© Springer Science+Business Media New York 2017
William B. Coleman and Gregory J. Tsongalis (eds.)The Molecular Basis of Human Cancer10.1007/978-1-59745-458-2_2727. The Molecular Basis of Prostate Carcinogenesis
(1)
Department of Urology and Cancer Center, University of California – Davis, Sacramento, CA, USA
Keywords
Prostate carcinogenesisProstate cancerEpidemiologyGenetic factorsFamilial cancerProstate-specific antigen testTransrectal ultrasoundNeedle biopsyLuminal cellsBasal cellsGlandular tissuesProstatitis27.1 Introduction
This review on prostate cancer is an attempt to update the reader on the current information we have on the disease. There are no definite cures yet for prostate cancer and the exact mechanism of development is far from clear. However, over the years, the information obtained on the disease has increased due to the unceasing flow of research data. In the following pages, you will be briefed on the basic characteristics of prostate cancer, the characterization of the genomic regions affected, the candidate genes involved, the secondary sites of metastases such as bone and the models that have been developed to investigate the disease in detail.
27.2 Epidemiology of Prostate Cancer
Langstaff reported the first case of prostate cancer in 1817 followed by J. Adams, a surgeon in London, who examined the cancer histologically [1, 2]. Prostate cancer is a non-skin cancer that is the cause of the second largest number of male-related deaths in the countries of the West [3]. It is known as the cancer of the elderly as older men over the age of 65 are diagnosed with cancer of the prostate [4]. This is probably why many men die with the cancer rather than due to it [4, 5]. This is observed in the case of asymptomatic sporadic prostate cancer which account for >90 % of the disease [6]. Hereditary and familial prostate cancer, on the other hand, is observed in younger males and is dependent on family history [5]. In 2007, the statistics in CA Cancer Journal for Physicians predict that prostate cancer accounts for ~218,885 new cases and ~27,350 deaths [7, 8].
27.3 Risk Factors for Prostate Cancer
There is no single cause of prostate cancer, but it primarily arises due to aging, discrepancies in sex hormone levels, genetic factors, and environmental agents [4, 9].
27.3.1 Aging
Prostate cancer is known as a cancer of the elderly for the main purpose that tumors arise with increase in age of men [10]. There is a logarithmic increase in malignant potential with increase in age. Prostate cancer has a long natural history and develops very slowly which might account for the delayed appearance of the tumor in the life of an individual. In most cases, carcinoma of the prostate rarely sees the light of day during the lifetime of an individual and is observed during an autopsy. Latent cancers of the prostate and benign prostatic hyperplasia (BPH) are observed more frequently, and differ from the few cancers that develop malignant potential. Surgery is the most common form of treatment to remove BPH [10, 11]. Another hypothesis for the contribution of aging to prostate cancer is the shortening of telomere sequences and a subsequent increase in the enzyme telomerase which can be observed in prostatic intraepithelial neoplasia (PIN) and carcinomas [9]. Carcinoma arises predominantly from the peripheral zone compared to the central zone of the prostate [11].
27.3.2 Hormones
Hormones are an integral part of the prostate as they regulate its growth, differentiation, and maintenance. Androgenic hormones such as dihydrotestosterone are found in the prostate. Testosterone diffuses into the prostate and is converted to dihydrotestosterone in the prostate which is the major form of androgen metabolized in the prostate. Prostate cancer shows high levels of androgens when first diagnosed and androgen ablation therapy is commonly used to counter the growth of the cancer which results in the blockade of the androgen supply. However, it has not been confirmed that androgenic hormones are the cause of prostate cancer [4, 12, 13].
27.3.3 Genetic Factors
Hereditary and familial cancer account for ~9 % of prostate cancer cases [4, 6, 9]. Prostate cancer is hereditary when there are at least three first-degree relatives or three consecutive generations or two or more relatives (at a young age) affected by the disease. The loci and the candidate genes affected in hereditary prostate cancer differ from those affected in sporadic cancer [6]. Linkage analysis of the hereditary prostate cancer families identified 6 loci that are associated with the disease. HPC1 or hereditary prostate cancer gene 1 is the name given to one of the loci, 1q24–25, and the gene that maps to the HPC1 locus is called RNASEL . In addition the other loci affected are 1p36 (gene CAPB), 1q42.2–43 (PCAP), 8p22–23 (MSR1), 17p11 (HPC2), 20q13 (HPC20), and Xp27–28 (HPCX) [6, 14, 15]. The two main candidate genes in the list are RNASEL and MSR1. RNASEL encodes an endoribonuclease involved in the proapoptotic activity of the interferon induced 2-5A system. Mutation in RNASEL reduces the enzymatic activity. MSR1 is a macrophage specific receptor binding to Gram-positive and Gram-negative bacteria. It is difficult to distinguish hereditary cancer from sporadic cancer especially when dealing with families that have a high penetrance of prostate cancer. The detection of hereditary cancer only in older patients prevents the simultaneous identification of the cancer in a younger generation to confirm the hereditary aspects of the disease [15]. Sporadic prostate cancer accounts for >90 % of prostate cancer. The chromosomal loci involved in sporadic prostate cancer differ from those affecting hereditary cases. The loss of chromosomal loci indicates the loss of potential candidate genes which may be tumor suppressor genes. These genes encode proteins that function as hormone receptors, cell-signaling molecules, and cell adhesion factors. Some of the loci that are affected are 3p22–26, 5q22, 10q23, 13q14, 18q21, and others.
