1.1 Introduction
All living things – animals, plants and even bacteria – can act as hosts for infectious organisms and thus have evolved mechanisms to defend themselves against infection. Infection can be by other living things, non-living things (viruses) and possibly even molecules (prions). Since it is so crucial to our own survival, much of our understanding of immunity has come from studies in humans – particularly in relation to the causes and prevention of disease – but deep insights have also come from experimental studies in animals such as mice. For these reasons, in this book we concentrate on the immune systems of humans and mice. These, along with other more recently evolved organisms (e.g. birds and amphibians), have the most complex and sophisticated immune systems, but the origins of these can in many instances be traced back to the most distant and ancient species in evolutionary history.
In this chapter we provide an overview of immunology in which we introduce the key players in immunity, largely focussing on the immune systems of humans and mice. We start by briefly considering how infection can be sensed by the host organism and how it is possible for a host to recognize many very different infectious agents (Section 1.2). We then introduce the tissues and specialized organs where immune responses occur (Section 1.3). To eliminate different infections effectively, immune responses need to be tailored to particular types of infection. This requires a variety of cells and molecules that can interact coherently to generate the mechanisms that are needed to eliminate each type of infection. As these mechanisms help to bring about or “effect” the elimination of infectious agents they are termed effector mechanisms.
Defence against infection is divided into two main forms termed innate immunity and adaptive immunity. Innate defence mechanisms are present in different forms in all multi-cellular organisms, including plants. Adaptive defence mechanisms have evolved more recently in vertebrates. In vertebrates, the interaction of innate and adaptive immune mechanisms is essential for the generation of effective immunity to infection.
To introduce the mechanisms of immunity we start by describing the different types of immune cells and their function in innate and adaptive immunity to infection (Section 1.4). We then introduce the major classes of molecule involved in functions such as the detection of infection, the recruitment of cells to infected sites, communication between cells and tissues, signalling within cells, and, usually, elimination of the infectious agent (Section 1.5).
The immune system that humans have evolved is, however, not perfect and we discuss some of these imperfections at the end of this chapter (Section 1.6). The immune system is a very effective killing machine, and if it goes wrong it can cause severe disease and even death of its host. To cover these latter areas we first consider how the immune system is able to discriminate between what needs to be eliminated and what does not – particularly in the case of adaptive immunity, which has evolved to recognize molecular structures largely at random. We then introduce the different ways in which the immune system can cause damage if it becomes directed not to infectious agents, but to otherwise harmless targets, including many inert substances around us and within the tissues of the host itself. We discuss the problems of transplants (some of which can even attack their hosts) and why the immune system fails to reject malignant tumours (cancer). Finally, we turn from problems to solutions and introduce two areas in which either the intact immune system and components of immunity can be harnessed for our own benefit and from which tools can be derived to treat disease.
1.2 Host Defence Against Infection
We need immune responses to defend ourselves against infection. Many different kinds of organism have the potential to infect us and, if they do so, can cause us harm in many different ways. To deal with all these potential threats we, as hosts for infectious agents, need a variety of different kinds of host defence mechanisms. Indeed this applies for any living organism.
1.2.1 Infectious Agents
To understand how the immune system works in infection we need to know who the aggressors are. Potentially infectious agents include the following:
- Viruses, which are non-living entities. Common examples are influenza virus, human immunodeficiency virus (HIV) and herpes simplex virus (HSV, which can cause cold sores or genital ulcers).
- Bacteria, are single-celled prokaryotic organisms. Examples include Staphylococcus and Streptococcus that cause acute infections such as abscesses and sore throats, and Mycobacteria that cause chronic infections such as tuberculosis and leprosy.
- Fungi, which are unicellular, such as Candida that causes thrush, or multicellular.
- Parasites, which are eukaryotic organisms. Some are single-celled protozoa that cause diseases such as malaria, others are large, multicellular organisms (metazoa) such as tapeworms.
In this book, for convenience, we will sometimes refer to smaller infectious agents, including viruses, as microbes because they are microscopic in size. However, many parasites, the metazoa, are often far from microscopic in size.
1.2.2 Host Defence
All organisms possess mechanisms to defend themselves against infection, and immunity is a specialized form of host defence. In mammals, defence mechanisms can be passive or active. Passive defence comes in the form of natural barriers that hinder infection. Examples are skin, which prevents access of microbes to the underlying tissue, and gastric acid in the stomach which, not surprisingly, can kill many microbes that might be ingested with food. Their existence is quite independent of the presence of infection. Active defence is brought about by immune responses that involve a diversity of different effector mechanisms that are induced by the presence of infection and which may eliminate the microbe. Thus, all forms of active immunity depend on specific recognition of molecules present in the infecting agent. This is turn leads to a response, involving the interaction of cells and molecules to produce different effector mechanisms that can often eliminate the infection.
Immunity is itself divided into two different forms – innate and adaptive. Innate responses occur rapidly and can generate effector mechanisms that are effective within minutes or hours of infection. In contrast, adaptive immunity takes much longer to become effective, usually over a few days. In immunity to most forms of infection, however, both innate and adaptive immunity are essential. A major advantage of adaptive immune responses, not seen with innate immunity, is that they generate memory – a second infection with the same microbe elicits a stronger, faster and usually more effective response. See Figure 1.1.
Fig. 1.1 Mechanisms of defence against infection. Natural barriers. These stop infectious agents entering the host or provide a hostile environment. Physical barriers to infection include the epithelia of the skin, lung and airways, and the gastro-intestinal and urogenital tracts. Cells in these barriers may also secrete agents that kill infectious agents. Innate immunity. This is the first form of immunity induced by infectious agents. Cells and molecules such as phagocytes and complement can make rapid responses that may eradicate the infection. Adaptive immunity. Later adaptive responses may be generated if the infectious agent is not killed by innate immunity. Cells and molecules such as lymphocytes and antibodies take longer to become effective, but adaptive immunity can also lead to a state of long-lasting resistance to re-infection termed immunological memory (not shown).
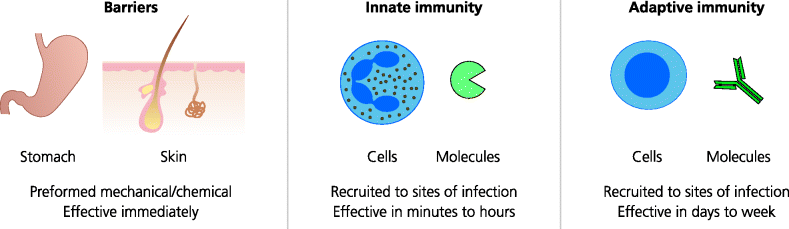
1.2.3 Immune Recognition
Different types of cells and molecules are involved in the initiation of innate and adaptive immune responses although, as mentioned above, their interaction is essential in defence against most infectious agents. So what do the innate and adaptive arms of immunity do in general terms? Broadly speaking we can view some components of the innate immune system as being involved in the detection of “harmful” things that represent “danger” to the organism, such as general classes of microbes that may have infected the host. Other components then endeavour to eliminate the microbe. In contrast, the adaptive immune system can discriminate very precisely between individual microbes, even of the same type, but can generally only make a response if it has been informed by the innate system that what is being recognized is “dangerous”. If so, adaptive responses may then help to eliminate the microbe, if it has not already been eradicated during the earlier innate response. Recognition of infectious agents is essential for any form of immunity and thus for host defence against them. Generally speaking, the types of receptors used for recognition differ in innate and adaptive responses. See Figure 1.2.
