylactic thyroidectomy is now recommended before the age of five years for MEN2A and before one year in MEN2B to pre‐empt the inevitable development of medullary thyroid cancer, which has a poor prognosis once it has become clinically evident. Follow‐up includes calcitonin monitoring. During adolescence the thyroid gland may become diffusely enlarged. TFTs and an autoantibody screen should be performed and, if both these are normal, the diagnosis – by elimination – is that of a colloid goitre. The goitre usually resolves spontaneously. However, in some cases the goitre can fluctuate in size and in a minority of cases results in a large nodular goitre in later life. There is no evidence that T4 treatment to suppress the TSH is helpful in colloid goitre, but this is sometimes tried if the goitre is large. Rarely, surgery is required for cosmetic reasons. In this condition, the thyroid gland is acutely inflamed because of a viral infection. There is often evidence of a recent or intercurrent upper respiratory tract infection. The patient may be febrile and the gland may be enlarged, tender, and painful. The inflammation results in leakage of preformed thyroid hormones into the circulation. TFTs may be normal but are usually elevated with symptoms and signs of hyperthyroidism. Inflammatory markers such as the ESR are raised while TPO antibody levels may be weakly positive. A radioisotope scan is sometimes performed to confirm the diagnosis and will demonstrate reduced or absent radioiodine uptake (in contrast to abnormally high uptake characteristic of Graves’ disease). Treatment is with analgesics and non‐steroidal anti‐inflammatory drugs but, in severe cases, steroids may be needed. A beta blocker may also be necessary to manage symptoms. Antithyroid medication is not indicated. Hyperthyroidism usually lasts for one to four weeks and may be followed by a period of hypothyroidism as the gland recovers. The total course of the illness is two to nine months with most patients making a complete recovery but, occasionally, permanent hypothyroidism may occur. Transitional clinics specifically for children with thyroid disorders are rare. More commonly the patient may be seen in an adolescent endocrine clinic. After the age of 16 years, males with congenital and acquired hypothyroidism can be discharged to the care of their general practitioner (family doctor). Most will just require annual reviews with annual TFT’s. In the case of girls with hypothyroidism, in whom periconceptual adherence to L‐T4 treatment is important for foetal well‐being, there is a case for transfer to adult services and supervision/counselling into early adulthood. Adolescents with active thyrotoxicosis which is still requiring medical treatment are best transferred from paediatric to adult care at a suitable age (16–18 years). In patients who have undergone second line treatment with radioactive iodine or surgery, it is reasonable to refer back to the general practitioner once the patient has been stabilized on T4 and has normal TFTs. Patients should be informed about the risk of neonatal Graves’ disease in the event of a future pregnancy. Adolescents treated for thyroid cancer should remain under follow‐up by an endocrinologist.