27.3.4 Diet
Dietary factors are known to affect the incidence of prostate cancer. It has been shown that Asian men are less likely to develop prostate cancer compared to their counterparts in the Western countries [1, 4]. The incidence of prostate cancer in African-American males is 15 % compared to 10 % in Caucasian-American males. The incidence of prostate cancer is 120 times lower in Asian men [16]. This is due to differences in diet in the two parts of the world. In addition, it has also been observed that when Asian men migrate to the west, there is an increase in prostate cancer incidence in the first and second generations of these men. Selenium and Vitamin E levels are inversely correlated to prostate cancer incidence [1, 4, 10, 16].
27.4 Diagnostic and Screening Tests
Langstaff reported the first case of prostate cancer in 1817. Since then the reported cases of incidence of prostate cancer have steadily increased due to the screening methods that have been employed to detect prostate cancer. Digital rectal examination (DRE) , Prostate-Specific Antigen (PSA) test , Transrectal UltraSound (TRUS) , Needle Biopsy are some of the tests used to diagnose prostate cancer. DRE detects palpable tumors which however can result in false positives if the growth is benign prostatic hyperplasia. The PSA test is the universal test used to detect prostate cancer or the initiation of the disease by measuring the serum concentrations of PSA. The normal concentration range for PSA is 0–4 ng/ml. TRUS is an imaging technique where a handheld transducer is used with a frequency between 6 and 7 MHz and rotated around in the prostate to give an idea of any potential outgrowths and their size range. Needle Biopsy is used to confirm a diagnosis of prostate cancer. The diagnosis obtained from either of the above three methods warrants confirmation by performing a biopsy. While each method identifies prostate cancer, the sensitivity is higher when two or more methods are combined to detect the cancer [4].
ABCD and TNM staging are the popular forms of staging of prostate cancer. In the ABCD system, early stage prostate cancers are referred to as stage A cancers and subsequent increases in tumor growth are labeled stages B, C, and D. The TNM classification identifies the cancer based on initial growth, presence of positive lymph nodes, and burden of metastasis. DRE, PSA, TRUS, and DNA ploidy are used to identify the stage of prostate cancer. Computed tomography (CT), magnetic resonance imaging (MRI), and radionuclide bone scans confirm the clinical staging results. Biopsies are histologically graded as per the Gleason grading system which is the most popular form of cancer grading. For most solid tumors, the histopathological indicators of biological aggressiveness have been determined through the comparison of tumor morphology with corresponding clinical outcome. Surgical staging is done by radical prostatectomy or lymphadenectomy [4].
The histological grade of the primary prostate cancer is evaluated using the Gleason grading system [17]. In this system, grading is based upon the degree of glandular differentiation and growth pattern of the tumor as it relates to the prostatic stroma. The pattern may vary from well differentiated (grade 1) to poorly differentiated (grade 5). This system takes into account tumor heterogeneity by summing the score of both the primary and secondary tumor growth patterns. For example, if the majority of the tumor is well differentiated (grade 1) and the secondary growth pattern is poorly differentiated (grade 5), the combined Gleason sum would be a 6. Low (i.e., score = 5) Gleason sum prostate cancers predictably have minimal aggressive behavior whereas very high (score = 8–10) Gleason sum tumors are usually highly aggressive [18]. Unfortunately, the intermediate (score = 5–7) Gleason sum tumors are highly unpredictable in their clinical aggressiveness [18]. This limitation is of particular importance as the majority of tumors (76 %) fall into this intermediate Gleason sum category [19]. Thus, predicting the biologic potential of the majority of prostate cancer in asymptomatic patients based upon histology alone is problematic.
27.5 Anatomy and Histology of the Prostate
To understand the molecular aspects of prostate cancer, it is essential to understand the anatomy and histology of the prostate gland. The prostate gland arises from different segments of the urethra and is located at the base of the bladder. It is a fusion of glandular and non-glandular regions [9, 20]. The prostate gland can be divided into three main glandular zones: (1) the peripheral zone, (2) the central zone, and (3) the transition zone, and in addition, a smaller periurethral gland region [9, 20, 21]. The basic function of the prostate is to secrete proteins to the seminal fluid [9]. The prostate develops from the urogenital sinus during embryogenesis and the differentiation process requires the interaction of the epithelial cells with the mesenchyme. This interaction is essential since absence of either component fails to generate the prostate and this has been elegantly shown through the recombination experiments of the laboratory of Cunha [22]. Androgen signaling also plays an important role in this interaction by acting on the mesenchymal component for the initial development of the prostate and subsequently on the epithelial component instructing the cells to secrete proteins.
The prostate epithelium consists of three types of cells: luminal, basal and neuroendocrine.
27.5.1 Luminal Cells
The luminal cells are secretory cells which secrete the seminal fluid. These cells also exhibit the androgen receptor and other proteins such as cell surface marker CD57 and prostate-specific antigen (PSA) [9]. The secretory cells of the peripheral, transition, and periurethral gland zones are more uniform in structure compared to the irregular luminal secretory cells [21].
27.5.2 Basal Cells
The basal cells lie between the basal lamina and the luminal cells. These cells do not have a secretory function, possess the androgen receptor in very low quantities, and cannot be detected immunohistochemically by staining for PSA. They express anti-apoptotic genes such as Bcl2, DNA damage protecting genes, for example, Gst-π, c-Met, p53-related protein p63, and prostate stem-cell antigen (PSCA) [20, 23]. The basal cells are made up of stem cells and the transit-amplifying cells which are intermediate cells of the prostate. These transit-amplifying cells are postulated to act as the precursors of prostatic inflammatory atrophic cells (PIA) which go on to become prostatic intraepithelial neoplasia followed by carcinoma [23].