Fig. 1.2 Immune recognition. Innate immunity. PRRs directly or indirectly recognize conserved features of infectious agents called PAMPs. PRRs are widely expressed throughout the innate immune system. Adaptive immunity. The two main types of lymphocytes, B cells and T cells, have highly discriminatory receptors for microbial components or antigens, BCRs and TCRs respectively. These recognize antigens in totally different ways. BCRs can be secreted as soluble antibodies and bind to different types of antigen, such as carbohydrates on glycoproteins in their unfolded, native form. In contrast, TCRs generally recognize small peptides, generated by degradation of microbial proteins, in association with specialized presenting molecules (MHC molecules) on the surface of other cells (i.e. as peptide–MHC complexes).
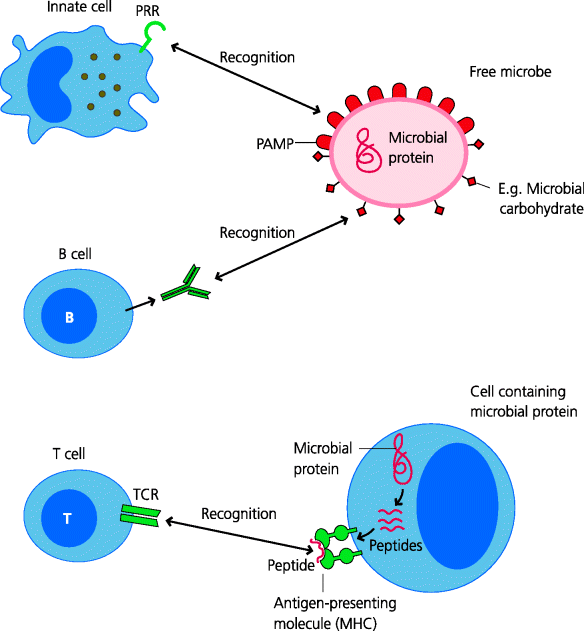
1.2.3.1 Recognition in Innate Immunity: Pattern Recognition Receptors
The key components of the innate immune system include cells such as phagocytes and soluble molecules such as complement. These work together to sense the presence of infection. The recognition of potentially dangerous microbes usually leads to the generation of inflammation, familiar to us all. One way of viewing this is that the innate immune systems of multi-cellular organisms can generate “alarm” signals in response to danger, and that some of these signals cause inflammation. Alarm is not a conventionally used term, but is one that we find helpful and therefore will use it from time to time in this book. Inflammation enables effector cells and molecules to be targeted to the site of infection. As noted above, other signals generated during innate responses can also determine whether, and in what way, the lymphocytes of adaptive immunity will respond.
The recognition of infectious agents in innate immunity is mediated by germline-encoded receptors called pattern recognition receptors (PRRs). These receptors generally recognize conserved features of infectious agents that are often shared by different classes of microbes, these microbial features are called pathogen-associated molecular patterns (PAMPs). PAMPs directly or indirectly stimulate innate immune responses by acting as agonists for PRRs. An agonist is anything that stimulates a response through a receptor, as opposed to an antagonist that inhibits it. PAMPs may bind directly the PRRs, therefore acting directly as ligands for these receptors, but some PAMPs can trigger responses by binding to a different molecule that then associates with a PRR, so it is useful to use the general term agonist. This also allows us to discriminate clearly between components of microbes that trigger innate responses, and molecular structures which are recognized in adaptive immunity that are termed antigens (below).
The cells responsible for initiating activation of the innate immune system are widely distributed in tissues and organs, and they possess many copies of different types of PRR that trigger rapid responses. This allows very rapid activation and deployment of the effector mechanisms of innate immunity. In many cases the innate system can eliminate the infectious microbe, often without any symptoms occurring (i.e. sub-clinically), and if there has been damage to the tissues at the site of infection the innate system will initiate repair and healing. Importantly, activation of the innate system is also essential for the triggering of adaptive immune responses
1.2.3.2 Recognition in Adaptive Immunity: Antigen Receptors
The key components of the adaptive immune system are the lymphocytes. It is convenient at this stage to divide these into two main groups (other types do exist). One group is the T lymphocytes (T cells) which have evolved to interact with other cells. The other is the B lymphocytes (B cells) which are the precursors of cells that can make soluble antibodies. The recognition of molecules from infectious agents by lymphocytes is mediated by their specialized antigen receptors, which are not present on cells of innate immunity. An antigen can be defined as a molecular structure against which a specific adaptive immune response can be made. In contrast to PRR agonists in innate immunity (above), the antigens which stimulate lymphocyte responses are generally unique to particular infectious agents no matter how closely they are related.
Collectively, lymphocytes express a vast range or repertoire of antigen receptors of different specificities, but each lymphocyte expresses multiple copies of a receptor of only a single given specificity. The term specificity relates to the particular antigen(s) that each lymphocyte is able to recognize. These receptors are generated by rearrangement of germline DNA, a process that is not known to occur for any other type of molecule. Their specificity is generated largely at random and in advance of any infection. Lymphocyte recognition of antigen is thus anticipatory. Lymphocyte antigen receptors are highly discriminatory and distinguish between even very small differences in antigens, such as an amino acid substitution in a peptide or a specific side chain in an organic molecule.
The vast repertoire of T and B cell antigen receptors means that a lymphocyte expressing a particular receptor is exceedingly rare. In addition, lymphocytes are normally small, relatively inactive cells. Thus, adaptive immune responses require the activation and proliferation of specific clones of B cells or T cells in order to reach a critical mass that can deal with the infectious agents and this takes time. Adaptive responses also need to generate the particular effector mechanisms that are most suited to eliminate the infection and this also takes time. Hence, adaptive immune responses are generally slower to be triggered than innate responses. However, following infection, increased numbers of antigen-specific lymphocytes remain in the body and these provide stronger, more rapid responses should re-infection occur.
1.2.3.3 Types of Recognition in Innate and Adaptive Immunity
Earlier, we suggested that some components of innate immunity can be viewed as recognizing danger, and that in general they are able to discriminate between harmful and harmless stimuli. Danger can be represented by the presence of an infectious agent or by signs of cell damage or stress that may or may not be associated with infection. In contrast, the antigen receptors of lymphocytes enable them to discriminate very precisely between what is “self” (any normal component of the host) and “non-self” (such as a component of a microbe), but not between harmless and harmful. Therefore, it is primarily the signals generated by the innate system that inform lymphocytes as to whether the antigens that they are recognizing originate from harmful or harmless agents, and hence determine whether or not these lymphocytes become activated and also precisely how they need to be activated in the context of the specific danger that is posed. If they are instructed that there is no danger (or not instructed that there is danger), then lymphocytes become unresponsive, or “tolerant”, to what they are recognizing (Section 1.6.1).
