Fig. 10.1
Systemic thromboembolic conditions in children and adults. TTP thrombotic thrombocytopenic purpura, ECMO extracorporeal membrane oxygenation, TAFI thrombin-activatable fibrinolysis inhibitor, TFPI tissue factor pathway inhibitor
10.2 Inherited Thrombophilia and Genetic Predisposition to Thrombosis
Thromboembolic events were less commonly reported in children than adults. The recent increase of pediatric thrombosis is attributed to advanced critical care and improved survival of high-risk infants. During three decades, neonatal managements have greatly improved the survival of preterm infants. Newborn infants are originally prone to thromboembolic events because of the physiologic hypercoagulability of the developing hemostatic system [11]. Sick preterm newborns all need central venous lines which run a high risk of VTE. The prothrombotic conditions may be augmented in the newborns harboring a heterozygous mutation of PC gene [12].
Thrombophilia is a disease entity to define severe congenital deficiency of PC, PS, and AT, for the analogy of hemophilia. On the other hand, persons having the heterogeneous mutation do not always experience thromboembolic events, and most of the patients develop thromboembolism over the mid-40s. Taken into account the distinct penetrance, genetic predisposition/diathesis to thrombosis is the precise nature of adult-onset but not pediatric-onset heritable thrombophilia.
10.2.1 Antithrombin (AT) Deficiency
AT is a plasma protein synthesized by the liver. A mutation in the AT gene (SERPINC1) located on chromosome 1q23-25 was identified in the first reported family with AT deficiency [13, 14]. The anticoagulant factor binds and neutralizes the serine proteases of thrombin, factor Xa, and factor IXa, which are generated by the coagulation cascade. The AT-mediated effects on the inhibition of these proteases are enhanced by binding to heparin. AT has two major active sites, the reactive center toward the carboxyl terminus and the heparin-binding site at the amino terminus. Thrombin cleaves the reactive site, and the inactive complex molecules are then cleared from circulation. Heterozygotes for AT deficiency are found in about 1% of patients having the first episode of deep venous thrombosis (DVT). The thrombotic risk of inherited AT-, PS-, or PC-deficient patients is higher than that of FV Leiden or PTII variant even in infants and children (Table 10.1). They have an eight- to tenfold higher risk for thrombosis than noncarriers [4, 15, 16]. More than 50% of the first-degree relatives of each deficient patients experience VTE until the first 25 years of life [17]. The first thrombotic episode of AT deficiency occurs in more than 40% of patients spontaneously. The remaining patients have some triggers including pregnancy, parturition, contraceptive use, surgery, or trauma.
Table 10.1
Reported risk of thromboembolism in thrombophilic children
Genetic predisposition | Relative risk (odds ratio) | [95% conf. interval] | |
---|---|---|---|
Cerebrovascular occlusion (first) | Deep vein thrombosis | ||
First attack | Recurrence | ||
Protein C deficiency | 9.3 [4.8–18.0] | 7.7 [4.4–13.4] | 2.4 [1.2–4.4] |
Protein S deficiency | 3.2 [1.2–8.4] | 5.8 [3.0–11.0] | 3.1 [1.5–6.5] |
Antithrombin deficiency | 7.1 [2.4–22.4] | 9.4 [3.3–26.7] | 3.0 [1.4–6.3] |
Factor V G1691A | 3.3 [2.6–4.1] | 3.6 [3.8–4.8] | 1.4 [0.4–1.2] |
Factor II G20210A | 2.4 [1.7–3.5] | 2.6 [1.6–4.4] | 2.1 [1.01–3.5] |
Lipoprotein (a) | 6.5 [4.5–8.7] | 4.5 [3.3–6.2] | 0.8 [0.5–1.4] |
LA/aPL | 6.6 [3.5–12.4] | 4.9 [2.2–10.9] | |
More than 3 | 11.9 [5.9–23.7] | 9.5 [4.9–18.4] | 4.5 [4.5–6.9] |
The odds ratio (OR) of thrombosis with AT deficiency is estimated to be 9.4, although the risk depends on the population selected (Table 10.1) [4]. The prevalence of AT deficiency was 1.1%, and the OR for thrombosis was 5.0 according to the Leiden Thrombophilia Study [18]. Heritable AT deficiency is an autosomal dominant disease with equal sex distribution. Common affected sites of thrombosis include deep veins in the legs, mesenteric veins, and pulmonary embolism. Complete AT defects due to null mutation lead to the fetal loss. Therefore, neonatal purpura fulminans is not the regular presentation of AT deficiency. Recurrent thromboses occur in approximately 60% of patients. AT deficiency may also be associated with perinatal, neonatal, and childhood arterial ischemic stroke. Cerebral venous sinus thrombosis (CVST) is the other presentation of AT deficiency. Although AT-deficient children are mostly asymptomatic before puberty, the substantial risk of thrombosis increases with age.
