4.1 Introduction
In a world governed by natural selection it is inevitable that there will be competition for resources. The available resources include all living organisms, as a source of food or as hosts for reproduction, distribution, shelter or other reasons. In several cases (e.g. infections with pathogenic Staphylococcus and Streptococcus), these bacteria are more like saprophytes. The molecules that they release and that cause tissue damage – we call them toxins – are used to kills cells and digest tissues in order to provide food for the bacteria. It is in the interests of hosts to defend themselves against those who would use them as resources. In most cases it is also in the interests of the users not to wipe out their hosts for then they would then lose their resources. It is thus not surprising that probably all living organisms have evolved mechanisms to defend themselves against the attentions of invaders.
Innate immunity is the only form of immunity available to the vast majority of organisms and in these organisms it provides effective defence against infection. Innate immunity appears very early in evolution and many of its components can be traced back to the most primitive organisms, with new types of components being progressively added or modified during evolution. As most of the components of innate immunity are pre-formed, defence mechanisms can be activated very quickly after infection. In higher vertebrates, innate immunity can provide rapid defence against some types of infection, although these species have come to rely on adaptive immunity for maximal protection. Innate immunity is, however, crucial for the activation and regulation of adaptive immunity, and adaptive immunity, in turn, utilizes innate mechanisms as effector mechanisms for the elimination of microbes.
In this chapter we examine the different components of innate immunity and their contributions to host defence (Section 4.2). We start by considering the recognition of different types of infectious agents by innate immune cells and the ways in which these cells can direct the responses most appropriate for their elimination. This includes the secretion of specialized molecules (cytokines) that enable communication between different cells and which act to modify their functions. We then discuss the specialized cells resident in all tissues that function to detect infection and trigger inflammation (Section 4.3). This enables recruitment of the effector cells and molecules that are needed for elimination of the microbe. We explain how these recruited cells and molecules provide defence against different types of infectious agent, using studies of human defects or genetically modified mice as evidence for their importance, and how they can help to trigger adaptive immunity (Section 4.4).
In the penultimate section we discuss the origins of the cells of immunity: where they come from and how their production is regulated (Section 4.5). Finally, we show how our knowledge of innate immunity is beginning to inform the design of more effective vaccines, in particular by using components of infectious agents or other biomolecules and even inorganic molecules as adjuvants to boost immune responses against pathogens (Section 4.6).
4.2 Induction of Innate Immunity
4.2.1 The Concepts: Pattern Recognition and Danger
A pivotal moment in the development of immunology, and in our thinking about immunity, came about some two decades ago when it was postulated that the detection of infection might be mediated by receptors of the innate immune system, rather than by the antigen receptors of T cells and B cells. It was suggested that, following pathogen sensing, the innate immune system instructed the adaptive immune system to respond if the antigen was of microbial origin. At the time these innate receptors were unidentified but, for a variety of reasons, it was suggested that they needed to be germline-encoded and that they would recognize conserved features of microbes termed pathogen-associated molecular patterns (PAMPs). The receptors themselves have become known as pattern recognition receptors (PRRs). This concept was put on a firm experimental basis when the germline-encoded Toll-like receptors (TLRs) were discovered. Initially “Toll” was discovered in flies as a molecule involved in development, but it was then found that this receptor also plays a role in defence. TLRs were then discovered in other species, including humans, and we now know that these and other, functionally related, types of receptors have the potential to discriminate between different components of pathogens.
Then, in the early 1990s, the danger hypothesis was put forward. It was suggested that rather than the immune system being concerned with what is self and what is not (self–non-self discrimination), it was actually discriminating between what represents danger and what does not. It was proposed that anything that can cause tissue damage could stimulate an immune response. Pathogens, because of the damage they cause to host tissues, would represent one obvious source of potential danger. However, this hypothesis also implied that other forms of tissue damage (e.g. sterile surgical trauma) might also trigger immune responses. More recently attention has been turning to danger (or damage)-associated molecular patterns; DAMPs. These are produced within or released from damaged cells and tissues, and can be recognized by the innate immune system whether or not they result from microbial infection. The difficulty we currently face in immunology is that while we have an increasing understanding of the PRRs that sense PAMPs, those that might recognize DAMPs are rather more elusive, although we are beginning to gain some insights into their potential nature (Section 4.2.2.4).