27.5.3 Neuroendocrine Cells
In all the zones of the glandular prostate, a small population of endocrine–paracrine cells is located on the basal cell layer but do not grow into the lumen. These are called neuroendocrine cells and were first described by Pretl [24]. These cells have no specific function but may play a regulatory role in the development of the prostate as well as in the secretory aspect of the luminal cells. Somatostatin, bombesin, serotonin, and calcitonin are some of the peptides secreted by the neuropeptide cells and can be used as markers to identify these cells [24]. During androgen ablation therapy, prostate cancer cells regress and subsequently regrow in the absence of androgen. Neuroendocrine cells are observed during the stage when the prostate cells start to develop in the absence of androgen [24].
27.5.4 Glandular Tissues of the Prostate
The urethra is the base around which the zones of the prostate develop. The urethral wall forms the backdrop for the development of the ducts of the peripheral and central zones. The prostatic urethra is divided equally into the distal peripheral and proximal central halves divided at the point of the verumontanum by an angular (35°) wall between the apex and the bladder neck. The ejaculatory ducts lie along the proximal part of the urethra ending at its base [20].
27.5.5 Peripheral Zone
The peripheral zone forms approximately 70 % of the prostate. The ducts protrude from the lateral side and posteriorly from the urethral wall. Most carcinomas arise in the peripheral region.
27.5.6 Central Zone
The central zone covers 25 % of the prostate and the ducts surround the ejaculatory ducts which lie in the distal urethra. Carcinomas rarely arise in the central zone.
27.5.7 Transition Zone
Benign prostatic hyperplasia (BPH) arises in the transition zone which lies in the angular region of the urethra between the verumontanum and the bladder neck. This zone consists of 5 % of the prostate.
27.5.8 The Periurethral Gland Region
This region consists of small ducts and acini that lies within the proximal urethral segment and is the site where BPH arises [20, 21]. There are ducts and acini in all the glandular regions of the prostate which terminate in either the capsule or the anterior fibromuscular stroma. The ducts and acini stain for PSA and prostatic acid phosphatase (PAP) [20, 21].
27.5.9 Non-glandular Tissues of Prostate
Preprostatic sphincter, striated sphincter, anterior fibromuscular stroma, and prostatic capsule are the non-glandular tissues of the prostate. The preprostatic sphincter lies posterior to the urethra and its fibers spread and connect with the ducts of the transition zone. Anteriorly, the fibers end in the anterior fibromuscular stroma. The anterior fibromuscular stroma extends up to the prostate apex and into the capsule laterally. It is also in contact with the preprostatic sphincter and indirectly with the transition zone. The prostate is covered with striated sphincter muscles that end incompletely at both the distal and proximal ends. The prostatic capsule encloses a major part of the prostate and the terminal acini of the peripheral and central zones are in contact with the capsule. The capsule does not extend over the ejaculatory ducts or the bladder neck. Neurovascular bundles innervate the lateral portion of the capsule.
27.6 Prostate Cancer Progression Pathway
How is the morphology of the prostate and prostatic cells interconnected with the tumorigenic process? The three major processes affecting the prostate are prostatitis, BPH, and prostatic adenocarcinoma [25].
27.6.1 Prostatitis
Inflammation of the prostate, prostatitis, affects 2–10 % of men who are under the age of 50. The National Institutes of Health (NIH) classified prostatitis into four categories: (1) acute bacterial prostatitis , (2) chronic bacterial prostatitis , (3) non-bacterial prostatitis , and (4) prostatodynia . Acute and chronic bacterial prostatitis are rare occurrences affecting 2–5 % of men. They are caused by infection from bacteria such as Escherichia coli and Serratia marcescens. Local symptoms are urinary frequency and pelvic pain and systemic symptoms are fever and chills. Local and systemic symptoms are seen in acute prostatitis while intermittent local symptoms are observed in chronic prostatitis. Treatment normally involves anti-microbial agents and drainage of urine. The common form of prostatitis is category III which is chronic non-bacterial prostatitis. It affects 90–95 % of men. Infectious cause is not seen in non-bacterial prostatitis. The National Institutes of Health Chronic Prostatitis Symptom Index Questionnaire (NIH-CPSI) is used to assess the condition. There are two subcategories of non-bacterial prostatitis which are inflammatory (IIIA) and non-inflammatory (IIIB). Treatment involves the use of anti-inflammatory drugs, thermal therapies. However, specific direct treatment of the condition has not been resolved. Category IV or asymptomatic inflammatory prostatitis does not require any treatment. It is observed in some cases where the PSA levels are high but there is no correlation to prostate cancer [26–28].