Heritable AT deficiency is classified into two types. Type I-deficient individuals show paralleled reduction of both antigen and activity levels of AT, representing a reduced synthesis of biologically normal protease inhibitor molecules. AT deficiency arises from mostly point mutations of SERPINC1 and rarely a deletion encompassing the gene. More than 100 mutations have been reported in type I-deficient patients [19]. Type II-deficient subjects show reduced levels of plasma activity but normal antigen levels of AT, representing a discrete molecular defect. Type I deficiency is subdivided into Ia (normal) and Ib (reduced), based on the heparin affinity. Type II deficiency is subcategorized into three groups assessed by progressive AT assays and heparin-binding assays, the molecular abnormality in reactive site (RS, 393Arg-394Ser), heparin-binding site (helices A and D) (HBS), and PE (pleiotropic effect). Type Ib is now designated as type II PE, because of the pleiotropic abnormalities affecting the reactive site, the heparin-binding site, and plasma concentration.
Type II HBS variants are infrequently associated with thrombotic events. The homozygotes were, however, reported in young children with recurrent thromboses born to consanguineous healthy parents carrying type II HBS. The prevalence of type I AT deficiency, estimated to be 1 in 2000 adults [20], rises to 1 in 250–500 healthy blood donors screened by heparin cofactor activity [21]. The mean concentration of AT in normal pooled plasma is about 12.5 mg/dL. The standard value ranges between 75 and 120% of normal pooled plasma for AT-heparin cofactor determinations. Circulating AT levels decrease due to consumption in cases of sepsis, burns, and disseminated intravascular coagulation (DIC), but less acute thrombosis. Reduced AT levels are ascribed to impaired production and loss in liver disease and nephrotic syndrome, respectively. Hormonal effects (oral contraceptives or estrogens) and heparinization reduce plasma AT levels. The physiologically low AT levels in healthy newborns gradually reach the adult levels by 6 months of age [11, 22]. Preterm infants show much lower AT levels than term ones, the levels of which are further depressed in respiratory distress syndrome, sepsis, necrotizing enterocolitis, and DIC. The levels of alpha-2-macroglobulin, which is a second thrombin inhibitor and also an acute phase protein, are higher in children than adults. It reduces the chance of pediatric thrombosis especially in infection. The family members having affected proband need a correct diagnosis for genetic counseling, replacement therapy, and prophylaxis in daily life.
10.2.2 Protein C (PC) Deficiency
PC is a vitamin K-dependent anticoagulant factor, which is synthesized by the liver and circulates as a zymogen. It exerts the proper anticoagulant function after the cleavage of the molecule to the serine protease, activated PC (APC). This activation is mediated by thrombin alone, but occurs more efficiently when thrombin is bound to thrombomodulin on the endothelial cell receptor. APC inactivates FV and FVIII by cleaving the critical sites of these activated molecules. This reaction is enhanced by PS, FV, and lipid cofactors of lipoproteins and phospholipids. Reduced concentration of circulating PC fails to control the propagation of thrombin generation by FVa and FVIIIa, even if PC deficiency originates from the decreased production and/or increased consumption. APC has anti-inflammatory and cytoprotective functions [23]. These are directly driven by the endothelial and immunocompetent cells via the specific receptor on the given cells. The protective effect of APC in animal models of sepsis depends on its capacity to activate protease-activated receptor-1 (PAR1) and not on its anticoagulant properties. The pleiotropic effects were expected for the treatment of sepsis. However, clinical trials of recombinant APC were withdrawn for the risk of bleeding [24]. Refined molecules of APC are being explored for the effective treatment of sepsis and DIC [25].