Generally speaking, a crucial outcome of recognition of both PAMPs and (so far as we understand them) DAMPs by the innate immune system is the initiation of inflammation. However, there are multiple forms of inflammation that have different functions in homoeostasis in the body (Section 3.3.2). The inflammation caused by sterile injury, for example, may be different, with different functions, from that caused by microbial infection. In the first case, it may primarily enable repair and healing of the damaged tissues, while in the latter it may principally enable the recruitment of soluble and cellular effectors that can help to eliminate the infectious agent (and contribute to healing, later). We ourselves subscribe to the view that innate immunity is indeed concerned with the recognition of danger – in the most general sense – and find it convenient to describe the outcome of recognition as being the production of alarm signals that for example trigger the different forms of inflammation.
4.2.2 Pattern Recognition Receptors (PRRs)
PRRs are now generally thought of as receptors that recognize microbially-derived PAMPs. So what are these molecules? In general, they are not proteins; there are few if any generic differences between bacterial, viral and vertebrate proteins, although there are some exceptions (below). However, there are clear generic differences between other classes of macromolecule expressed in vertebrates and pathogens. One of the best characterized PAMPs is lipopolysaccharide (LPS), also known as endotoxin, a complex of lipid and carbohydrate that is present in the cell wall of Gram-negative bacteria. Another is lipoteichoic acid, present in the cell walls of Gram-positive bacteria. These molecules are integral components of these different types of bacteria and are therefore not dispensable, microbes cannot easily evolve so that they do not express these molecules. Other PAMPs include bacterial and fungal carbohydrates with external mannose residues, glycolipids, and a few unique proteins such as flagellin, a major structural component of bacterial flagellae. See Figure 4.1.
Figure 4.1 Pathogen-associated molecular patterns. Many microbes express molecules that are not expressed by mammals. These include unique carbohydrate and lipid structures, as well as nucleic acids such as single-stranded (ss) or double-stranded (ds) RNA, or DNA containing CpG motifs and a few proteins. Mammals have evolved PRRs that recognize broadly-shared features of PAMPs. These receptors are encoded in the germline. A few examples of the type of molecule that can be a PAMP are indicated.
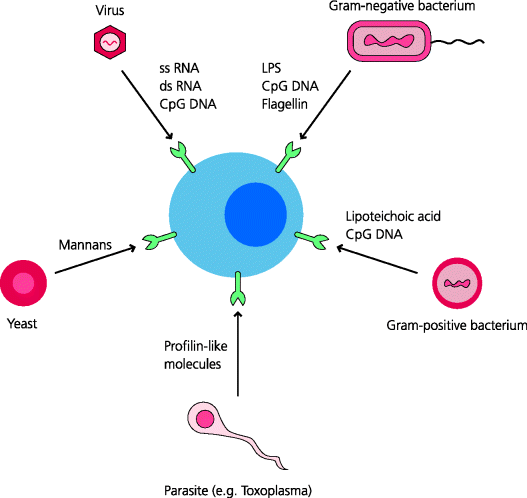
Pathogens can inhabit extracellular tissues, may be internalized by endocytosis or phagocytosis into the endosomal pathway, and some can gain direct access to the cytoplasm during infection of cells. If pathogens are not to escape detection by the immune system, there is a need for sensors at the cell surface and in all of these compartments. These sensors are the PRRs and they exist in a variety of molecular forms. Many are cell-associated, although a number of others are extracellular soluble molecules, which are sometimes termed pattern recognition molecules (PRMs). PRRs are widely expressed throughout the immune system, including the tissue-resident cells of innate immunity that initially sense infection and even by lymphocytes. See Figure 4.2.
Figure 4.2 Cellular locations of pattern recognition receptors. PRRs are located where they are most likely to be able to interact with different types of PAMPs and often where there is little possibility of their meeting host-derived molecules that are cross-reactive with PAMPs. Thus, TLRs may be expressed on the plasma membrane or in endosomes, while other types of PRRs are present within the cytoplasm. Some PRRs can also promote uptake of infectious agents.