27.6.2 Benign Prostatic Hyperplasia (BPH)
BPH and prostate cancer are observed with a high frequency in men of advanced ages. How does BPH differ from prostate cancer? BPH develops in the region of the prostate that is adjacent to the urethra. BPH nodules, unlike prostate cancer, arise in the transition epithelial zone of the prostate. BPH involves an increase in the size of the prostate from its normal size of 15–20 g to three times the size of approximately 33–52 g during autopsy and surgery, respectively [29]. BPH accounts for a large percentage of surgeries in the Western world due to improved screening procedures. The prevalence of BPH is low in men of approximately 30 years of age. However, the prevalence of BPH increases linearly with increasing age and is maximum in men who are in their seventh decade of their lives [29, 30]. There are two stages in BPH: microscopic and macroscopic. The microscopic stage is found to be present in almost all men of the world during their lifetime. This consists of the development of the BPH nodules. One-half of the microscopic nodules develop further resulting in a macroscopic enlargement of the prostate now termed as the macroscopic stage. The macroscopic stage consists of the pathological phase and the clinical phase [30]. Detection of the clinical stage of BPH or dysuria involves surgical intervention. A couple of factors resulting in the development of BPH are a functioning testis providing a steady supply of testosterone coupled with a steady increase in age of the individual. The influence of hormones is very obvious since BPH fails to develop in castrated men [29, 30]. However, the changes that occur within the cells of the prostate also contribute to the development of BPH. There are three theories that have been proposed which may explain the etiology of BPH. The first theory deals with the influence of hormones for e.g. DHT. The DHT hypothesis states that a functioning testis, supplying androgens, is involved in the development of the prostate. With aging the androgen balance shifts resulting in an increase in androgen levels which may explain the hyperplastic growth of the prostate leading to BPH. The second theory involves the stem-cell-epithelial cell interaction. This was proposed by Reischauer in 1925 and restated by McNeal where they observed that BPH results from an initial glandular budding and branching of the stromal cells of the prostate evolving into alveoli consisting of epithelial cells [30]. In subsequent analyses on animal models, this induction of epithelial cells from stromal cells could be demonstrated. Cunha et al. have shown the induction of bladder epithelial cells to form prostate cells by mouse embryonic urogenital sinus mesenchyme which essentially are prostate stromal cells [22]. The induction is sensitive to androgens as castrated animals fail to show the stromal-epithelial induction. The third hypothesis is the stem-cell hypothesis. Stem cells are regenerative cells that can grow in conditions where the cell number is very low due to extreme physiological conditions or environmental influences. The prostate is a self-renewing tissue comprised of equal numbers of proliferating cells and cells undergoing apoptosis. This equilibrium in the tissue allows the prostate to maintain its size in an individual. Originating from the stem cells, the amplifying cells exhibit limited proliferation and are active in the regeneration of tissue within the prostate. The limited proliferation results in an amplification of the number of cells added to the regenerating prostate. The transit cells are a subset of the amplifying cells and have very limited clonal expansion. Unlike the amplifying cells, are dependent on the supply of androgens. The possible explanation for the development of BPH can be attributed to the clonal expansion of these stem cells which results in the overgrowth of the prostate. However, with androgen ablation, the transit cells which, make up majority of the prostate, die to cause involution of the prostate. This stem cell theory explains the regeneration of the prostate when androgen therapy is given to castrated men resulting in the prostate regaining its maximal size. Other factors also play a role in the development of BPH. The changes observed in BPH cells compared to normal are androgen metabolism and receptor differences, variable degrees of cytosine residue methylation and variations in levels of secretory proteins [30]. The incidence of BPH is similar to prostate cancer exhibiting racial differences with blacks showing a large frequency of the disease and Asians show the least [31].
Latent prostate cancers are most often observed during screening tests. Some of the latent cancers develop into malignant metastatic cancers. The prostate cancer progression pathway has been shown to consist of four predominant molecular chromosomal aberrations. The normal epithelium of the prostate cell develops into prostatic intraepithelial neoplasia (PIN) when the basal cells are lost. A candidate gene that is a cause of this transformation is NKX3.1, which is a result of loss of the locus 8p21. LOH is observed in PTEN and Rb which lie on loci 10q and 13q resulting in loss of the basal lamina. Invasive carcinoma is generated with the breach of the basal lamina. Eventually with the loss of 17p containing the candidate gene p53, the cancer cells metastasize to distant sites such as bone [25, 30, 32].
Understanding the molecular aspects of cancer is vital to understand the underlying pathways actively playing a role in tumorigenesis. The tumorigenic process results in the transformation of the cells in the prostate resulting in the following stages. Inflammation due to damage to the tissue results in damage to the cells and subsequently the genome. It also triggers the production of cytokines and other DNA repair mechanisms. Oxidative radicals such as hydrogen peroxide, singlet oxygen, superoxide, and nitric oxide are produced to counter the infectious agents. Their action affects the infectious agents as well as the bystander host cells. The damage to the host cells can result in genomic damage and mutations which may initiate the tumorigenic process. GSTP1 hypermethylation or the reduced effect of the DNA glycosylase/AP lyase-OGG1 reduces the ability of normal cells to counter the damage of oxidants [25]. Besides inflammation, other factors such as unequal levels of androgens and dietary factors can initiate the tumorigenic process.
27.6.3 Prostatic Inflammatory Atrophy (PIA)
DeMarzo in 1999 proposed the development of PIN from prostatic inflammatory atrophy or PIA through the atrophy of the luminal secretory cells with surrounding inflammation and is observed adjacent to cells that have undergone PIN and carcinoma. Such cells are found in the peripheral zone of the prostate and very rarely in the central zone of the prostate [23, 33]. The epithelial cells undergoing atrophy are associated with acute or chronic inflammation. Such cells undergo morphological changes to resemble prostatic intraepithelial neoplasia (PIN) or may resemble minute carcinoma cells directly without a precursor lesion of PIN. Chromosome 8 is amplified in PIA cells and show other changes similar to PIN and cancer such as GSTP1 promoter methylation and p53 mutations. Certain cells that do not show inactivation of GSTP1 activity are able to counter the effects of oxidative damage [15, 33]. Cells undergoing PIA are proliferative, expressing AR and bcl-2 and rarely undergo apoptosis [23]. They also exhibit low levels of the cyclin-dependent inhibitor p27Kip1 which may be responsible for the initiation and proliferation of cells [33]. High levels of COX-2 and glutathione S-transferase A1 (GSTA1) are observed in cells undergoing PIA [30, 32, 33]. PIA may give rise to PIN in some cases while in other cases this is not observed. Hence, PIA can be considered as a precursor or intermediate lesion in the tumorigenic process of the prostate.