Plasma levels of PC activity are assessed by chromogenic (amidolytic) or coagulometric (clotting) assays. The standard value in healthy adults ranges from 0.65 to 1.35 IU/mL (65–135% of normal). “Mild,” “moderately severe,” and “severe” PC deficiencies are defined as the range of >20% (>0.2 IU/mL), 1–20% (0.01–0.2 IU/mL), and <1% (<0.01 IU/mL), respectively [22]. PC gene (PROC) is located on chromosome 2q13-q14. The first report of PC deficiency was a 22-year-old Caucasian with recurrent thrombophlebitis and pulmonary embolism [26]. Heterozygous PROC mutation inherits “mild” or at most “moderately severe” PC deficiency in an autosomal dominant manner. The first presentation of PC deficiency includes the newborn-onset and the teens-onset modes. Neonatal purpura fulminans and stroke are the proper expression of PC deficiency [27, 28]. Thereafter, DVT in the legs and pulmonary thromboembolism are commonly affected sites. Approximately 70% of patients first present in teens spontaneously and the remaining 30% with the risk factors. Nonhemorrhagic arterial stroke is associated with pediatric PC deficiency. PC deficiency is the major heritable thrombophilia in Japanese children [29], while PS deficiency is that in the adult cases [30, 31].
Severe PC deficiency is inherited in an autosomal recessive fashion. The complete defects exclusively arise from biallelic PROC mutation, presenting neonatal purpura fulminans and/or intracranial hemorrhage. It is hard to differentiate in infancy between primary and secondary forms of PC deficiency, because of the physiologically low activity. Both antigen and activity levels increase after birth and reach the lower limit of adult references (~50 IU/dL) during 6 months to 1 year of age [11, 32]. PC levels increase in adolescence and further do 4% per decade in adults. Standard PC activity has wide ranges than PS activity during the first 7 days after birth. PC levels in preterm infants are much lower than those in term infants. Acquired PC deficiency occurs in the settings of neonatal asphyxia, liver disease, sepsis, and DIC, along with acute thrombosis. Newborn infants are prone to vitamin K deficiency bleeding. For the genetic screening, PC activity levels should be followed concurrently with PS activity, protein induced by vitamin K absence or antagonists II (PIVKAII), D-dimer, and antiphospholipid antibodies, along with FVII activity. Unexplained dissociation between PC and PS activity levels portends a diagnosis of heritable PC deficiency [28, 33].
The prevalence of PC deficiency was initially estimated between 1 in 16,000 and 1 in 32,000 within the general population, based on the assumptions that the autosomal dominant disorder shows more than 50% of penetrance. However, the parents of infants with neonatal purpura fulminans rarely experience thrombotic events. Each parent has a heterozygous PROC mutation resulting in the modest effect on the thrombotic activity. The prevalence of heterozygosity for PC deficiency is considered to be 1/200–500 [34]. Heterozygous PC-deficient adults have a sevenfold increased risk for an initial episode of DVT compared with those having normal PC activity. On the other hand, asymptomatic carriers have low annual incidence of thrombosis at less than 1.0%. The OR of pediatric thrombosis with PC deficiency is estimated at 7.7 [4] (Table 10.1).
Heterozygous PC deficiency is classified into two types. The common form of type I represents an equally reduction in both immunologic and biologic activities. More than 200 mutations and rarely deletions were identified [35]. Type II-deficient subjects show normal antigen and decreased activity levels. However, several cases show normal levels of antigen and amidolytic activity, but with reduced levels of clotting activity. It may be ascribed to a reduced ability of APC to interact with the platelet membrane or its substrates of FVa and FVIIIa. The coagulant assay has then high sensitivity in screening for PC deficiency. Nevertheless, repeated studies are needed to clarify the mutation spectrum. Recently, K193del has been recognized as the most common variant in Chinese thromboembolisms [36]. On the other hand, it was considered as a polymorphism in Japan, because the heterozygotes often show normal activity for PC in resting conditions [37]. It was originally reported as PC-Tottori, in the homozygote that developed DVT for the first time at 28 years of age [38]. Based on our observations [12], age-dependent increase of PC activity seems to be delayed in the heterozygote infants for PC-Tottori. There is increasing number of reports in Asian countries on the patients with double mutations who escaped neonatal thromboses. In Caucasians, FV Leiden and FII variant might mask the effects of PROC variants on the thrombotic risk of patients [39].