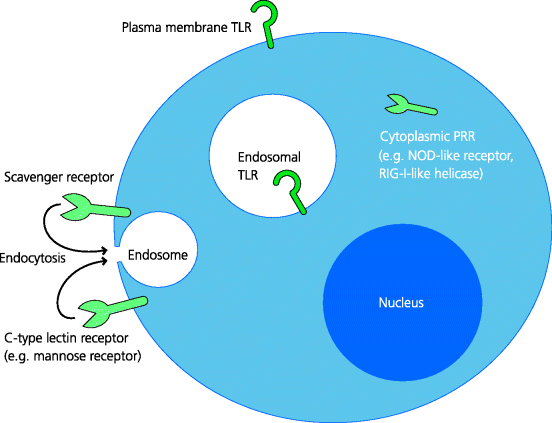
4.2.2.1 Cell-Associated PRRs that Promote Uptake
In the case of phagocytes there are structurally different types of PRR at the cell surface that can also promote internalization of what is recognized, through receptor-mediated endocytosis or phagocytosis. These include some C-type lectin receptors such as the macrophage mannose receptor, which recognizes mannose residues on bacteria. Other members of this family are dectin-1 and dectin-2 that recognize complex carbohydrates (glucans) found in fungi, and which lead to their uptake and also signal to the nucleus to change gene expression (below). Another type of PRR is the family of scavenger receptors, which recognize a wide range of pathogen-associated ligands. One such receptor is CD36, which plays an important role in the recognition, uptake and clearance of apoptotic cells by macrophages for example. Other scavenger receptors also recognize other forms of altered self components such as modified low-density lipoprotein, very important in the pathogenesis of atherosclerosis. Hence the concept of a PRR may need to be extended to include these types of ligands or agonists, as well as those that are microbially derived (below.) See Figure 4.3.
Figure 4.3 Functions of pattern recognition receptors. Most PRRs have signalling properties while some, such as the mannose receptor, are also endocytic receptors promoting uptake (e.g. phagocytosis). Agonist binding to PRRs activates intracellular signalling cascades of different types that modulate cellular functions. Some of their effects can be broadly divided into metabolic, cytoskeletal and changes in gene expression leading, for example, to cytokine secretion. Most of the cytokines secreted are pro-inflammatory.
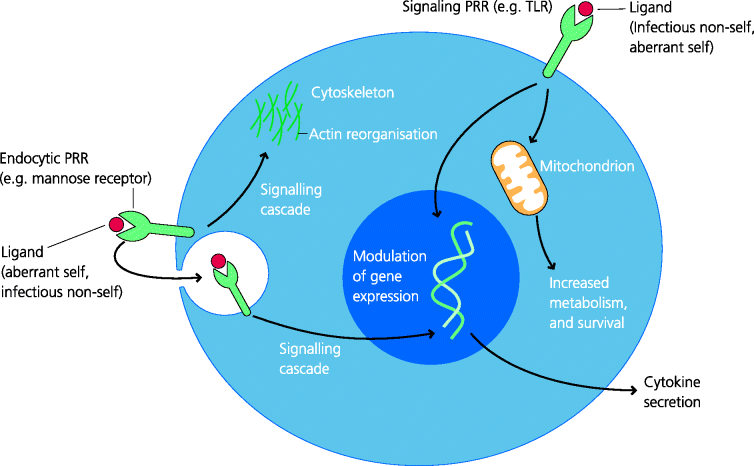
4.2.2.2 Cell-Associated PRRs that Signal to the Nucleus
A large number of other PRRs are critically important to host defence because their ligation induces profound changes in gene expression in the cells the express them; this capacity is crucial for inducible defence. For example, in the case of macrophages, PRR signalling can trigger changes in the expression of hundreds of different genes. These encode molecules such as cytokines and chemokines, anti-microbial peptides, as well as molecules involved in coagulation and tissue repair; we will focus particularly on the former. Some cytokines have direct defence functions, whereas others initiate the local and systemic features of inflammation, and regulate the quality of adaptive immune responses. They include particularly the Type I interferons (IFNs) and important pro-inflammatory cytokines (below).
