The Male and Female Reproductive System in Thyrotoxicosis
Gerasimos E. Krassas
Nikolaos E. Pontikides
The reproductive system has been suggested to be relatively resistant to the effects of thyroid dysfunction. This view has been challenged by recent evidence. For example, the classic assumption that the adult male gonad is unresponsive to thyroid hormone is no longer tenable; the seminiferous epithelium of the prepubertal testis can now be considered a novel thyroid hormone–responsive tissue (1).
Historically, Kendle reported for the first time in 1905 the development of precocious puberty in a young girl with severe hypothyroidism (2). It has since been largely confirmed that significant associations do exist between thyroid disorders and abnormalities of the reproductive system: Both thyrotoxicosis and hypothyroidism in males and females have been well documented to produce variable degrees of gonadal dysfunction (3,4,5,6,7,8).
The aim of the present communication is to discuss relevant information regarding the effects of thyrotoxicosis on reproduction in males and females.
Reproductive Effects of Thyrotoxicosis in Male
For a better understanding of the effect of thyrotoxicosis on the male reproductive system, a brief review of the normal development and physiology of the male reproductive system follows.
The Male Reproductive System from Fetal to Adult Life
Male gonadal differentiation begins at 7 weeks of gestation, with organization of the gonadal blastema into interstitium and germ cell–containing testicular cords. Primitive Sertoli’s cells and spermatogonia become visible within the cords, while the epithelium differentiates to form the tunica albuginea (9). Leydig’s cells derived from the undifferentiated interstitium are visible by the end of the eighth week of gestation and are capable of androgen synthesis at this time. By 14 weeks of gestation these cells make up as much as 50% of the cell mass, but as the tubules develop they account for a smaller percentage of the tissue. The fetal testes grow from approximately 20 mg at 14 weeks of gestation to 800 mg at birth; at 5 to 6 months they descend into the inguinal canal in association with the epididymis and the ductus deferens (9). Testicular secretion of testosterone (T) in the fetus reaches a peak late in the first trimester and then declines until parturition (10). The fetal testis also produces anti-müllerian hormone,
which causes dedifferentiation of the müllerian duct system in the male fetus (11). In the first year of life, there is a transient increase in T, after which the testis remains relatively quiescent until the onset of puberty. The pulsatile secretion of gonadotropin-releasing hormone (GnRH) causes pulsatile secretion of gonadotropins (Gns). Plasma levels of luteinizing hormone (LH) and follicle-stimulating hormone (FSH) in the fetus increase after the establishment of the hypothalamic–pituitary portal system until midgestation and then decrease toward term as inhibitory control begins to function; mean levels of fetal plasma FSH are higher in females than in males (12). During the first 2 years after birth, plasma levels of LH and FSH increase intermittently to adult values and occasionally higher, and then they remain low until puberty.
which causes dedifferentiation of the müllerian duct system in the male fetus (11). In the first year of life, there is a transient increase in T, after which the testis remains relatively quiescent until the onset of puberty. The pulsatile secretion of gonadotropin-releasing hormone (GnRH) causes pulsatile secretion of gonadotropins (Gns). Plasma levels of luteinizing hormone (LH) and follicle-stimulating hormone (FSH) in the fetus increase after the establishment of the hypothalamic–pituitary portal system until midgestation and then decrease toward term as inhibitory control begins to function; mean levels of fetal plasma FSH are higher in females than in males (12). During the first 2 years after birth, plasma levels of LH and FSH increase intermittently to adult values and occasionally higher, and then they remain low until puberty.
In the adult male, GnRH and Gns are secreted in discrete pulses. The secretory pulses of LH occur at a frequency of 8 to 14 pulses per 24 hours and vary in magnitude (13). Pulsatile secretion of FSH is temporally coupled to that of LH but is lower in amplitude (14). The secretion of LH is controlled by the negative feedback action of gonadal steroids on the hypothalamus and the pituitary. Both T and estradiol (E2) can effect this inhibition. T can be converted to E2 in the brain and the pituitary, but on the basis of the results of many studies the two hormones are thought to act independently (15,16). LH stimulates Leydig’s cells to secrete T and, to a minor extend, E2 (17). Also, LH appears to govern testicular aromatization in Leydig’s cells. In the normal adult male, T, dihydrotestosterone (DHT), and to some extend E2 circulate in the plasma bound in part to sex hormone–binding globulin (SHBG), which is synthesized in the liver (18). Thyroxine (T4) increases serum SHBG levels (18,19). Sex hormones bound to SHBG are inactive and appear not to be readily metabolized (18). The unbound sex hormones or those bound to albumin are biologically active. The T concentration in the testes is maintained at a high concentration relative to serum by androgen-binding protein secreted by Sertoli’s cells under the influence of FSH. The high intratesticular concentration of T may be necessary for normal Sertoli’s cell function or may play a role in spermatogenesis or sperm transport (20). In certain tissues, especially the prostate, circulating T enters the cell and is metabolized to more active products, especially DHT, through the action of the enzyme 5α-reductase II. In other tissues, 5α-reductase I is the major isoenzyme (21). Although DHT is the active androgen in some areas, T itself is an active androgen in some tissues that lack the enzyme, such as muscle. There is a decline in testicular function due to age (22), although the interplay of lifestyle and disease processes probably plays a role (23).