PC activity is recommended to assess with the concurrent measurements of FVII, a vitamin K-dependent zymogen with a similar short plasma half-life, to exclude the effects of consumption coagulopathy. Discontinuation of oral warfarin therapy is needed to screen the baseline PC (and PS) activity. However, heparin is substituted in patients who cannot be stopped for anticoagulant therapy because of severe thrombotic diathesis. It occurs a few days after the start of warfarin therapy, in association with the initial loading doses. Erythematous macules progressively expand with the necrosis on the trunk and extremities over several hours. The clinical setting and histopathology (fibrin thrombi and interstitial hemorrhage) share those of neonatal purpura fulminans. Even the initial standard dose of warfarin reduces PC activity to about half of the normal within 1 day. FVII levels decline followed by the decreased activity of other vitamin K-dependent factors according to the half-lives. The half-life of plasma PC (6–8 h) is shorter than that of PS and other procoagulant vitamin K-dependent factors. The enzymatic activity of PS depends on free PS concentration. Acute inflammation reduces PS activity due to binding with C4b. Warfarin-induced skin necrosis is a noticeable complication in PC- or PS-deficient cases with infection. On the other hand, neonatal purpura fulminans has been exclusively reported in PC deficiency. It may be explained by not only the shorter half-life of PC but also the low binding capacity of C4b to PS at birth. Replacement of PC concentrates is essential for the treatment of purpura fulminans [40–43].
PC and PS levels are influenced in diverse conditions throughout childhood [35]. Vitamin K antagonists reduce the levels of PC and PS activities. Vitamin K deficiency precipitates bleeding (i.e., hemorrhagic diseases of the newborn, vitamin K deficiency bleeding in infancy) and also thrombosis (i.e., warfarin-induced skin necrosis). Severe infections lower the plasma activity of natural anticoagulants. The mechanisms of “infectious purpura fulminans” are involved in antibody-mediated coagulopathy (i.e., post-varicella purpura fulminans) or toxic effects (i.e., meningococcemia purpura fulminans) associated with acquired PC/PS deficiency. PC or PS deficiency arises from the loss or consumption in patients with nephrotic syndrome, sepsis, and/or DIC and from the impaired synthesis in those with liver dysfunction. In contrast to AT, antigenic concentrations of vitamin K-dependent plasma proteins are elevated in nephrotic syndrome. Uremic patients have low anticoagulant activity, but normal amidolytic activity and antigen levels for PC, because of the interfering dialyzable moiety with clotting PC activity. Warfarin therapy reduces functional and, to a lesser extent, immunologic assays of PC. These conditions complicate the diagnosis of PC deficiency.
10.2.3 Protein S (PS) Deficiency
PS is a vitamin K-dependent protein that enhances the anticoagulant effect of APC. This coenzyme is primarily synthesized by hepatocytes and also by endothelial cells, megakaryocytes, and brain cells. It serves as a cofactor for APC in the setting of FVa and FVIIIla inactivation. The inactivation of FVa occurs, at first, in the rapid cleavage at Arg506 (FV Leiden, Gln506) of the molecules, followed by the slower cleavage at Arg306 (second binding site of PS with APC) and then Arg679 [44]. PS with APC increases the affinity for phospholipids to enhance FVa inactivation. Approximately 40% of circulating PS molecules is in the free form, and the remainder 60% is bound to C4b-binding protein (C4BP) not to interact with APC. Free PS levels are responsible for the direct anticoagulant effects.
PS deficiency was first described in 1984 in the families with low PS activity and recurrent thrombosis [45]. Heterozygous mutations in the gene encoding protein S (PROS1) on chromosome 3q11 cause autosomal dominant thrombophilia. Homozygotes or compound heterozygotes for the mutation are quite rarely found as an autosomal dominant thrombophilia. However, most reported cases of neonatal purpura fulminans had PC but not PS deficiency [22, 28]. It may account for the relative increase of free PS concentrations (by physiologically low levels of C4BP) and the narrower ranged activity and shorter half-life of PS during the early neonatal period.
PS deficiency confers a risk of thrombosis similarly to PC deficiency. The clinical findings in the heterozygotes for PS deficiency are similar to those for AT or PC deficiency. Common affected sites are DVT and pulmonary emboli. The first thrombotic event in PS deficient patients occurs at approximately 25–30 years of age. More than half of the episodes were spontaneous, and the remainder were associated with identifiable factors. In pediatric cases, the OR for venous thrombosis due to PS deficiency is estimated to be 5.77 (Table 10.1) [4]. Several reports suggest an association between PS deficiency and arterial thromboses including ischemic stroke in infants and children [46].