27.6.4 Prostatic Intraepithelial Neoplasia (PIN)
When the luminal cells undergo proliferation with dysplasia along the ducts, it results in prostatic intraepithelial neoplasia or PIN. PIN has two forms, low-grade (LGPIN) and high grade (HGPIN) . In 1986, McNeal and Bostwick proposed diagnostic criteria to detect PIN in the prostate which resulted in the three grade system [34]. However in 1989, the American Cancer Society and National Cancer Institute converted this into a two grade system where Grade 1 is LGPIN and Grades 2 and 3 together are HGPIN. In 1987, Bostwick and Brawer coined the term prostatic intraepithelial neoplasia. In general, HGPIN is considered to be a precursor of invasive carcinoma, but some studies have implicated the possible involvement of LGPIN as a precursor as well [16]. It is possible that the changes accumulating in LGPIN and HGPIN ultimately evolve into carcinoma despite the long latency period. LGPIN have enlarged nuclei, small nucleoli, normal chromatin content which may be slightly increased as well. The basal cell layer is intact in LGPIN. HGPIN on the other hand, have large uniform nuclei and nucleoli which are similar to carcinoma cells. The basal cell layer is disrupted in HGPIN and is absent in carcinoma [35]. A key marker protein to indicate the presence or absence of the basal cell layer is the anti-keratin stain 34βE12 through immunohistochemical analysis. Some of the marker proteins, amplified in the transition of HGPIN to carcinoma, are bcl-2, C-erbB-2, C-erbB-3 oncoproteins, racemase, c-Met protooncogene, telomerase, AMACR, and PSCA [35, 36]. Secretory marker proteins such as prostatic acid phosphatase (PAP) and PSA are downregulated with advanced PIN and carcinoma. Common allelic loss is observed in PIN and invasive carcinoma in loci such as 8p, 10q, and 16q. Epigenetic regulation of GSTP1 where the promoter is hypermethylated is observed in both PIN and carcinoma [37]. Digital rectal examination, TRUS, and PSA tests may be used to detect PIN. Repeat biopsies for cancer detection have reduced following a diagnosis of HGPIN. This is due to screening of younger males and as a result a reduction in tumor volume. Androgen deprivation therapy reduces the number of cases of PIN due to apoptosis in these cells [35].
27.6.5 Intraductal Prostatic Adenocarcinoma (IDC-P) and Invasive Carcinoma
Melicow and Pachter identified the differences between IDC-P and HGPIN. IDC-P is the next stage after HGPIN and coexists with adjacent invasive carcinoma [20, 38]. IDC-P is a late event in prostate cancer found adjacent to tumors with sizes greater than 4 cm3. IDC-P comprises 0.4–0.8 % of prostatic adenocarcinomas and is characterized histologically by Gleason grade 3 and 4 cribriform carcinoma [20, 38–40]. This histology of the prostate is seen in the ducts and acini where the lumen is filled with malignant cells [41]. Endometrioid carcinoma is another term given to IDC-P due to the observation of ductal outgrowths near the verumontanum of the prostate resulting in an enlarged palpable prostate. Distant metastases were observed in the bone, brain, lymph nodes, and lungs [39]. Histologically, the tumor cells show pseudostratification with elongated nuclei and ample amounts of amphophilic cytoplasm. Other histologies include papillary form cells, glandular gland like cells with slit-like lumen spaces and central necrosis (comedonecrosis). Invasive carcinoma is classified as Gleason grade 5 where the cells lost the basal cell layer as compared to HGPIN and IDC-P. Invasive lesions contain tiny blood vessels and can be detected by immunostaining for basal cell-specific keratin. While IDC-P is restricted to the ducts and acini of the prostate, invasive carcinoma can metastasize [42]. The tumors involving IDC-P originated from the periphery of the prostate or secondary prostatic ducts [40]. Ultrastructurally, IDC-P cells reveal the following features of gland like cells with distinct basal lamina, microvilli in the lumen, large nuclei, and nucleoli. In addition, prostatic origin is confirmed in IDC-P cells due to the presence of prostatic acid phosphatase (PAP) and prostate-specific antigen (PSA). There are a few variables that are strongly correlated with tumor volume. The volume of IDC-P tumor is strongly correlated with the extent of penetration of the capsule in terms of its thickness and width [42]. IDC-P tumors have much in common with invasive carcinomas in terms of genomic instability with higher frequencies of allelic imbalance (60 %) observed compared to HGPIN or BPH. Allelic imbalance in the form of loss of heterozygosity is seen in IDC-P and invasive carcinoma. LOH in certain loci are shared between IDC-P and invasive carcinoma while there are areas of LOH unique to each of them. If IDC-P evolves into invasive carcinoma, the unique areas of LOH in IDC-P can only be explained as the result of selective clonal growth in IDC-P which gives rise to invasive carcinoma. The LOH observed in IDC-P indicates its correlation with a poor prognosis of prostate cancer. Gene amplification of c-myc on 8q24 and gene fusion of TMPRSS2-ERG are seen commonly in IDC-P as well as invasive carcinoma [38, 41]. McNeal et al. [42] observed that rather than being a precursor to invasive carcinoma, IDC-P was found as large foci within invasive carcinoma which was larger than 2–4 cm3. When IDC-P arises within invasive carcinoma, it generates a malignant potential within the invasive tumor. IDC-P that develops as foci within invasive carcinoma can be distinguished based on its cribriform cells which still retain the basal cell layer [42]. IDC-P possesses two types of cells, tall pleomorphic, mitotically active cells staining poorly for prostate-specific antigen (PSA) and cuboidal, cells containing a secretory layer along with abundant cytoplasm expressing large amounts of PSA [41]. The treatment regimen for IDC-P is similar to that followed for hormone dependent prostate cancer. Transurethral resection, hormone ablation using diethylstilbestrol or orchiectomy, and radiation therapy are some of the techniques utilized [43].