The signalling PRRs that change gene expression belong to structurally or functionally different families and they have distinct cellular localizations. As indicated earlier, the first set of PRRs to be thoroughly characterized were the TLRs. A subset of TLRs is mainly expressed on the plasma membrane of cells. In general these recognize bacterial components, some acting singly, others acting in pairs. Bacterial components recognized include lipoproteins (TLR2 paired with TLR1 or TLR6), lipoteichoic acid found in the cell wall of Gram-positive bacteria (TLR2 and TLR6) and bacterial flagellin (TLR5). Some surface-expressed TLRs also recognize parasitic molecules; for example TLR11 recognizes another protein, a profilin-like molecule expressed by Toxoplasma gondii. In some cases, TLRs do not directly recognize microbial components although they signal in response to them; for example is TLR4 for which LPS is an agonist (below); TLR4 can also be translocated to endosomes. See Box 4.1
Another set of TLRs is primarily or exclusively expressed in the endosomal system where many viruses and bacteria can be digested after uptake. These TLRs are conventionally viewed as being able to recognize or respond to internalized viral or bacterial nucleic acids, including double-stranded RNA (TLR3), single-stranded RNA (TLR7/8), and viral and bacterial DNA with characteristic features called CpG motifs in which the nucleotides are methylated (TLR9). In fact, it is possible that these particular TLRs may not actually discriminate between self and non-self nucleic acids but instead they may sense these components in an abnormal location. Thus, host cell DNA and RNA is normally confined to the nucleus or cytoplasm; if it is not, there is something wrong.
All viruses and some bacteria can also enter the cytoplasm of cells when they have infected cells. To cope with these, PRRs are expressed within the cytoplasm. However, these are not members of the TLR family. They include a family of so-called Nod-like receptors (NLRs) which recognize components of intracellular bacteria such as muramyl dipeptide, and a family of RIG-I-like helicases (RLHs) that apparently recognize double-stranded RNA produced during viral replication. The former include NOD1, NOD2 and Nalp3 or cryopyrin (Section 4.2.2.4), and the latter RIG-I and MDA5.
It is now absolutely clear that all the PRRs we have noted above can initiate cellular responses to components of infectious agents, PAMPs. There is, however, increasing evidence that some of these receptors may also recognize modified host components. Thus, some TLRs can apparently also recognize host components such as heat shock proteins, fibrinogen, and fragments of heparan sulphate and hyaluronic acid (TLR2, TLR4) which are all associated with signs of stress or damage to the host. If so, the concept of a PRR needs to be extended to include components of both microbial PAMPs and “stressed self” as agonists. It is not yet clear to what extent the latter can also be viewed as representing DAMPs (Section 4.2.2.4).
4.2.2.3 Structure and Function of TLRs
Structures Revealed by Crystallography
All TLRs have a common structural organization with an extracellular recognition domain composed of leucine-rich repeats (LLRs), a transmembrane domain that anchors them to cell surface or endosomal membranes, and an intracellular signalling domain. The latter is called a TIR domain as it is shared by TLRs and the interleukin (IL)-1 receptor. The extracellular domains of TLRs are in the shape of a horseshoe and it appears that many TLRs function as dimers. In some cases, PAMPs (or altered self components) can bind directly to TLRs and therefore they act as true ligands for these receptors. In other cases, the interaction is indirect and involves the ligand binding to other molecules which then interact with the TLR. Binding of ligands or agonists is thought to induce dimer readjustment that can subsequently initiate signalling. Interestingly, it also appears that TLRs can use different molecular mechanisms to bind their respective ligands or agonists (e.g. in the convex or the concave region of the horseshoe-shaped extracellular LRR domain). Moreover, in some cases, the ligand or agonist has to be chaperoned to the TLR by another molecule(s). See Figure 4.4.
Figure 4.4 General structure ofToll-like receptors. TLRs have similar general structures, but the ways in which they interact with their agonists varies greatly. TLRs have a horseshoe-shaped LRR domain connected to a globular TIR domain that is involved in signalling. In some cases, the agonists interact directly with the LRRs. In others cases, one or more accessory molecules may be involved in binding to LLRs and the triggering of signalling can involve several distinct molecular interactions. In general, the activation of signalling requires the dimerization of two TLR molecules.