On the other hand, adults with low PS levels but no family history of venous thrombosis have minimal risk of VTE. Low free PS or total PS levels (both <0.10th percentile) were not associated with an increased risk of VTE. Young patients with recurrent VTE are associated with double mutations of PROS1. Their parents were reportedly asymptomatic although they carried type I PS deficiency. PS deficiency is classified into three types, according to the levels of total and free antigens, along with functional activity. Type I deficiency shows about half levels of normal PS antigen, although free PS antigen and functional activity levels are greatly reduced [47]. Most patients have the missense mutations and base pair insertions. Type II is a qualitative deficiency with normal levels of total antigen and free PS, but impaired function. The rarity of type II-deficient patients indicates the insufficient screening power of functional assays. Type III is characterized by normal levels of total PS antigen, but reduced levels of free PS and functional activity. Plasma activity levels rather than antigen levels are then preferable for screening PS deficiency. Although the biologic basis of type III PS deficiency remains unclear, PROS1 mutations were responsible for the type I deficiency, but not the type III phenotype of age-dependent free PS deficiency [48]. The prevalence of PS deficiency depends on the ethnicity. In Asian countries, PC and PS deficiencies are dominantly reported in adults and children with thrombosis [49–51]. PS-Tokushima K196E is found in one per 55 healthy Japanese adults as a common variant for thrombosis in Japan [52].
The lower limit of plasma levels of total and free PS (~65%) in heterozygous deficiency considerably overlaps the ranges of healthy controls [28]. PS levels are lower in female than male and increase with advanced age. PS, PC, and AT levels are reduced in inflammation. Because C4BP is an acute phase reactant, it shifts PS to the complexed inactive form leading to the reduced PS activity. On the other hand, PS but not PC levels decrease in pregnancy. In cases of nephrotic syndrome, the balance of elevated C4BP concentrations and loss of free PS reduce the functional activity levels despite the increased antigen levels. Repeat sampling, family studies, and genetic tests are required to make the correct diagnosis for pediatric thrombophilia.
10.2.4 Factor V Leiden
The resistance to APC in an activated partial thromboplastin time (APTT)-based clotting assay accounts for familial thrombophilia [53]. The major genotype of APC resistance is FV Leiden (Arg506Gln) [54], being found in more than 80% of APC resistant patients. In Western countries, FV Leiden was found in 20–50% of adult patients with VTE. FV Leiden is a risk factor for venous and arterial thrombosis. Retrospectively, heterozygosity for FV Leiden was identified in 12% of patients who had a first episode of DVT or pulmonary embolism and in 6% of controls. In the elderly (>60 years of age) who suffered from the first VTE without triggers, 26% were heterozygotes for FV Leiden. Pediatric studies found that FV Leiden heterozygosity conferred an odds ratio of 3.6 for a first episode of VTE and 1.4 for recurrent VTE in children [4] (Table 10.1). The OR for pediatric CVST in FV Leiden carriers was 2.74. On the other hand, the annual incidence of VTE in asymptomatic carriers of the FV Leiden is low (0.58%), which raises questions about the screening asymptomatic family members [55]. FV Leiden was not associated with the risk of myocardial infarction or stroke, but potentially perinatal and pediatric arterial ischemic stroke. FV Leiden homozygotes may have the similar high risk of VTE to the heterozygotes for AT, PC, or PS deficiency. As FV and AT genes are located on chromosome 1p, the coinheritance of FV Leiden and AT mutations leads to a more severe thrombotic diathesis.
Heritable thrombophilias including FV Leiden are associated with a tripling risk for the late fetal loss, but no risk for the first-trimester loss. High risk of thrombophilias was reported in association with obstetric complications other than preeclampsia and intrauterine growth retardation. The prevalence of heterozygosity for FV Leiden ranges 1–9% in Caucasians, but quite rare (<1%) in African blacks, Chinese, Japanese, or Native American ancestries. FV Leiden is more prevalent in northern Europe than the southern countries. A single founder allele was suggested among whites of differing ethnic backgrounds. Severe infections might shape the distribution of FV Leiden, because of the survival advantage inferred by the mice models. Co-segregation of heterozygous APC resistance due to FV Leiden and type I FV deficiency results in severe APC resistance in APTT assays, as found in homozygous FV Leiden patients (pseudohomozygous). Several polymorphisms in FV gene include a haplotype (HR2) containing the R2 polymorphism (Hisl299Arg) with mild APC resistance. Although FV Leiden was not found in Japan, FV Nara showing APC resistance has been recently identified in a Japanese family with recurrent thrombosis [56].