Thyroid Disorders
Embryology, anatomy, and physiology of the thyroid gland
Embryology and anatomy
Physiology
Control of thyroid metabolism
T4 synthesis
T4 metabolism
Action of the thyroid hormones
Foetal and neonatal thyroid metabolism
Foetal thyroid metabolism
Thyroid function in term neonates
Thyroid function in preterm neonates
Thyroid function tests (TFTs)
Newborn period
TSH
1.2–10.5 mU/L
Free T4
15–40 pmol/L
1.17–3.1
Thyroglobulin (cord blood)
11–127 μg/L
Thyroglobulin (day 3)
62–400 μg/L1
Antibody tests – all ages
TSH
0.5–5.5 mU/L
Free T4
9–26 pmol/L
0.79–2.0 ng/dL
Total T4
80–160 nmol/L
6.2–12.4 μg/L
Free T3
4.0–7.5 pmol/L
2.6–4.9 pg/ml
Total T3
1.5–3.4 nmol/L
97.6–221.3 ng/dL
Antibody tests
TPO antibodies
<35 mU/L
TSH receptor antibodies
≤14 u/L (Thybia assay)
Definition and classification of thyroid disorders
Neonatal hypothyroxinaemia
Hyperthyrotropinaemia
Transient neonatal hypothyroidism
Primary congenital hypothyroidism
Permanent, non‐syndromic
Thyroid dysgenesis (75%)
Ectopia (40%)
Athyreosis (30%)
True (thyroglobulin undetectable)
Apparent (thyroglobulin detectable)
Hypoplasia in situ (5%)
Thyroid dyshormonogenesis (20%)
Autosomal recessively inherited defects within structurally normal thyroid gland due to mutations in genes encoding:
Thyroglobulin
Thyroid peroxidase
Pendrin (Pendred syndrome)
Dual oxidase 2;dual oxidase
maturation factor 2
Dehalogenase system
Rare causes (<5%)
Transplacental transfer of thyroid peroxidase or thyrotropin receptor blocking antibody
Maternal radio‐iodine exposure
Permanent syndromic
Thyroid dysgenesis
Mutations in:
GNAS (Albright’s hereditary osteodystropy)
PAX8 (with kidney agenesis/other genito‐urinary malformations)
TTF‐1/NKX2–1 (with lung disorders; choreoathetosis)
TTF‐2/FOXE1 mutation (with cleft palate, spiky hair)
Thyroid dyshormonogenesis
Mutation in Pendrin gene with goitre and deafness (Pendred’s syndrome)
Transient
Maternal causes
Foetal abnormalities
Congenital abnormalities
Syndromic disorders including Down syndrome
Postnatal problems
Perinatal illness (e.g. hypoxia, sepsis, exchange transfusion)
Iodine exposure
Central congenital hypothyroidism
Permanent
Idiopathic with other pituitary deficiencies isolated
Congenital hypothyroidism
Aetiology
Thyroid dysgenesis
Thyroid dyshormonogenesis
Thyroid screening in the newborn
Background
Type and timing of sampling
Screening tests
Protocol for notification of positive results
Second screening for preterm infants, sick neonates, and in multiple births
Point‐of‐care testing
Clinical assessment of infants with suspected congenital hypothyroidism
History
Signs
Initial investigation of infants with TSH elevation
Biochemical
Imaging
X‐ray of knee
Thyroid imaging
Treatment of congenital hypothyroidism
Neonatal period
Infancy
Beyond infancy
Age
L‐T4 dosage guide (μg)
Clinic frequency
Growth monitoring
Assessment/education
Birth–1 mo
See text
Weekly
Length, weight, OFC
Initial investigations including imaging; L‐T4 dose titration; counselling
1–6 mo
37.5
2–4 wk
Length, weight, OFC
Consolidation of family education/counselling
Neurodevelopment
6–12 mo
37.5–50
4–8 wk
Length, weight, OFC