Although the testicular secretion of E2 is important, the major source of circulating hormone derives from aromatization of T in peripheral tissues, including adipose tissue, muscle, and skin; however, little aromatization appears to occur in the liver (24). These estrogens are further metabolized, primarily in the liver, to estriol and the catechol estrogens (25). The hypothalamus and pituitary can aromatize androgens, so it is uncertain whether T itself or the estrogens formed locally from T are the main negative feedback mechanisms for LH release (26). However, there is good evidence that one of the major factors controlling LH release is T itself. Inhibin is a major factor in the control of FSH secretion (27).
Therefore, masculinization in men depends on the actions of both T and DHT. The exact role of E2 is uncertain, but it appears in some processes, like bone formation, to play an important role.
Animal Studies on the Effects of Thyroid Hormone on the Reproductive System
The effects of thyroid hormone alterations on the reproductive system have been studied extensively in animals and have generally shown that changes from normal thyroid function resulted in decreased sexual activity and fertility (28,29). The underlying mechanisms, however, are not constant throughout all species, and results from different studies disagree.
In intact rats, the administration of T4 resulted in decreased serum gonadotropin levels (30). In immature male mice aged less than 4 weeks, the administration of slightly supraphysiologic T4 doses resulted in a tendency toward early maturation and shortening of the mice development period. Conversely, larger thyroid hormone doses resulted in decreased testes weights and seminal vesicles, both in mice and rabbits (28). Direct effects of T4 resulted in minimal oxygen consumption changes in the testes when T4 was present in testicular slice incubations (31). Administration of excess T4 to mature male rats also resulted in a decrease in total lipids, cholesterol, and phospholipids in the testes and rats rendered thyrotoxic by excess T4 administration were shown to synthesize increased amounts of T (30,32). Finally, the effects of T4 on spermatogenesis are conflicting, but it would appear that T4 does not exert a direct effect on spermatogenesis in mature rats or rams (33).
In rat, triiodothyronine (T3) affects testis maturation, and thyroid receptors (TR)α and TRβ are known to be expressed in rats’ testes (34). TRα1 is the specific TR isoform that has been proposed to be involved in testis function and development. Maximal Sertoli cell proliferation coincides with maximal T3 binding capacity in the testis, suggesting that the main target of T3 action is the Sertoli cell. However, T3 also plays a significant role in differentiation of the seminiferous epithelium, and studies in rodents have shown that T3 is an important factor in the maturation of Leydig cells. The presence of T3 is necessary to initiate differentiation of mesenchymal cells into Leydig progenitor cells and T3 works in concert with other hormones [LH, insulin growth factor (IGF)-1] to promote Leydig cell development (1,35).
Data from other animal species (such as deer, sheep, cattle, birds, mink) also suggest that T3 is a component of the neuroendocrine system that regulates seasonal cycles of reproductive activity (1). Although the underlying mechanisms remain unknown, it has been postulated that T3 triggers cessation of reproduction at the end of the reproduction season, since circulating T3 levels in deer rise at the time of seasonal transition to the non-breeding state and thyroidectomy results in absence of seasonal regression of the testis. T3 may also interact with – and modulate – the action of other hormonal systems such as growth hormone and steroids.