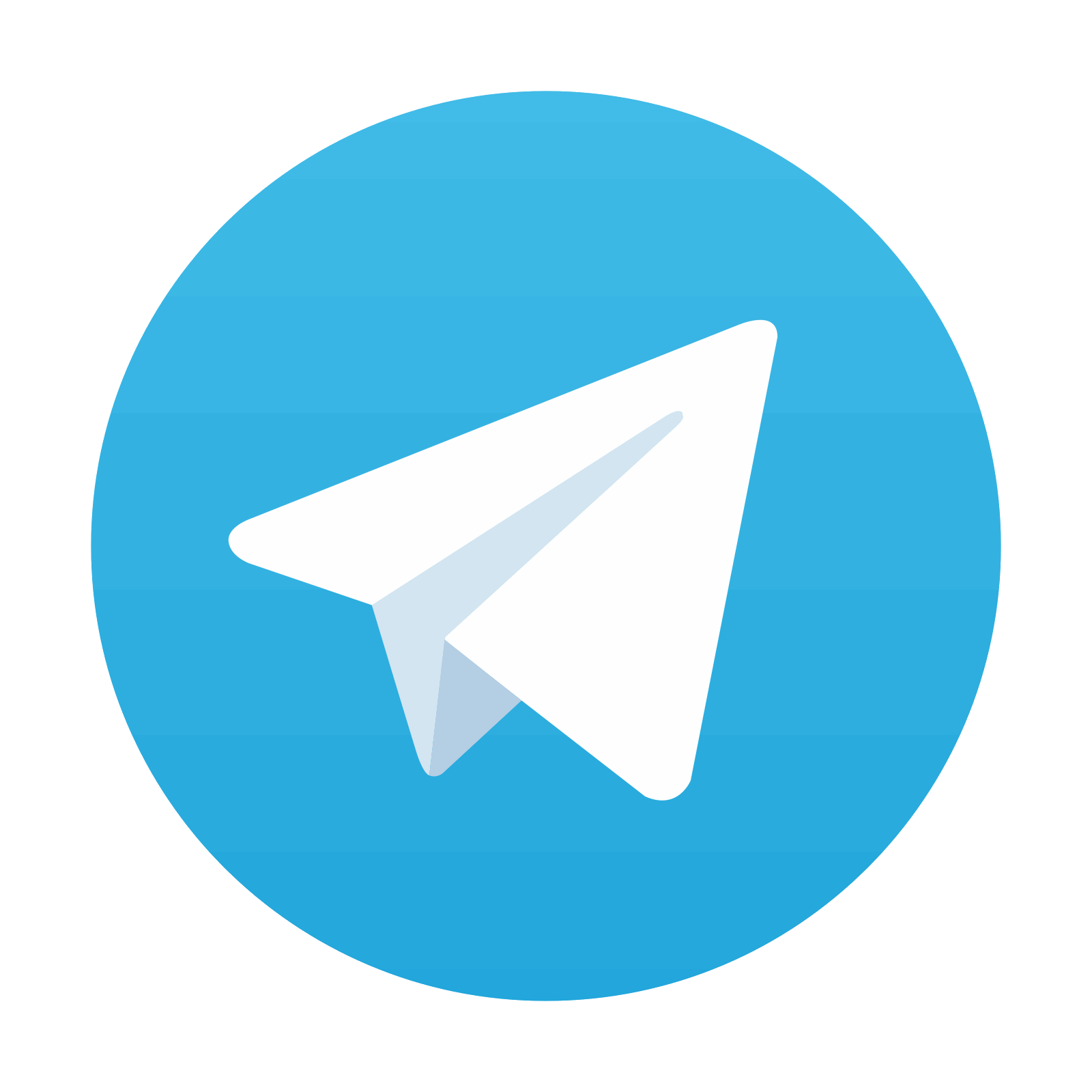
Stay updated, free articles. Join our Telegram channel

Full access? Get Clinical Tree
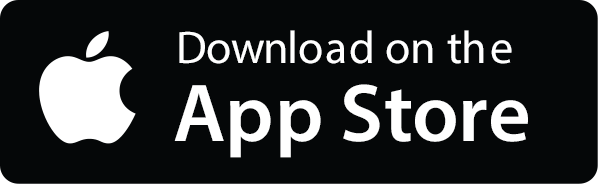
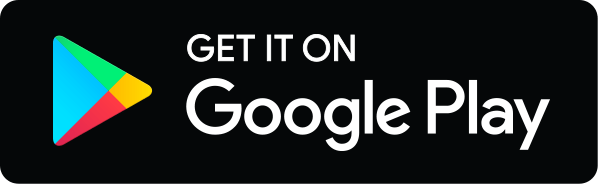