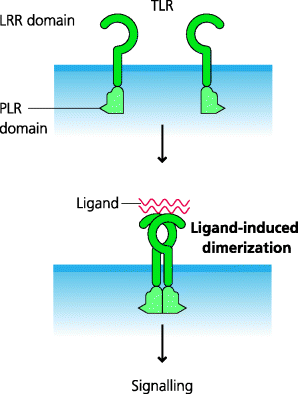
Our increased understanding of the structure and function of TLRs has led to much interest in the development of drugs that inhibit their function for therapeutic purposes. For example, antagonists of TLR4 are being designed as mimetics of the lipid A component of LPS for potential therapy of septic shock in which there is massive production of tumour necrosis factor (TNF)-α as a consequence of ligation of TLR4 on blood monocytes by LPS (Section 4.4.6). Others are being developed as microbially derived adjuvants for use in vaccination (Section 4.6.2).
Signalling via TLRs
What is the outcome of the PRRs recognizing the presence of microbial components? In the case of TLRs we can generalize and say that particularly important outcomes are the secretion of anti-microbial peptides and of pro-inflammatory cytokines that include IL-1, IL-6 and TNF-α (below). Activated TLRs bind adapter molecules which trigger signalling cascades within the cytoplasm of the cells. These result in the activation and nuclear translocation of transcription factors that induce gene expression. For most TLRs the adapter molecule is Myd88. While it might seem that genetically modified mice in which the Myd88 gene is deleted would be informative in terms of dissecting TLR signalling, this adapter is also used by the IL-1 receptor and, in fact, many mouse knock-out phenotypes are actually due to defective signalling through this receptor rather than the TLRs. One of the most important transcription factors for the synthesis of pro-inflammatory cytokines is the nuclear factor NF-κB; another is AP-1, which is induced by triggering of the mitogen-activated protein (MAP) kinase pathways. See Figure 4.5.
Figure 4.5 Signalling pathways from Toll-like receptors. Following agonist binding and dimerization, different adapter molecules bind to TLRs. The key ones are Myd88, which is used by most TLRs, and TRIF, which is only used by one, TLR3; TLR4 can use either depending on its location. The canonical Myd88 pathway leads to NF-κB activation, as well as activation of the MAP kinase pathways which activate the AP-1 transcription factor; typically this results in activation of genes for pro-inflammatory cytokines. In contrast, the TRIF pathway typically leads to activation of IRFs that act on “IFN-responsive elements” in the DNA (not shown) and the activation of genes for Type I IFN production. Cross-talk between these pathways can occur (e.g. stimulation of the Myd88 pathway can also lead to IFN production). Each pathway involves multiple signalling components that are not shown for simplicity.
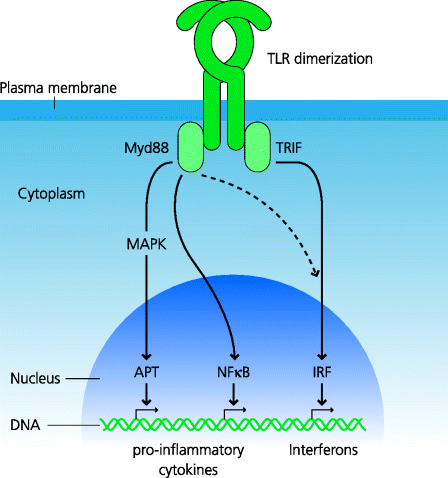
It is very important to appreciate that signalling through TLRs does not trigger the release of a single bolus of cytokines, rather it is a carefully regulated process. For example, different pro-inflammatory cytokines are produced by the responding cells at different times after stimulation and probably with different kinetics in different cell types. If TLR stimulation is sustained, the cells eventually become refractory to TLR stimulation; they stop producing cytokines, presumably to limit the host damage that might be otherwise be caused. In contrast, the capacity to produce anti-microbial peptides usually remains.