Table 27.1 Summary of Hormonal Changes in Male and Female Thyrotoxicosis | ||||||||||||||||||||||||||||||||||||||||||||||||||
---|---|---|---|---|---|---|---|---|---|---|---|---|---|---|---|---|---|---|---|---|---|---|---|---|---|---|---|---|---|---|---|---|---|---|---|---|---|---|---|---|---|---|---|---|---|---|---|---|---|---|
|
Human Studies
Hormonal Changes in Male Thyrotoxicosis
An increase in SHBG has been a feature consistently associated with thyrotoxicosis, leading to increased circulating levels
of total T and reduction in the metabolic clearance rate of T (36,37). By contrast, free T concentrations usually remained normal, although bioavailable T was found subnormal in hyperthyroid males (5). Total and free E2 concentrations were often elevated and, consequently, the free T/free E2 ratio was lower in hyperthyroid males compared with normal individuals (3,5,38,39,40). Relative free E2 elevation may contribute to the higher incidence of gynecomastia observed in hyperthyroid males with decreased libido (41,42). Another consistent finding has been that LH and FSH responses to GnRH administration were exaggerated in hyperthyroid males, contrasting with a blunted response of Leydig cells to human chorionic gonadotropin (hCG) administration, as assessed by serum T responses (38,39,40) (Table 27.1). Such abnormalities of the hypothalamic–pituitary–gonadal axis were significantly correlated with increased serum T4 levels (Fig. 27.1) and shown to be entirely reversible with restoration of the euthyroid status, thus indicating that no specific treatment of these thyroid hormone–induced abnormalities is required.
of total T and reduction in the metabolic clearance rate of T (36,37). By contrast, free T concentrations usually remained normal, although bioavailable T was found subnormal in hyperthyroid males (5). Total and free E2 concentrations were often elevated and, consequently, the free T/free E2 ratio was lower in hyperthyroid males compared with normal individuals (3,5,38,39,40). Relative free E2 elevation may contribute to the higher incidence of gynecomastia observed in hyperthyroid males with decreased libido (41,42). Another consistent finding has been that LH and FSH responses to GnRH administration were exaggerated in hyperthyroid males, contrasting with a blunted response of Leydig cells to human chorionic gonadotropin (hCG) administration, as assessed by serum T responses (38,39,40) (Table 27.1). Such abnormalities of the hypothalamic–pituitary–gonadal axis were significantly correlated with increased serum T4 levels (Fig. 27.1) and shown to be entirely reversible with restoration of the euthyroid status, thus indicating that no specific treatment of these thyroid hormone–induced abnormalities is required.
Table 27.2 Semen Characteristics in Thyrotoxic Men | ||||||||||||||||||
---|---|---|---|---|---|---|---|---|---|---|---|---|---|---|---|---|---|---|
|
Spermatogenesis, Fertility, Sexual Dysfunction and Thyrotoxicosis
Effects of thyrotoxicosis on semen quality have been the subject of only a few studies. Clyde et al. (43) investigated three young thyrotoxic males and found that two patients presented marked oligospermia with decreased motility, whereas the third patient had a borderline low sperm count with decreased motility. Kidd et al. (44) investigated five hyperthyroid patients and found that all had low total sperm counts. In 1992, Hudson and Edwards (45) had already assessed testicular function in 16 hyperthyroid males, and reported that the mean sperm density was slightly but not significantly lower than controls. In addition, forward progressive sperm motility in these patients was significantly reduced compared with normal males. Abalovich et al. (5) investigated the effect of hyperthyroidism on spermatogenesis in 21 hyperthyroid patients: 9 patients (43%) had low total sperm counts, 18 (86%) presented lineal motility defects, and 13 (62%) displayed progressive motility abnormalities.
In another study (41), 23 thyrotoxic males and 15 healthy controls were investigated prospectively by semen examination both before and 5 months after the restoration of euthyroidism by methimazole (MMI) treatment only (in 14 patients) or MMI plus radioiodine (131I) (in nine patients). Total fructose, zinc (Zn), and magnesium (Mg) concentrations were also measured in the seminal plasma in 16 of 23 patients. The results indicated that mean semen volume was within the normal range in thyrotoxic patients, but mean sperm density was lower, but not statistically significant when compared to controls. A similar trend was found for the analysis of sperm morphology; mean sperm motility was lower in thyrotoxic males when compared with controls. After treatment of thyrotoxicosis, both sperm density and motility improved, although sperm morphology did not change. The type of treatment employed (MMI alone or MMI plus 131I) had no impact on sperm count or morphology. Mean values for seminal plasma fructose, Zn, and Mg concentrations did not differ between controls and patients, before and after restoration of euthyroidism, and the values did not correlate
with sperm parameters or with thyroid hormone levels in the thyrotoxic period (Table 27.2).
with sperm parameters or with thyroid hormone levels in the thyrotoxic period (Table 27.2).
Another interesting aspect concerns the effects of thyrotoxicosis on sexual behavior. Despite normal basal T concentrations in hyperthyroid men, anecdotal reports claimed that erectile dysfunction (ED) was observed frequently, with incidence rates reaching 70% (46). Carani et al. (47) investigated 34 adult men with hyperthyroidism in a prospective, multicenter, uncontrolled study. The patients were screened for hypoactive sexual desire (HSD), ED, premature ejaculation (PE), and delayed ejaculation (DE) at presentation and again 8 to 16 weeks after restoration of the euthyroid state. Figure 27.2 shows that hyperthyroidism was associated with a marked increase in the prevalence of HSD, DE, PE, and ED. After restoration of euthyroidism, HSD and DE resolved in most cases. The most striking effect was the marked decrease in PE from 50% to 15%, that is, a figure similar to that found in the general population.