1.2.4 Stages of Immunity
Immune responses to infectious agents involve a sequence of events that usually occur in distinct anatomical compartments. Immunologists divide tissues into lymphoid tissues, on one hand, and non-lymphoid or peripheral tissues, on the other. As we shall see (below), lymphocytes are produced in primary lymphoid tissues, but tend to localize in specialized sites, the secondary lymphoid tissues. In contrast, the cells of innate immunity are distributed throughout all tissues and organs. Once the natural barriers are breached by an infectious agent, innate agonists such as PAMPs, derived from the infecting agent, trigger the activation of innate immunity. Infections most commonly start in peripheral, non-lymphoid tissues, and it is the resident innate cells such as macrophages and mast cells in these tissues that first recognize innate agonists. The subsequent inflammatory response is then crucial for the rapid recruitment of other innate effector cells and molecules, such as specialized phagocytes and complement, into the site of infection to help eliminate the microbes that are present there.
Adaptive immunity is also initiated by the infection and becomes evident a few days to a week later. For this to happen, antigen needs to be transported to secondary lymphoid tissues (e.g. to lymph nodes or spleen; below) during the innate phase so that it can be recognized by lymphocytes that tend to congregate in these organs. The molecules responsible for antigen recognition are the respective antigen receptors of the two main groups of lymphocytes: T cell receptors (TCRs) and B cell receptors (BCRs, that can later be secreted as antibodies). The activated lymphocytes then either become effector cells themselves (e.g. some T cells develop into cytotoxic cells that can kill virally infected cells, or B cells develop into plasma cells that secrete antibodies) or they can recruit and activate other effector cells at the site of infection, often including cells of innate immunity. Together, these different effector mechanisms, which are recruited into sites of infection because of inflammation, in most cases control and ultimately clear the infection. Finally, as noted above, in most forms of adaptive immunity, memory lymphocytes are generated in lymphoid tissues. Once induced, memory T and B cells localise to non-inflamed peripheral tissues or secondary lymphoid organs and, in the case of re-infection with the same organism, generate rapid, stronger adaptive responses. See Figure 1.3.
Fig. 1.3 Stages of immunity. Innate and adaptive immunity are closely interlinked. Specialized local (“alarm”) cells of innate immunity can sense the presence of infectious agents. Consequent inflammation enables blood-borne innate effector cells and molecules to enter the tissue. (a) Dendritic cells (DC) at the site of infection sense the presence of an infectious agent and capture molecules (antigens) from it. (b) They migrate into secondary lymphoid tissues and activate lymphocytes that are specific for the infectious agent. (c) Some lymphocytes then make antibodies that circulate in blood to the site of infection and attack the infectious agent; other lymphocytes enter sites of infection and help or recruit other cells to kill the infectious agent, or directly kill infected cells.
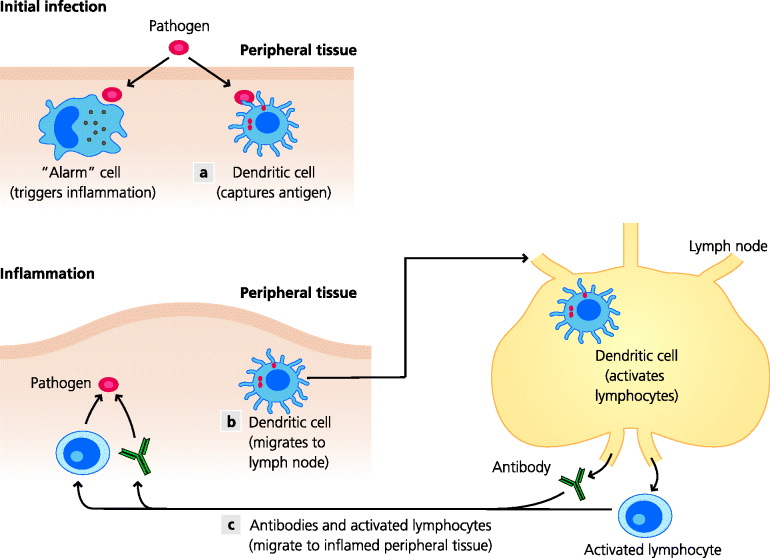
1.3 Anatomical Basis of Immunity
Immune responses occur in complex living organisms. Infection has driven the evolution of tissues and organs in which these responses take place. To understand immune responses requires an understanding of the structure of these tissues and organs in relation to their immune functions. For the benefit of readers who have little or no background in anatomy and histology we will first give a very general introduction to this area.
1.3.1 Peripheral Tissues
For immunologists, peripheral tissues refer to most tissues and organs of the body. They exclude primary and secondary lymphoid organs (below). A generalized tissue or organ is covered by an epithelium, a continual layer of cells that is often in contact with the external environment. Examples are the outer layers of the skin, and the lining of the gastro-intestinal, respiratory and urogenital tracts. Underlying the epithelium there is usually a basement membrane and under this lies loose connective tissue containing cells such as macrophages, mast cells and fibroblasts, and structural components such as collagen fibres (e.g. Figure 1.10).
All tissues and organs are vascularized they have a blood supply. It is crucial for water and solutes, such as nutrients and dissolved oxygen, to be able pass through the linings of blood vessels into the surrounding tissues, so that the cells in these extravascular sites can be nurtured and maintained. This extravascular fluid with its solutes then needs to be collected, as lymph, and returned back into the blood. Therefore, all tissues and organs contain vessels of two types: blood vessels and lymphatics (see Figure 1.4).
Blood leaving the heart is brought to tissues by arteries, which subdivide to form arterioles and ultimately capillaries. The latter are the sites where molecules normally diffuse into and from extravascular tissues. Capillaries join to form venules, these are the vessels where cells migrate into tissues under both normal and inflamed conditions. The capillarity then join to form venules that join to form veins, which ultimately return the blood to the heart. Lymphatics originate from blind endings in tissues. They collect molecules as well as motile cells from the extravascular spaces in tissues and transport them to lymph nodes in afferent lymph. Lymph nodes serve as filters and are sites where adaptive immunity can be initiated. Efferent lymph, leaving the lymph nodes, then carries cells and molecules into larger vessels. The main one is the thoracic duct, which then drains back into the blood.
Fig. 1.4 Blood and lymph. Blood transports water, oxygen and small molecules that diffuse into extravascular tissues across endothelial cells of small blood vessels. Blood also carries leukocytes (white blood cells), but these cells, and larger molecules such as antibodies, can only enter tissues in large amounts at inflammatory sites (Figure 1.3). Extravascular fluid collects into lymphatic vessels and ultimately re-enters blood. Lymph also transports cells from sites of infection into lymph nodes, enabling adaptive immunity to be triggered (Figure 1.3).
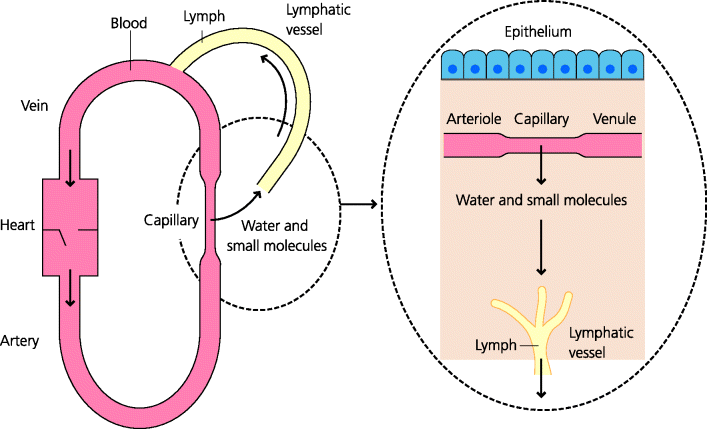
During immune responses the blood acts as a delivery system. Blood vessels and lymphatics are lined by flattened endothelial cells. The blood transports leukocytes, which are the white blood cells as well as erythrocytes, which are the red blood cells (RBCs). If molecules on the plasma membranes of leukocytes recognize complementary molecules on the endothelium, they can adhere to it and may subsequently migrate into the extravascular spaces of the local tissue. As noted above, some small molecules can normally diffuse freely out of the blood in capillaries and venules, but most macromolecules are retained in the blood. Such macromolecules can, however, be delivered selectively to sites of inflammation because gaps open up between venule endothelial cells at these sites (e.g. Figure 1.6).