27.6.6 Androgen-Independent Prostate Cancer
It is well known that hormone ablation treatment of prostate cancer initially causes the cancer to regress. Most patients are disease free for approximately 1.5–3 years before the cancer reappears with intense ferocity and gains the capability to proliferate independent of the supply of androgens. Androgens maintain the ratio of cells within the prostate where there is a balance between dividing proliferating cells and the cells undergoing death. On hormone deprivation, the cells undergoing apoptosis increases and the equal ratio of cells is affected leading to cancer cell death and the regression of prostate cancer. Charles Huggins and Clarence V. Hodges showed the effect of castration on acid phosphatase and alkaline phosphatase. Acid phosphatase levels decreased sharply on castration while alkaline phosphatase levels decreased gradually. Alkaline phosphatase is an enzyme found in bone and cartilage and is indicative of activity of cells outside the prostate gland. Acid phosphatase is found in the cells of the prostate and is an effective indicator of the growth of prostate cells [44].
Androgens comprise testosterone, dihydroepiandrosterone (DHEA) , androstenediol, and androstenedione. Testosterone makes up 90 % of the androgens and freely diffuses into prostate cells. Testosterone which is synthesized in the testes is found in blood in the bound form where albumin or sex-hormone-binding-globulin binds the hormone. On entering prostate cells, the enzyme 5α-reductase (SRD5A2) converts testosterone to the active hormone, dihydrotestosterone (DHT), which binds to the androgen receptor (AR) . This causes a conformational change in the receptor which then dissociates from the heat shock proteins and is translocated into the nucleus where it binds to androgen response elements in the promoter regions of genes to activate their transcription [45–47]. So what causes the cells in the prostate to get recharged after castration and to proliferate resulting in a more aggressive androgen-independent cancer? There have been many theories proposed on the causes of recurrence of prostate cancer. Five main theories are: (1) Hypersensitive pathway, (2) Outlaw pathway, (3) Promiscuous pathway, (4) Coactivators and Corepressors, and (5) Bypass pathway [45–47]. These theories deal with the androgen receptor and the role it plays in the development of the hormone refractory or androgen-independent cancer. In the hypersensitive pathway, the androgen receptor becomes extremely sensitive to very low amounts of androgens that may be present even after castration or medical blockade of androgens. The androgen receptor through mutations or overamplification or increased 5α-reductase enzyme levels may become sensitive to low levels of androgens. 30 % of androgen-independent cancers show amplification of androgen receptor in cells which may be a result of selective outgrowth following death of cells during castration [46, 48]. Increased 5α-reductase enzyme levels result from a polymorphism where there is a substitution of valine with leucine at codon 89. This is commonly observed in African men indicating the ethnic influence in prostate cancer [46, 47]. Androgen-independent prostate cancer, though independent of the supply of androgens tend to express the androgen receptor at high levels in most cases. The outlaw pathway is functional when the AR pathway is activated by growth factors and receptor tyrosine kinases. In these cases, growth factor pathways such as insulin growth factor (IGF), keratinocyte growth factor (KGF) can bind and activate the androgen receptor in the castrated state as they are overexpressed. Receptor tyrosine kinases such as HER-2/neu are overexpressed in androgen-independent cancers that results in the activation of the androgen receptor. IL-4 and IL-6 are also activators of the androgen receptor pathway in the hormone refractory state [45, 46]. The cross talk between NF-kappa B and AR have indicated the role of the NF-kappa B signaling pathway in the development of androgen-independent cancer. The promiscuous pathway results in the androgen receptor (AR) being receptive to ligands other than DHT. Non-androgenic steroids, anti-androgens can activate the AR due to missense and other mutations which may expand the ligand-binding specificity of the AR. LNCaP cells possess an AR where threonine is substituted with alanine at codon 877 which is sensitive to a wide range of steroid ligands. CWR22 cells also show a substitution of histidine with tyrosine at codon 874 in the sequence that encodes AR. Mutations in the AR are observed in TRAMP mice in three regions: (1) the signature loop of the receptor, (2) the flanking region where p160 coactivators bind, and (3) the ligand-binding domain [46]. Coactivator levels are increased in androgen-independent cancers which enhances the sensitivity of the androgen receptor to various ligands other than androgens. Coactivators such as ARA70, ARA55, SRC-1, P/CAF, and GRIP1/TIF2 are overexpressed in prostate cancer [45, 46, 49]. Bypass pathway, as the name suggests, circumvents the AR pathway and utilizes other pathways in stimulating the prostate cells to proliferate in an androgen-independent environment. The activation of bcl2 in PIN lesions can cause the cells to avoid utilizing the androgen receptor pathway. Similarly, the activation of oncogenes or inactivation of tumor suppressor genes may result in other bypass pathways. Neuroendocrine cells secrete neuropeptides such as bombesin which enhance the proliferative rate of cells in a cancerous environment in the absence of hormones [45, 46, 49]. Shi et al. [50] have examined the alterations involved in the transition of LNCaP cells into androgen-independent cells and have observed upregulation of growth factors, the Bcl-2 protein and the Akt pathway. The three sublines generated were representative of the theories proposed of development of androgen-independence [50]. Chen et al. [51] also performed similar analyses using LNCaP and the androgen-independent C4-2 cells and analyzed genes such as TMEFF2, NKX3.1, and AMACR to compare the gene expression differences. In addition, these genes were further analyzed in xenografts of these cells to observe consistency in expression. The study analyzed 51 candidate genes and compared the results to human prostate cancer tissues and showed a correlation. The study by Shi et al., as well as Chen et al., showed the difference in gene expression in androgen-dependent and androgen-independent prostate cancer [51].