Using the Sexual Health Inventory for Males (SHIM), the impact of thyrotoxicosis on male sexual health in 27 hyperthyroid male patients (and 71 controls) who participated in a prospective, controlled study was evaluated (48). The patients were asked to respond to the SHIM five-item questionnaire, before and 1 year after initiation of treatment. A global score between 25 and 22 was considered normal, between 21 and 11 was indicative of mild to moderately severe ED, and 10 or less was diagnostic of severe ED. The results showed that 70% of hyperthyroid patients had a SHIM score of 21 or less, compared with only 34% in control individuals completing the questionnaire (p <0.0001). There was a positive correlation between serum-free T4 (FT4) values and SHIM scores (p = 0.005). Significant increases in SHIM scores occurred following the restoration of euthyroidism. The conclusion was that ED was extremely common in hyperthyroid males and that treatment restored normal erectile function. The authors also recommended thyroid function screening in all men complaining of ED and that, for hyperthyroid males, specific ED treatment could be postponed for at least 6 months after restoring euthyroidism.
Radioiodine Treatment for Hyperthyroidism and Reproduction
Radioiodine (131I) is widely used in the treatment of hyperthyroidism (49) (see Chapters 32 and 55). Because of potential mutagenic effects of radiation on the gonads, there is a legitimate concern regarding the possible side effects of 131I administration on reproductive function in young men. Reassuringly, several studies have reported normal reproductive performance in men with thyrotoxicosis after 131I therapy. 131I therapy is therefore justifiably used by clinicians in the treatment for thyrotoxicosis in adults of all ages (50,51,52,53).
Ceccarelli et al. (54) have evaluated a series of 15 thyrotoxic male patients before and at different times after 131I therapy. Mean basal FSH concentration was within the normal limits and did not change after therapy, although two patients showed substantial FSH increases lasting for 1 year (although one was already mildly hypogonadal before treatment). Asthenospermia was observed before 131I treatment in 10 of the 15 patients and sperm quality was significantly improved in 5 of these 10 patients within 1 year after therapy. Sperm morphology did not show any significant modification. LH was normal and did not change after 131I therapy, whereas T levels were reduced 45 days after 131I therapy and returned to basal values 1 year later. Thus, the radiation dose to the testes may account for small and transient damage, both to the germinal epithelium and Leydig cells. However, this damage is difficult to distinguish from that caused by hyperthyroidism per se.
For children aged 10 years or older, 131I administration may also be considered as a first line therapeutic option. The goal of radioactive iodine therapy in children is to achieve hypothyroidism by ablating the thyroid gland, and there is no apparent risk for future fertility (55,56,57).
After the administration of 131I for thyroid cancer, deleterious effects of gonadal irradiation are directly related to the
cumulative dose absorbed by the gonads, which is difficult to estimate after multiple 131I doses (58,59,60). Dose fractionation appears to increase testicular toxicity of irradiation (61). There are only a few reports on spermatogenesis and fertility status in male patients treated with 131I for thyroid cancer (62,63,64,65,66,67,68). Most of those studies have shown that 131I treatment for differentiated thyroid cancer (DTC) may cause transient impairment of testicular function. Specifically, transient suppression of spermatogenesis or, even complete azoospermia, has been noted, lasting up to 3 years in patients who received the higher doses. Sperm cryopreservation before 131I should be addressed in all young men, especially those likely to receive high and/or cumulative 131I doses (67,68).
cumulative dose absorbed by the gonads, which is difficult to estimate after multiple 131I doses (58,59,60). Dose fractionation appears to increase testicular toxicity of irradiation (61). There are only a few reports on spermatogenesis and fertility status in male patients treated with 131I for thyroid cancer (62,63,64,65,66,67,68). Most of those studies have shown that 131I treatment for differentiated thyroid cancer (DTC) may cause transient impairment of testicular function. Specifically, transient suppression of spermatogenesis or, even complete azoospermia, has been noted, lasting up to 3 years in patients who received the higher doses. Sperm cryopreservation before 131I should be addressed in all young men, especially those likely to receive high and/or cumulative 131I doses (67,68).