Some tissues are called “mucosal”. In general, but not always, this is because their epithelia contain goblet cells which secrete mucus onto the epithelial surface; mucus acts as a lubricant and as a barrier to infection. Mucosal tissues include the gastro-intestinal tract, the respiratory and urogenital tracts, the eye, and the lactating mammary gland. From an immunological point of view mucosal tissues are important because they are associated with immune responses that differ in important respects from those associated with other peripheral tissues.
1.3.2 Lymphoid Tissues
Lymphoid tissues are those whose major functions are associated with adaptive immune responses. Primary lymphoid tissues are sites where lymphocytes are produced. In adult humans they include the bone marrow and a specialized organ called the thymus, which is the organ in which T cells develop. The bone marrow is also the site where other leukocytes are generated, such as monocytes (which can develop into macrophages) and granulocytes (which are themselves of different types). The process of generating blood cells is called haematopoiesis (below), so the bone marrow can also be termed a haematopoietic organ. In contrast, the secondary lymphoid tissues are the sites where adaptive immune responses are initiated and regulated. These tissues include lymph nodes, which are distributed throughout the body, and the spleen. There are also specialized secondary lymphoid tissues associated with mucosaecalled mucosal-associated lymphoid tissues (MALTs), such as Peyer’s patches in the small intestine and the tonsils and adenoids in the throat and nose. See Figure 1.5.
Fig. 1.5 Primary and secondary lymphoid tissues. (a) Primary lymphoid tissues are sites where lymphocytes are produced. In adult humans and mice the main sites are the bone marrow and the thymus, where B cells and T cells, respectively, undergo most or all of their development. (b) Secondary lymphoid tissues or organs are sites where adaptive responses are induced and regulated. The main tissues are lymph nodes, the spleen and specialized MALTs such as the Peyer’s patches in the small intestine. All have highly organized areas containing T cells and B cells. Lymphocytes recirculate between blood and lymph and can monitor different anatomical compartments for infection and mount appropriate responses.
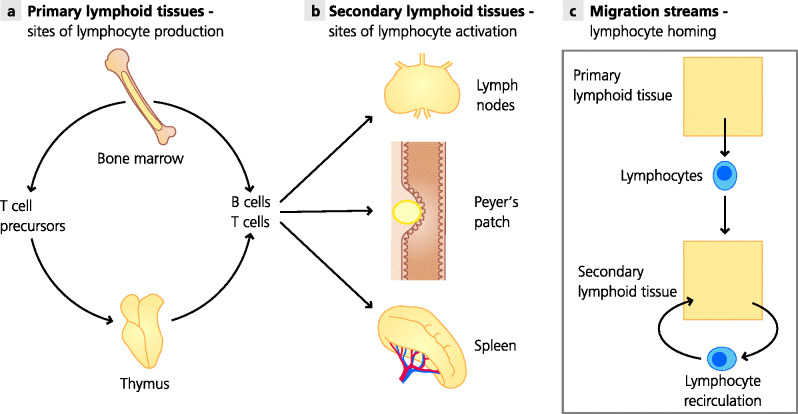
1.3.3 Inflammatory Sites
Usually, recognition of infection in a peripheral site by the innate immune system leads to local inflammation. This is clinically evident as reddening, heat, swelling and pain at the site of infection. One of the main functions of inflammation is to ensure that the cells and molecules needed to deal with the infecting agent are delivered to the right place at the right time. These include, for example, specialized phagocytes and complement components and, often later, lymphocytes and antibodies.
Fig. 1.6 Acute and chronic inflammation. (a) Acute inflammation. Infectious agents such as extracellular bacteria trigger inflammation that starts quickly and lasts a relatively short time. The main features are recruitment of cells and molecules such as neutrophils and complement, and the accumulation of extravascular fluid (oedema). (b) Chronic inflammation. Infectious agents that are not quickly eliminated and which trigger adaptive immune responses can lead to chronic inflammation that can last much longer. The main features are recruitment of blood monocytes, which become macrophages, and activated lymphocytes, especially T cells, and sometimes the development of long-lasting granulomas.
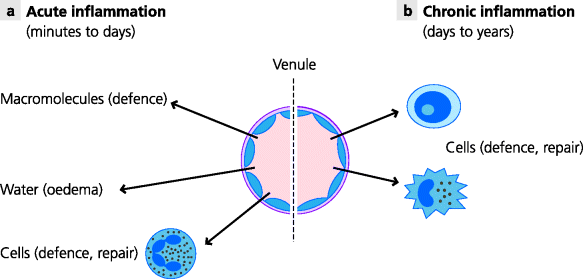
If the infectious agent can be eliminated rapidly (within days or a week or so) the response is known as acute inflammation, and repair and healing of the tissue follows. Typical examples known to almost everyone are abscesses (boils) and sore throats. If, however, the infectious agent persists, continuing inflammation, termed chronic inflammation, leads to continuing tissue damage. This is typically seen with infections such as tuberculosis which may persist for months or years (See Figure 1.6).
More distant sites can also be affected by inflammation at sites of infection, these changes are called the systemic effects of inflammation. Thus, the bone marrow can be stimulated to produce more leukocytes, the liver to produce larger amounts of soluble effector molecules that can contribute to eliminating the infectious agent and the hypothalamus in the brain to induce fever.
1.4 Cellular Basis of Immunity
The primary function of the immune system is to eliminate infectious agents that have breached the natural barriers. This is brought about by the integrated actions of different cells and molecules that, directly or indirectly, lead to recovery from infection. It is the effector cells and molecules that actually bring about the elimination of infectious agents. To simplify understanding; we focus first on the cellular basis of immunity, before turning to molecules involved in immunity in Section 1.5. This is an artificial distinction, since cells and molecules are usually both involved in immune responses, as will be seen in the rest of this book.
1.4.1 Origin of Immune Cells
1.4.1.1 Haematopoiesis
Haematopoiesis is the name given to blood cell development. In the adult mammal this takes place primarily in the bone marrow (also a primary lymphoid tissue), but in the foetus this happens in the liver. The earliest cells in haematopoiesis are the multi-potential stem cells, which can give rise to any and all blood cells. When cells divide each of the progeny cells is usually of the same type. Stem cells are unusual in that the one of the progeny cells is another stem cell, thus maintaining this population, while the other can start to differentiate into a different cell type, ultimately producing all the cells of different lineages.