TLR3, which binds viral double-stranded RNA, is unusual in that it signals via an adaptor called TRIF instead of Myd88 (and TLR4 can in fact signal via both). Again we can generalize and say that one particularly important outcome of signalling through this pathway is the secretion of Type I IFNs. TLR3 and some RLHs signalling, leads to activation of IFN-regulated factors (IRFs), which are transcription factors that induce the expression of Type I IFN genes. The signalling pathways involved in all these responses are very complex, but understanding how they work is crucially important for the development of therapies (above). It is also very important to stress that while many components of these pathways are shared between different pathways and different cells, there are many that are cell-specific or pathway-restricted See Box 4.2.
Consequences of Defects in TLR Signalling
TLRs are crucial for many aspects of vertebrate host defence against infection. This is reflected by the diversity of different viral components that can interfere with signalling at different points. Viruses can sequester or inhibit the upstream adaptor proteins thus preventing the initiation of TLR signalling. Some examples include vaccinia virus which sequesters multiple adaptors, and hepatitis C virus which uses one of its proteins (ND5A) to bind Myd88 and a protease (NS3-4A) to cleave TRIF. Other viruses can inhibit the downstream transcription factors IRF3 or IRF7 by degrading or sequestering them, or by competing for binding to the promoter sequences of the DNA. Other microbes, such as certain protozoa, can also prevent or reduce TLR signalling.
Figure 4.6 Western blotting. This technique permits identification of specific proteins in a cell lysate. Proteins are dissociated by typically treating with a detergent, sodium dodecylsulfate (SDS). The mix is then electrophoresed on a polyacrylamide gel in which the rate of a protein’s migration when a charge is applied is proportional to its molecular size. This process is called SDS–polyacrylamide gel electrophoresis (PAGE). Antibodies cannot bind to proteins in the gel so typically a nitrocellulose membrane is apposed to the gel, and the proteins are made to migrate into the membrane, again by applying a current; this process is often called “blotting”. Once in the membrane, the proteins can be detected by using antibodies labelled with enzymatic or other labels.
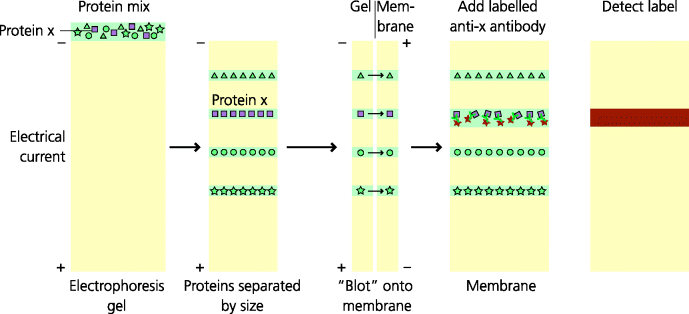
Figure 4.7 Inflammasomes. TLR agonists can lead to the transcription and translation of the IL-1β precursor, pro-IL-1β. Inflammasomes are molecular complexes in the cytoplasm that contain multimerized NLRs, such as Nalp3, associated with a proteolytic enzyme, caspase-1. In activated inflammasomes, caspase-1 processes pro-IL-1 into the mature, functional form of IL-1, which can then be secreted. Hence, particularly in DCs, two stages are needed for production of IL-1 (IL-18 is similarly processed), but how these pathways interact is not well understood; these pathways and requirements may differ in other cell types such as macrophages.
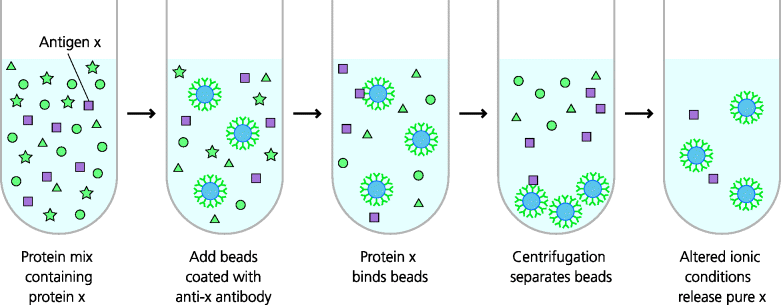
Evidence for the role of TLRs in defence against infection comes from studies of patients with genetic defects in the respective receptors, the adaptors or other signalling components, and from gene knock-out mice in which the corresponding genes have been selectively targeted. Thus, exceedingly rare individuals have been identified with defects in TLR3 or a crucial component in its signalling pathway. These patients present with a herpes simplex virus (HSV)-related encephalitis (inflammation of the brain), thus demonstrating the importance of this pathway, at least in the central nervous system (CNS) in defence against this class of virus. Other individuals with defects in Type I IFN production also suffer from increased viral infections.