Besides the androgen receptor, the microenvironment plays a role in the development of androgen-independent cells. Studies by Cunha et al. have shown the effect of the stroma on the epithelial cells of the prostate. Recombination of mice epithelial cells with inactive AR with stromal cells of mice containing functional AR showed that the mice epithelial cells developed normal prostatic cells yet the reverse combination failed to yield functional epithelial cells despite the addition of androgens. This indicated the importance of the stroma in prostate cell development [48]. The stromal cells can activate the epithelial cells through paracrine signals while the growth of prostate cells in an androgen-independent environment can also occur in an autocrine manner. This was observed in experiments where prostate cancer cells could proliferate in nude mice that possessed either wild type AR or where the AR was inactive. The proliferation of prostate cells in an AR-null environment indicated autocrine signaling of cells to activate pathways besides the AR signaling pathway to survive in a hormone refractory environment [48]. Akakura et al. [52] have demonstrated the effect of intermittent androgen therapy on androgen-dependent tumors where it was observed that androgen-dependent tumors transplanted in castrated mice showed a regression. When the tumors had regressed 30 %, they were transferred to intact mice. The tumors started to develop only to be transplanted back into castrated mice. This intermittent procedure was conducted to observe the rate at which the tumors became androgen independent. The time taken for the tumors to develop into androgen-independent tumors was threefold. Such intermittent therapy could delay the development of the aggressive tumor that is hormone refractory [52]. Research has been carried out on androgen-dependent prostate cancer cells to observe their growth into androgen-independent cells and to analyze the changes that occur with this transition. Hendriksen et al. [53] have analyzed the androgen receptor pathway in LNCaP cells as well as xenografts from intact and castrated mice to observe the genes that are upregulated or downregulated in the transition to androgen independence. It was observed that androgen receptor genes that were not stroma-associated were upregulated in primary prostate cancer with low levels of stromal cells. In low grade carcinoma, genes that were involved in cell growth, proliferation, and apoptosis were expressed to higher levels compared to high-grade carcinoma where differentiation is lowered. The genes that are upregulated in low and high-grade carcinomas were involved in metabolism, exocytosis, and protein folding. SIM2 and AMACR were upregulated in prostate carcinoma. The study showed that stress response genes, HERPUD1 and STK39 were downregulated and were good indicators of metastasis [53].
27.7 Epigenetic Regulation in Prostate Cancer
The term epigenetics refers to the external modification of DNA bases which are inherited but do not cause a change in the DNA sequence. Epigenetic modifications vary from methylation of DNA to acetylation, phosphorylation, and methylation of histones [54]. DNA methylation occurs when a methyl group is added to the 5′ carbon of cytosine. Such modifications are generally observed in CpG islands that are 0.5–2 kb long and found in the promoter regions of genes. CpGs comprise 1 % of the genome. DNA methyltransferases or DNMTs are enzymes that catalyze the methylation of cytosines where S-adenosylmethionine (SAM) is the methyl donor for the reaction [54, 55]. In the cancer context, DNA methylation can be of two types, hypermethylation and hypomethylation. Hypermethylation results in the silencing of genes by methylation of the promoters of genes. Thus, loss of heterozygosity (LOH) observed in many cancers may be the result of methylation of an allele resulting in the loss. Hypomethylation on the other hand results when there is a loss of methylation leading to activation of genes. Genes such as GSTP1 and 14-3-3 are methylated and silenced in prostate cancer [55]. Yegnasubramanian et al. [56] analyzed the hypermethylation patterns in 73 primary and 91 metastatic human prostate cancer and compared the changes in methylation observed in the progression of cancer. They identified a unique methylation pattern involving the genes GSTP1, APC, PTGS2, MDR1, and RASSF1a. Hypermethylation of the CpG islands at the promoter region of the above genes was found at a high frequency in prostate cancer and was a distinguishing characteristic from other cancers. Recurrence in prostate cancer was correlated independently with high Gleason grade, pathological stage as well as methylation of the PTGS2 gene which encodes a cyclooxygenase gene that converts arachidonic acid to prostaglandins which are involved in inflammation. The methylation pattern did not differ between primary and metastatic carcinomas which indicated that methylation arose early in the progression of prostate cancer. There was a striking difference in the number and frequency of genes that were methylated between BPH and primary prostate cancers [56]. Lodygin et al. [57] in a similar study identified genes that were silenced in prostate cancer and analyzed 50 genes that were induced by treating prostate cancer cell lines (LNCaP, PC3, DU145) with the demethylating and inhibition of deacetylase agents 5-aza-2′deoxycytidine and trichostatin A (TSA). These genes were selected based on their functions and can be considered as potential candidates for therapy in prostate cancer. SFRP1 and DKK3 were genes that downregulated the Wnt pathway, p57/KIP2 regulates the cell cycle negatively, Glutathione peroxidase 3 (GPX3) protects cells against oxidative stress. These genes were shown to be methylated in prostate cancer which explains the release of the prostate cells from regulating factors and the development of dedifferentiation [57]. Histone modifications such as acetylation, methylation, ubiquitinylation regulate the transcriptional activities of genes. Histone acetyltransferases (HAT) catalyze the transfer of acetyl groups to histones. The androgen receptor is regulated by p300, PCAF that are histone acetyltransferases and upregulate AR gene expression. Similarly, histone deacetylation of the vitamin D receptor is observed in prostate cancer cells which inhibit the anti-proliferative activity of the receptor. Deacetylation is promoted by the histone deacetylase enzyme (HDAC) . Histones are additionally modified by methylation of lysine and arginine residues. Global hypomethylation which involves a reduction in the overall CpG methylation content of the genome is observed in benign as well as malignant prostate cancer. Gene-specific hypomethylation in prostate cancer is observed in genes such as PLAU which encodes a tumor invasive gene urokinase plasminogen activator and heparanase [54, 55]. Epigenetic mechanisms contribute to the process of tumorigenesis in prostate cancer along with mutations and are also involved in the progression to androgen-independence. As in the study by Murillo et al. [58], it was observed that methylation of specific genes can be observed in androgen refractory prostate cancer cell lines. LNCaP and its derivative androgen-independent cell lines, Rf and RfL were analyzed for genetic changes as well as epigenetic alterations. The locus 6q24-q26 was studied since it is known to have high allelic loss in cancer. The gene PLAG1 was identified in this locus and was seen to be downregulated in the androgen refractory cell lines. On further analysis, this downregulation and loss of one allele of the 6q locus was attributed to methylation [58]. Treatment of epigenetic modifications is universal irrespective of the cancer. Methyltransferase inhibitors such as 5-aza-2′deoxycytidine and decitabine (DAC) restore expression of genes such as GSTP1 and histone deacetylase inhibitors such as MS-275, SAHA can be used to reverse the deacetylation of genes resulting in their activation. These treatments are effective for short periods of time and do not completely shrink the tumors. Combination therapies involving demethylase and HDAC inhibitors are used [54, 55].