Initially stem cells divide to form cells that can either give rise to different types of “myeloid” cells or “lymphoid” cells – these are the common myeloid progenitor(CMPs) and common lymphoid progenitors (CLPs). In turn, the former precursors can ultimately produce monocytes and macrophages, different types of granulocytes, and other cells including erythrocytes (RBCs), megakaryocytes (the precursor of blood platelets) and some DCs. In contrast, the latter give rise to the lymphocytes (T cells and B cells) as well as natural killer (NK) cells and other DCs. The development of cells in each pathway is regulated by growth factors that are more or less restricted in their activities. Thus, interleukin (IL)-3 and stem cell factor (SCF) stimulate stem cells which can develop into many different lineages, granulocyte macrophage colony-stimulating factor (GM-CSF) preferentially stimulates development of monocytes, granulocytes and some DCs, and erythropoietin stimulates red cell development. See Figure 1.8.
Fig. 1.7 Haematopoiesis. All blood cells are formed from haematopoietic stem cells. On division they form another stem cell (self renewal) and a more committed precursor for a blood cell. In turn, the CLP gives rise to different types of lymphocyte as well as natural killer (NK) cells, while the CMP can generate monocytes and macrophages, granulocytes, and other cell types. The production of different blood cells is regulated by different growth factors and is largely under feedback control.
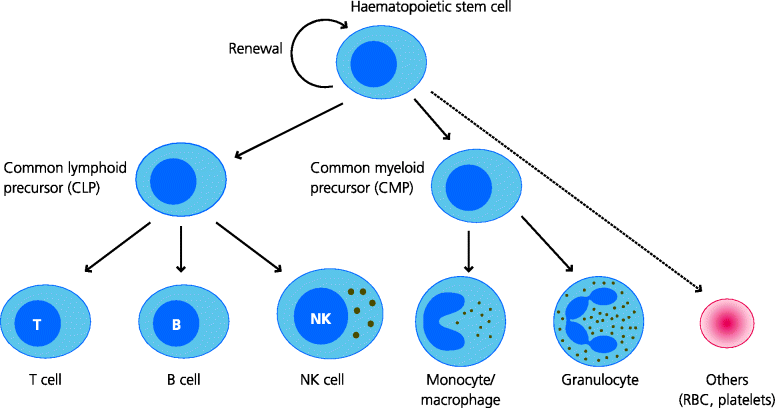
1.4.1.2 Lymphopoiesis
The term lymphopoiesis refers to the generation of lymphocytes, in contrast to myelopoiesis which refers to generation of myeloid cells (above), and thus can be viewed as a subset of haematopoiesis. In the adult, common lymphoid precursors are present in the bone marrow. B cells start to differentiate in the bone marrow and are released as partially mature (transitional) B cells into the blood. T cell precursors, however, migrate directly to the thymus and T cells complete their development in this organ (a primary lymphoid tissue). It is important to realize that lymphocytes can also undergo further rounds of division and development outside the primary lymphoid organs. This normally happens after they have recognized the antigens for which they are specific, such as molecular components of infectious agents and occurs in organs such as lymph nodes, spleen and Peyer’s patches (secondary lymphoid organs). The phenomenon of clonal expansion is an essential feature of adaptive immune responses for it enables clones of specific lymphocytes to expand and reach a critical mass that can now help to deal effectively with eliminating the infection.
1.4.1.3 Cell-Mediated Immunity
The principal mechanisms by which cells can help to defend against infection are the following:
These cellular responses, which contribute to cell-mediated immunity as a whole, need to be tightly regulated and coordinated and, quite often, different cells need to collaborate to help to bring about an overall response that lead to the elimination of an infectious agent. See Figure 1.8.
Fig. 1.8 Mechanisms of cell-mediated immunity. There are three main ways in which cells can eliminate infectious agents or protect against infection. (a) Direct killing. Specialized phagocytes, mainly macrophages and neutrophils, can internalize microbes such as bacteria and destroy them intracellularly. (b) Killing of infected cells. NK cells and cytotoxic T cells can kill infected cells, preventing the replication and release of microbes such as viruses. (c) Maintaining natural barriers. Larger parasites, such as intestinal worms, cause damage to the epithelium, risking entry to the body. Cells such as eosinophils can help to repair the damage and, in some cases, may help to expel the organisms or kill it by secreting toxic substances.
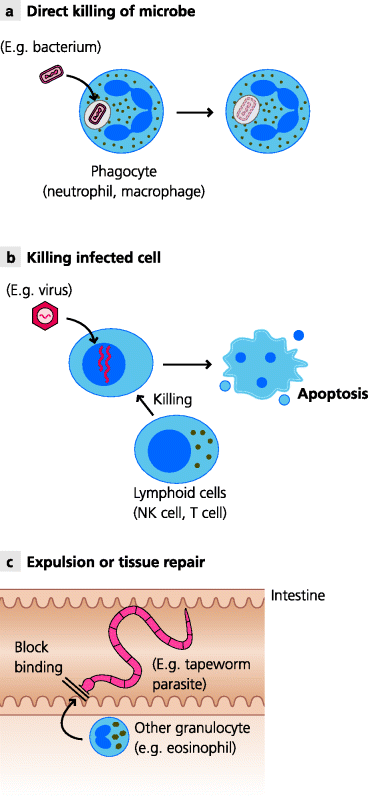
1.4.1.4 Introduction to Cells of the Immune System
The different cells of the immune system can be classified according to overlapping principles. The most important classification is functional – what do they do? This relates to other aspects – their developmental origins and relationships, and their anatomical distribution in the body. Cells are also classified by their morphology, but this can be misleading – naïve B and T cells look more or less the same under the light microscope – and it is more accurate to combine morphology with other approaches such as identification of the surface molecules that the cells express. Based on the these criteria we will now briefly introduce the major groups of cells involved in immune responses before discussing each in more detail. We will divide the cells into those primarily thought of as belonging to innate immunity and those of adaptive immunity, while emphasizing that in reality there is much overlap between the two groups.
1.4.1.5 Cells of Innate Immunity
Macrophages
These are divided into two main types:
- Resident macrophages are present in steady-state tissues (i.e. before infection occurs) and can detect the presence of microbes. In turn they can help to trigger inflammation.
- Recruited (or elicited) macrophages are not tissue-resident cells, but they develop from circulating precursors called monocytes that can be recruited into sites of infection. After development into macrophages they can act as effector cells to help eliminate the infection.
Macrophages are one of the two main types of specialized phagocyte that can engulf and internalize (phagocytose), and subsequently kill microbes such as bacteria.
Mast Cells
These cells also reside in steady-state tissues and can detect the presence of microbes. Mast cells contain granules that are discharged when they are stimulated, and the granule contents can contribute to triggering of local inflammation.
Granulocytes
These are the major populations of blood leukocytes, the circulating white blood cells. They are called granulocytes because they contain cytoplasmic granules that are visible under the light microscope. They have nuclei that possess two or more lobes, and are thus also called polymorphonuclear leukocytes to distinguish them from monocytes and lymphocytes, the mononuclear leukocytes, which do not have lobulated nuclei. Granulocytes are divided into three groups derived from a common precursor: neutrophils, which are abundant in blood and are a very important type of phagocyte; the rarer eosinophils, and the basophils that are somewhat related to mast cells in function. A crucial feature of granulocytes is that they normally circulate in the blood but can be recruited selectively into inflammatory sites in response to different types of infection. (Note that although mast cells also contain granules, they do not circulate in the blood in a mature form and are therefore by definition not included in the term granulocyte; they may also originate from a distinct progenitor.)