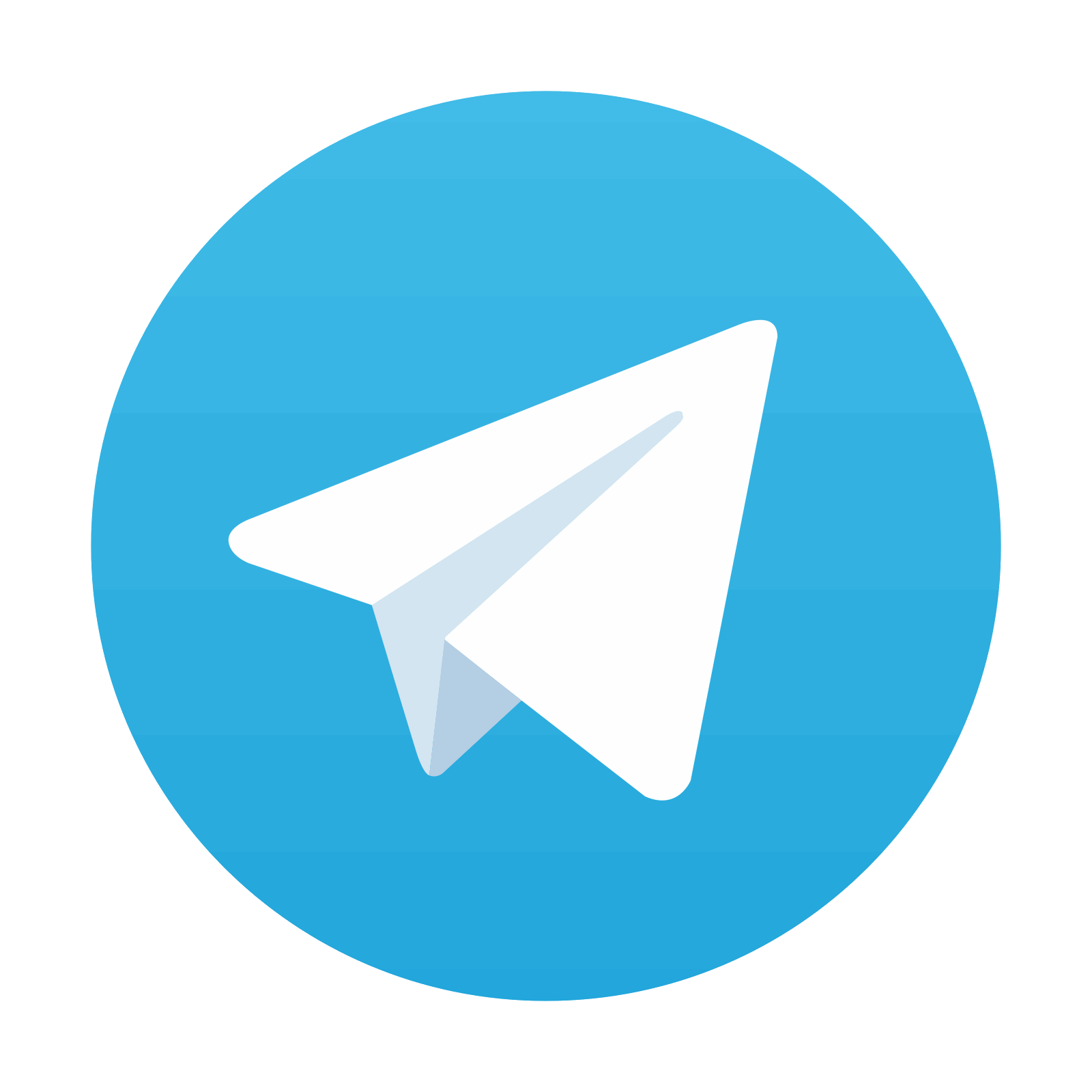
Stay updated, free articles. Join our Telegram channel

Full access? Get Clinical Tree
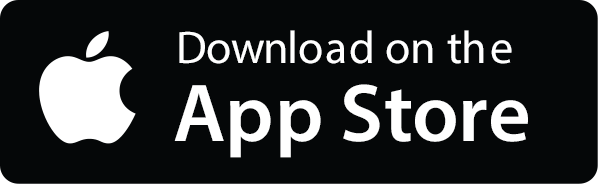
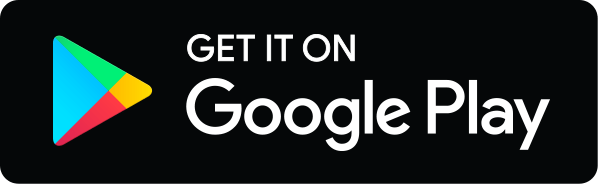