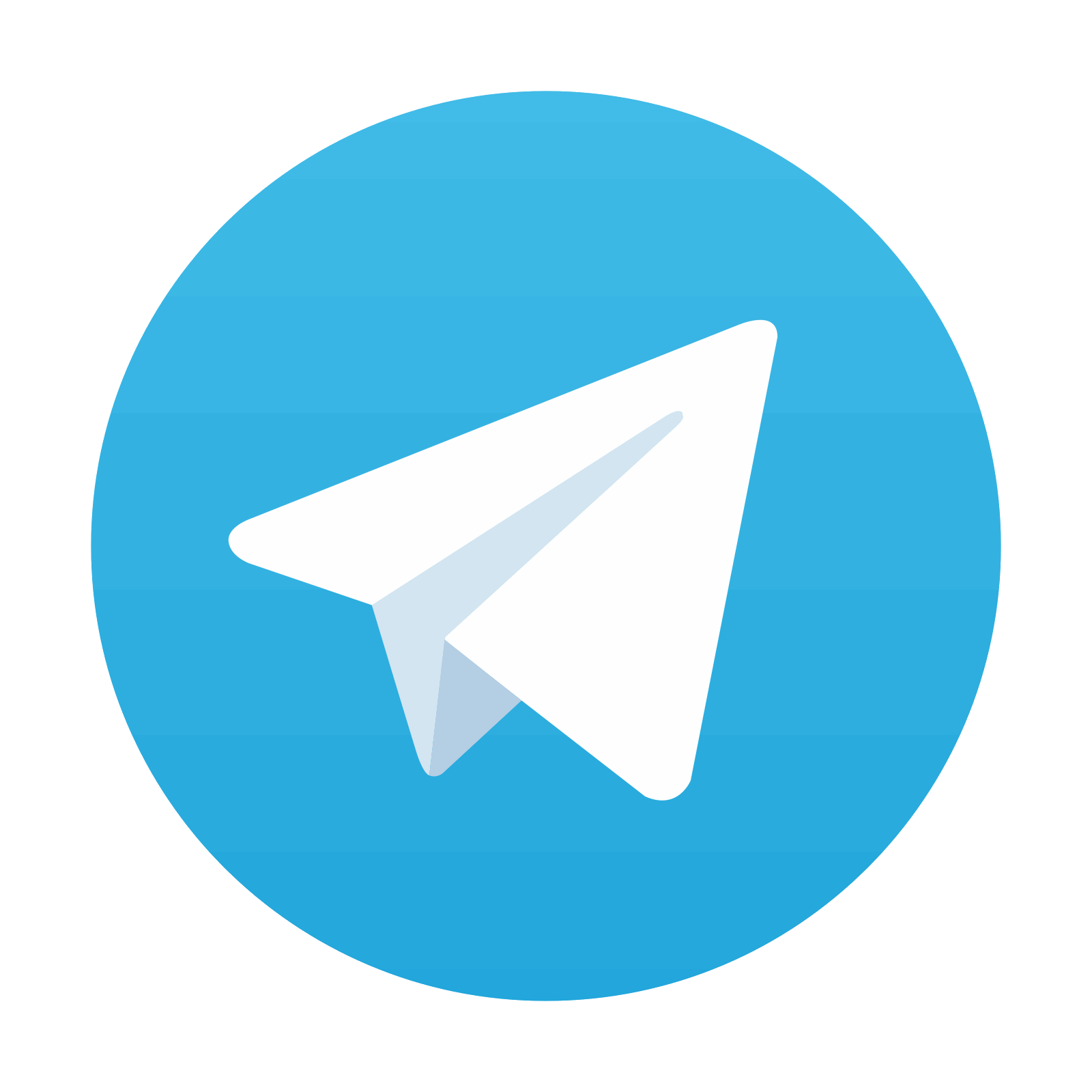
Stay updated, free articles. Join our Telegram channel

Full access? Get Clinical Tree
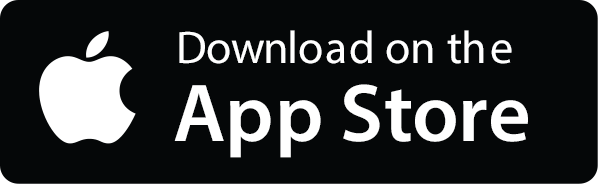
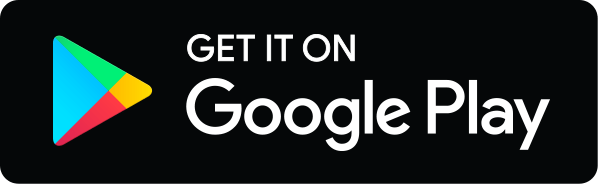