NK Cells
NK cells are developmentally related to lymphocytes, but differ in many aspects from them. NK cells are present in tissues as resident cells and can also be recruited to sites of inflammation. They can kill other cells, such as virally infected cells (i.e. they have cell-killing (cytotoxic) activity) and they also regulate immune responses.
1.4.1.6 Cells of Adaptive Immunity
Lymphocytes
These are the primary cells involved in adaptive immune responses. As noted earlier, they have highly discriminatory antigen receptors and are divided into two main groups: B cells and T cells. B cells are the precursors of plasma cells that secrete antibodies. T cells are themselves divided further into two main groups: CD4 T cells that function principally as regulator and coordinator cells in adaptive immune responses, and CD8 T cells that can develop into cytotoxic cells with the capacity to kill cells infected with viruses or other microbes. Cytotoxic T lymphocytes (CTLs) and NK cells, noted above, are the two main types of cytotoxic cells in the immune system.
1.4.1.7 Cells that Directly Link Innate and Adaptive Immune Responses
DCs are another cell type, which actually exist as several subgroups. Most of these are involved in the initiation and regulation of adaptive immune responses. They can be viewed as linking innate and adaptive immunity because they respond quite rapidly to the presence of infection and subsequently change their properties so they can activate lymphocytes, particularly the CD4 T cells.
1.4.2 Phagocytes
All cells in the body are capable of sampling their extracellular milieu in a general process called endocytosis. They may do so by internalizing small samples of fluid and solutes into membrane-bound vesicles called endosomes. This is the process of fluid-phase endocytosis or pinocytosis. It is used by cells, for example to obtain nutrients, and in some cases cell surface receptors extract the required nutrient molecules in a related process called receptor-mediated endocytosis. Some specialized cells are also capable of internalizing particles through the process of phagocytosis; the particles are contained in membrane-bound vesicles called phagosomes. In all forms of endocytosis, including phagocytosis, membrane-bound vesicles called lysosomes, containing catabolic enzymes, can fuse with the endocytic vesicle or the phagosome and release the enzymes into the vesicle. Generally speaking this usually leads to the degradation of the vesicular contents. Note that the term endocytosis can be used in a general sense to include phagocytosis (as we do), but is sometimes used for all processes other than phagocytosis. See Figure 1.9.
Fig. 1.9 Mechanisms of endocytosis. Phagocytosis. Phagocytes can internalize particles such as bacteria into vesicles called phagosomes. Pinocytosis. All cells engulf small quantities of fluids and their dissolved solutes. These are taken up in membrane-bound vesicles called (primary) endosomes. In all cases, the primary endosomes or phagosomes then fuse with other intracellular vesicles called lysosomes to form secondary endosomes or phagolysosomes in which the internalized contents can be degraded. This can lead to killing of phagocytosed microbes.
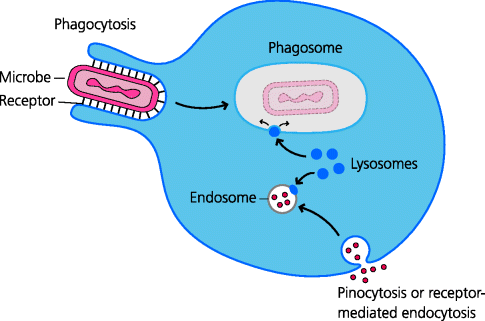
Phagocytes are specialized cells that are able to internalize particles such as bacteria and small protozoa, and are able to kill microbes intracellularly. The two main classes of phagocytes are macrophages and neutrophils. Other cells such as eosinophils are also weakly phagocytic, but this is not known to be one of their main purposes.
1.4.2.1 Macrophages
Macrophages reside in almost every tissue of the body and are important components of both innate and adaptive immune responses. Circulating precursors of macrophages, which are produced in the bone marrow, are called monocytes. When a monocyte enters a tissue it may develop into a form called mature macrophage.
Macrophages in tissues are generally long-lived cells (tattoos, which last for a lifetime, represent ink particles inside macrophages). Resident macrophages in connective tissues and solid organs play crucial roles in the remodelling and growth of organs during development and in regulation of normal tissue functions (homeostasis). Tissue-resident macrophages possess specialized receptors that recognize cells that have been programmed to die through the process of apoptosis and assist in tissue remodelling without scarring (e.g. during embryogenesis).
Infection most often begins in peripheral tissues and resident macrophages play very important roles in the initiation of innate immune response. They possess receptors (PRRs) that can recognize different types of infectious agent, enabling them to distinguish between broad classes of viruses, bacteria and fungi for example. Some of these receptors enable the macrophage to phagocytose the infectious agent so it can be destroyed intracellularly. Importantly, other PRRs signal to the nucleus to change gene expression, leading, for example, to the secretion of small, hormone-like proteins (cytokines; below) which act on other cell types. Crucially, some of the cytokines that resident macrophages secrete early in infection (as alarm signals; Section 1.2.3.1) then help to stimulate local inflammation by modifying the structure and function of the endothelial cells of blood vessels. Later they may also stimulate more distant tissues, inducing systemic inflammatory responses. See Figure 1.10.
Fig. 1.10 Types of macrophages. Macrophages are plastic cells that can develop different specialized functions. Tissue-resident macrophages are long-lived cells present in connective tissues. In the steady state they are involved in homeostasis, helping to maintain the architecture of the tissue, and can also sense infectious agents and help to trigger inflammation (e.g. Figure 1.3). Inflammatory macrophages. Local inflammation recruits blood monocytes into tissues. In response to infectious agents these can become highly secretory cells that produce many innate effectors such as complement components. Activated macrophages. Innate and adaptive responses can help turn macrophages into potent anti-microbial cells. Alternatively activated macrophages. Macrophages can also develop functions involved in wound repair and healing, and may help to dampen down the inflammation.
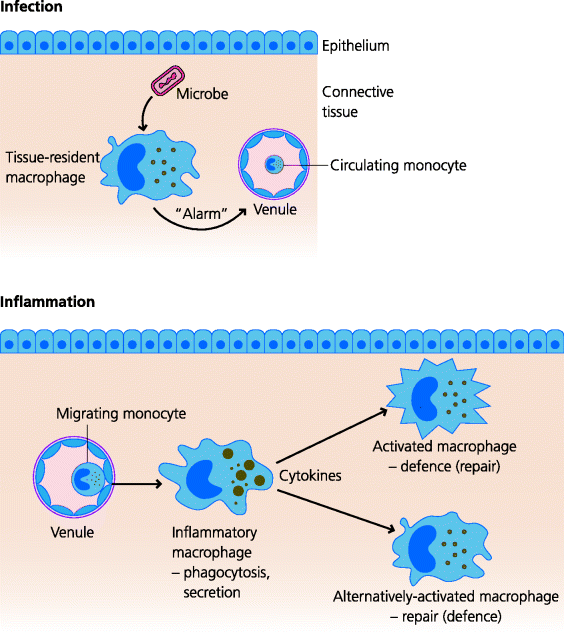
Once the inflammatory process has been initiated, large numbers of monocytes can be recruited from the blood to the site of infection. These cells develop into inflammatory macrophages with a much larger repertoire of functions than the resident cells. (Depending on how they are being studied these cells are sometimes also called elicited macrophages.) For example, they are much more phagocytic and secrete an enormous diversity of molecules that help to amplify the inflammatory response, bring in other types of effector cells to the area and eliminate the infectious agent. These and other macrophages may also contribute to the repair and healing of the tissue.