1–3 yr
50–75
4–6 mo
Height, weight, OFC
Neurodevelopment
Preschool audiology
3–16
75–150
Annuallya
Height and weight (pubertal staging not normally necessary)
Child and adolescent education including pre‐conceptual counselling in girls
Follow‐up and retesting in selected cases
Follow‐up
Re‐evaluation of thyroid status
Outcome
Influence of prenatal hypothyroidism on outcome
Influence of adequacy of postnatal treatment on outcome
Influence of over‐treatment on behaviour and attention
Acquired hypothyroidism
Aetiology
Iodine deficiency
Autoimmune (hashimoto’s) thyroiditis
Miscellaneous causes
Clinical features
History
Signs
Investigations
Treatment
Follow‐up
Outcome
Hyperthyroidism
Aetiology
Hyperthyroidism due to Graves’ disease and Hashimoto’s thyroiditis
Hashimoto’s thyroiditis
Graves’ or Hashimoto’s disease?
Clinical features of hyperthyroidism in children and adolescents
History
Examination
Diagnosis
Treatment of hyperthyroidism in children and adolescents
Initial medical treatment
Dose titration regimen
Block and replace regimen
Dose titration or block and replace?
Adverse effects of anti‐thyroid drugs
Management of graves’ ophthalmopathy
Duration of treatment with antithyroid drugs
Radioactive iodine
Thyroid surgery
Check TSH receptor antibody (TRAB) titre in 3rd trimester of pregnancy in women with a history of Graves’ disease, past or present. This includes mothers in whom ablative treatment has been carried out previously.
If maternal TRAB titres are undetectable no further action is needed.
If maternal TRAB is above the laboratory reference range neonatal follow up is required
Neonatal thyrotoxicosis is likely if maternal TRAB is x 2–3 above normal
In babies of mothers with detectable TRAB
Check cord blood for TRAB, FT4 and TSH
Check thyroid function days 3, 5, 7, 10, and 15 (keep baby in hospital for first seven days)
Examine for evidence of thyrotoxicosis (tachycardia, excessive crying, goitre)
If biochemical evidence of thyrotoxicosis (FT4 > 30 pmol/l, TSH <0.1 mU l−1 ± symptoms and signs:
Give Carbimazole or Methimazole 250 μg kg−1 three times daily initially
Propranolol 1 mg kg−1 twice daily for 2 wk (monitor pulse, blood pressure and blood glucose)
Titrate Carbimazole dose to keep FT4 ~15–20 pmol/l
Check TRAB monthly and stop Carbimazole when titres become undetectable (usually within 3 months)
Neonatal thyrotoxicosis
Other causes of hyperthyroidism
Thyroid hormone resistance syndrome
Autonomous nodules
TSH‐dependent hyperthyroidism
Activating mutations of the TSH receptor
Thyroid neoplasia
Follicular tumours
Papillary carcinoma
Follicular adenoma
Follicular carcinoma
Anaplastic carcinoma
Non‐follicular tumours
Medullary carcinoma
Lymphoma
Teratoma
Metastatic
Miscellaneous
Presentation
Risk factors
Investigation
Treatment
Medullary thyroid carcinoma
Miscellaneous disorders
Colloid (simple) goitre
Subacute thyroiditis
Transition
When to involve a specialist centre
Future developments
Controversial points
Common pitfalls
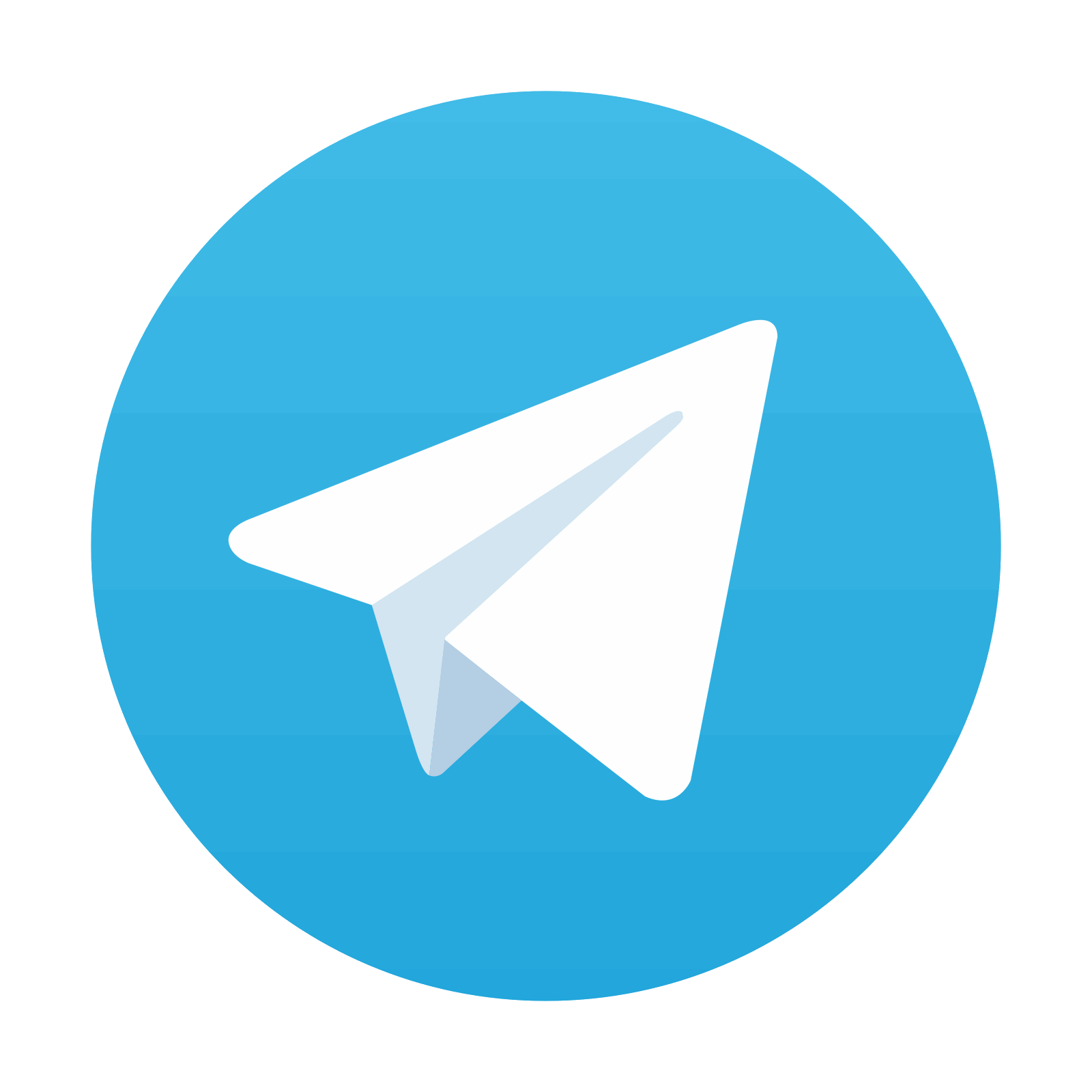
Stay updated, free articles. Join our Telegram channel

Full access? Get Clinical Tree
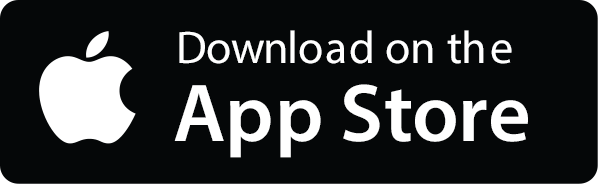
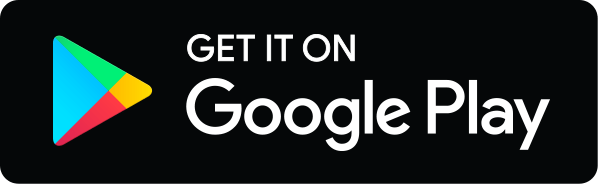