In some circumstances macrophages can acquire new and highly potent antimicrobial killing mechanisms. This is particularly the case once the later adaptive immune responses have been initiated, although it can happen earlier. A key macrophage-activating agent, produced in such responses is the cytokine interferon (IFN)-γ. Activated macrophages do not have the ability to produce the wide variety of different mediators typical of elicited macrophages, but they concentrate on killing the microbes they have phagocytosed. For this they produce, for example, nutrient-depleting molecules that prevent growth and replication of the microbes, highly toxic agents that poison them, including reactive oxygen intermediates/species (ROIs/ROS), and a diversity of enzymes that digest them. Activated macrophages are very important in defence against certain bacteria, such as the mycobacteria, some of which cause tuberculosis and leprosy. In other circumstances different types of macrophage activation (e.g. alternative activation) or deactivation can be triggered, perhaps as sequential stages, depending on the local environmental conditions (e.g. which cytokines are present). You can see that the macrophage is therefore a highly adaptable cell type.
1.4.2.2 Neutrophils
Neutrophils are the most abundant leukocytes in human blood, comprising approximately 70% of total white blood cells. Neutrophils are crucial in defence against pyogenic (pus-forming) bacteria such as Staphylococcus and Streptococcus which typically cause abscesses and similar infections involving acute inflammation. Neutrophils are released from the bone marrow as mature cells and have very short lifespans (1–2 days). They are not present in normal tissues, but are rapidly recruited to sites of acute inflammation.
Neutrophils are highly phagocytic. They constitutively possess a wide variety of antimicrobial killing mechanisms (in contrast to macrophages, in which these are usually induced). Many of the molecules needed to generate killing mechanisms are preformed and stored in different types of cytoplasmic granules. When a microbe has been phagocytosed, some of these granules fuse with the phagosome, delivering their toxic contents – some of which are similar to those of activated macrophages (e.g. ROIs) – onto the microbe. Neutrophils may also have the capacity to kill microbes extracellularly. In addition to their anti-microbial agents the granules also contain proteolytic enzymes that breakdown (liquefy) the surrounding tissue. When neutrophils encounter bacteria that are able to resist killing, they die and in some infections, typically with Staphylococcus aureus, the liquefied tissues form the pus that discharges from abscesses. See Figure 1.11.
Fig. 1.11 Killing by neutrophils. Neutrophils can kill microbes intracellularly and perhaps extracellularly. Intracellular killing. After phagocytosis of microbes the phagosomes fuse with cytoplasmic granules that contain or can produce anti-microbial agents. The latter include reactive oxygen intermediates and other compounds that may be toxic to the microbes and proteases which may degrade them. Extracellular killing. These cells can also extrude neutrophil extracellular traps (NETs) composed of chromatin that are thought to trap extracellular microbes. The contents of other granules may subsequently discharge to release anti-microbial agents and proteases to help kill them, although this is not yet proven.
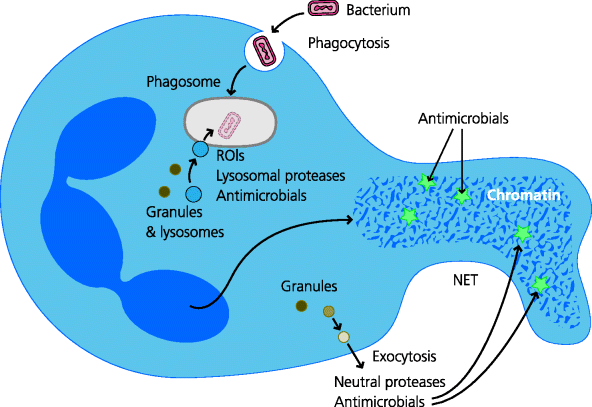
1.4.3 Mast Cells, Eosinophils and Basophils
As for the different phagocytes (Section 1.4.2), the functions of mast cells, eosinophils and basophils significantly overlap, although each, too, has its own specialized properties. Mast cells can be viewed as being resident cells in tissues, whereas the basophils and eosinophils are typically recruited to inflammatory sites. They are commonly found in the anatomical barriers of the body e.g. immediately under mucosal epithelia or in the skin.
1.4.3.1 Mast Cells
Mast cells are resident cells in mucosal tissues and loose connective tissues. They function, at least in part, by sensing infection or tissue damage and then triggering inflammation. Mast cells possess cytoplasmic granules that contain histamine and cytokines. Mast cells may be activated through their PRRs and by small molecules such as complement fragments. When activated these cells very rapidly release their stored mediators, but also synthesize cytokines and lipid mediators. These secreted molecules are generally involved in the induction of acute inflammation. The lipid mediators also induce contraction of smooth muscle, such as in the lung and airways and in the gut. In the latter case, muscle contraction may help expel parasites. Mast cells are, however, better known for the roles they play in pathological, allergic responses. See Figure 1.12.
Fig. 1.12 Mediators produced by mast cells. Mast cells are present in all connective tissues. Mast cells have large cytoplasmic granules that store pre-formed inflammatory mediators such as histamine and cytokines, and which are discharged when the mast cell is activated (degranulation). Activated mast cells also synthesize inflammatory mediators including lipid metabolites and other cytokines when activated. Mast cells can be activated by pathogen molecules or by molecules produced during acute inflammation. They can also be activated during allergic reactions such as hay fever.
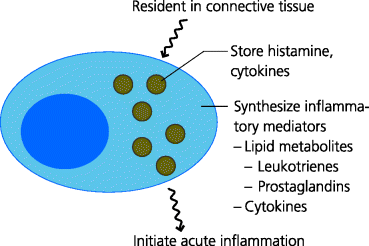
1.4.3.2 Eosinophils
These granulocytes, normally representing 1–3% of all blood leukocytes, usually possess a bi-lobed nucleus and large cytoplasmic granules of different types that stain orange with the acidic dye, eosin (hence their name). They are recruited to certain types of inflammatory sites, particularly those associated with parasitic infections and allergic responses. As for mast cells, the contents of their granules are released when eosinophils are activated by cytokines. Other mediators can be synthesized later. Several functions of eosinophils overlap with those of mast cells (above). These include inducing the increased production of mucus, which may help prevent attachment of infectious agents to the underlying epithelial cells. Eosinophils may have a role in healing certain types of tissue damage, such as that caused by intestinal infestation of worms. Thus, eosinophils may be important in defence against parasites such as intestinal worms. See Figure 1.13.
Fig. 1.13 Types of granulocytes. Granulocytes are leukocytes that contain different cytoplasmic granules with characteristic staining properties. All can be recruited to different sites of inflammation. Neutrophils are the most abundant leukocytes in blood. Large numbers can be recruited rapidly to sites of acute inflammation (Figure 1.6) and their production from the bone marrow can be greatly increased during some bacterial infections. They are highly active phagocytes (Figure 1.11). Eosinophils are normally present in low numbers, but can be recruited particularly into mucosal tissues. Larger numbers can be produced from the bone marrow during other types of infection, especially with large parasites against which they may provide resistance or defence, and in allergic responses such as asthma. Basophils are normally very rare in blood. They, too, can be recruited to inflammatory sites where they typically accompany eosinophils. They have very similar properties to mast cells but their roles in host defence are poorly understood.
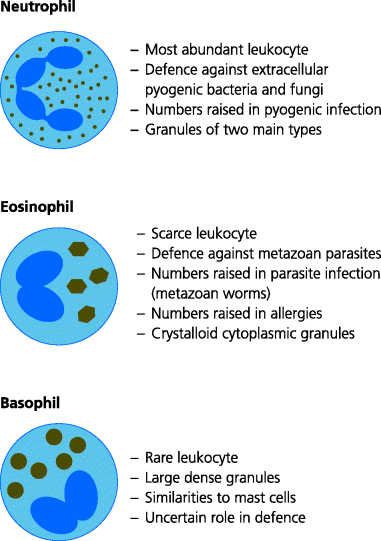
1.4.3.3 Basophils
Basophils possess bi-lobed nuclei and their large cytoplasmic granules stain dark blue with basic dyes (hence the name). Basophils circulate in the blood in very small numbers (normally less than 1% of leukocytes) and are normally absent from tissues. However, they can be recruited into inflammatory sites, typically together with eosinophils (above). They are functionally similar to mast cells, although there are some differences, and basophils are also potent stimulators of inflammation.
1.4.4 Natural Killer Cells
All viruses need to infect cells in order to replicate. To limit viral replication and the release of new virions, immune cells have evolved that can kill virally infected cells and perhaps some tumour cells. The ability of an immune cell to kill another cell in this way is termed cellular cytotoxicity and the cell that does the killing is generally referred to as a cytotoxic cell. Such cells kill by triggering a process called apoptosis in the cells they recognize. All cells have the potential to undergo apoptosis (e.g. during tissue remodelling) and some specialized cells of the immune system have evolved mechanisms to latch on to this process; they therefore kill by enforced suicide rather than murder of their targets. The advantage of triggering this form of cell death is that the contents of the dead and dying cell can be rapidly eliminated, usually without triggering inflammation. One type of cell that can do this, although it may not be its principal function in host defence, is the NK cell. natural killer (NK) cells were first recognized by their ability to kill tumour cells in culture as soon as they were isolated from blood. They are present in normal blood and organs such as spleen and liver, but other NK cells can be recruited to inflammatory sites. Owing to their spontaneous cytotoxic capacity, they were termed natural killer cells – as opposed to cytotoxic T cells (CTLs) that need to be activated before they can kill (Section 1.4.5.2).
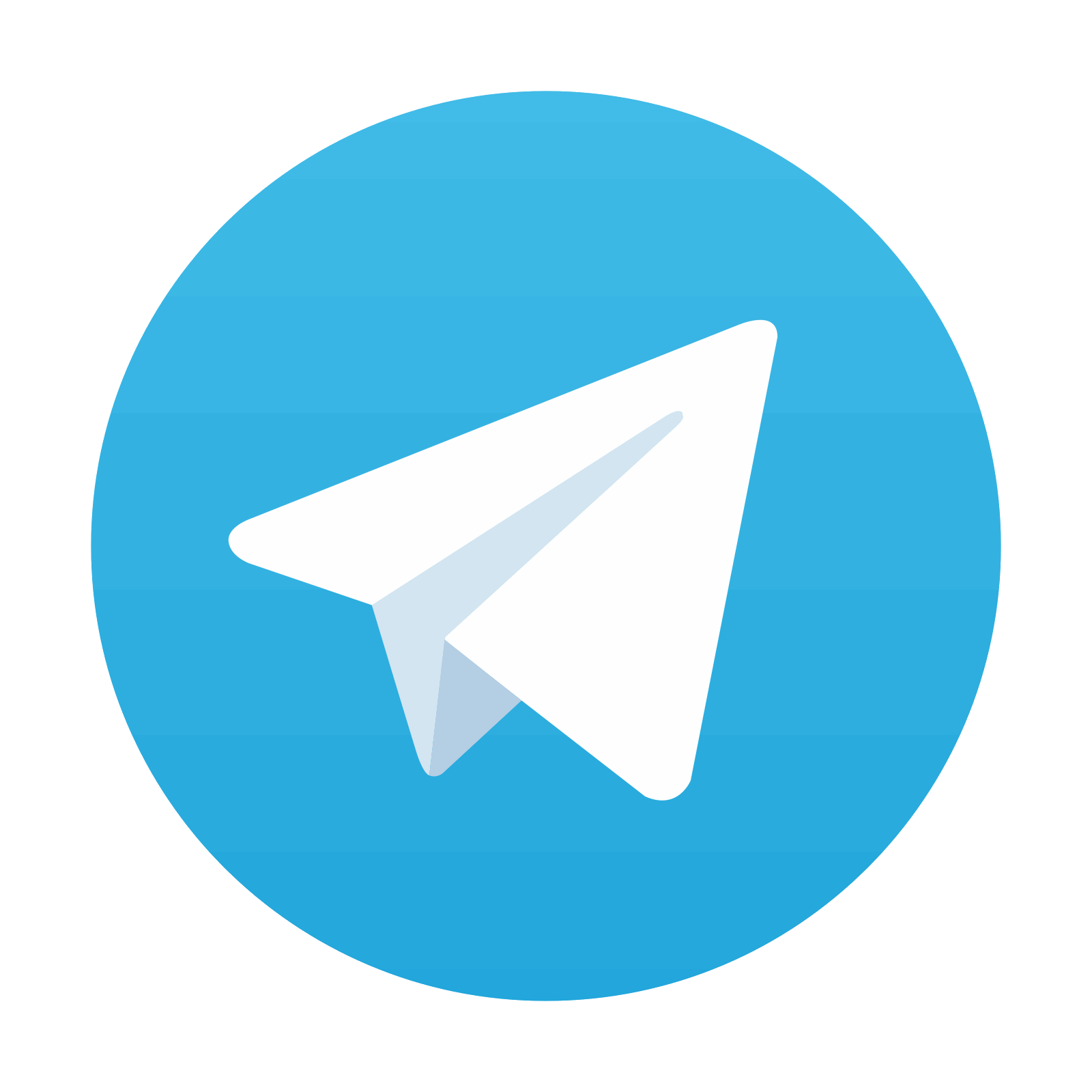
Stay updated, free articles. Join our Telegram channel

Full access? Get Clinical Tree
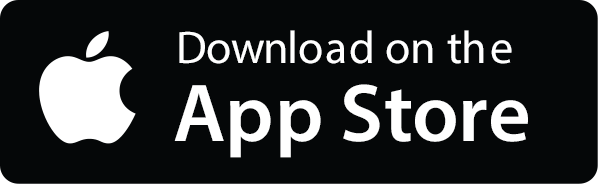
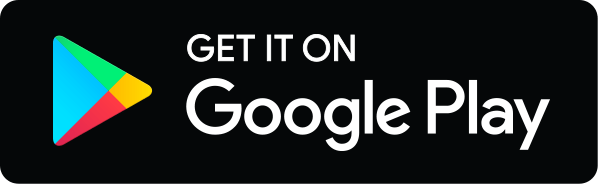