Rare patients with genetic defects in Myd88 or IRAK-4 which are involved in TLR signalling have also been identified. These individuals suffered in early life from recurrent pyogenic infections, particularly invasive pneumococcal disease. Surprisingly, however, these infections were not apparent in later life. In contrast, knock-out mice in which the gene for TLR4 was targeted were susceptible to a very wide range of microbial infections for life. (Note, however, the earlier point that IL-1 receptor also signals through Myd88.) This clearly demonstrates that we must be very cautious in extrapolating from studies in mice to the human situation.
4.2.2.4 Inflammasomes and Autoinflammatory Diseases
The original concept of a PRR was that of a receptor for a conserved microbial component, a PAMP. As noted earlier, however, there is increasing evidence that some TLRs may also recognize self components that have become altered or modified through cellular damage or stress, although to what extent this does occur or is relevant in vivo is still not clear. What is, however, clear is that the NLRs can sense both PAMPs such as bacterial peptidoglycans (above) and non-microbial signs of danger including metabolic stress.
Some, possibly all, NLRs – including Nalp3 (also called cryopyrin and by other names) – can act as platforms for the assembly of molecular complexes called inflammasomes which are involved in the activation of specialized proteases – the inflammatory caspases. An example is caspase-1 that processes the precursor form of IL-1β into the active cytokine; the same is also true for a related cytokine IL-18. Thus, a PRR such as a TLR may induce translation of pro-IL-1, but the active molecule cannot be secreted until it is processed by an inflammatory caspase; we still have much to learn about the interactions of these pathways. See Figure 4.8.
Figure 4.8 Immunoprecipitation. This technique is used to isolate specific molecules from a complex mix in solution. Beads coated with antibodies specific for a particular molecule are added to the mix and will bind the specific molecule. Centrifugation can be used to isolate the beads that have bound the molecule; alternatively, magnetic beads can be used and can be captured in a magnetic field. By altering the ionic conditions, the specific molecule can be released from the beads and can be further characterized.
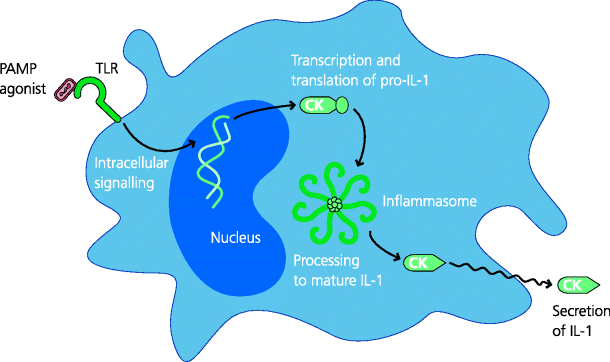
Inflammasomes can be activated by a variety of non-microbial danger signals such as those associated with cell damage perhaps, for example, by sensing abnormal levels of intracellular potassium ions or extracellular ATP, as well as reactive oxygen intermediates (ROIs). Large particles such as monosodium urate crystals, formed from uric acid as an end-product of purine metabolism, as well as silica and asbestos can apparently activate the Nalp3 inflammasome directly or indirectly. Currently, there is much interest in the roles of the Nalp3 inflammasomes in the actions of aluminium-based adjuvants (Section 4.6.1).
IL-1β, produced after inflammasome activation, has multiple biological activities. Crucially, it functions as an endogenous pyrogen in stimulating fever through its actions on the hypothalamus (this term must not be confused with pyogenic, something that induces pus; cf. pyogenic bacteria. For some time it has been known that rare individuals suffer from unexplained and recurrent episodes of fever often accompanied by severe inflammation. These conditions are often familial and known as hereditary periodic fevers. These conditions are examples of autoinflammatory diseases which are known or suspected to result from mutations in components of inflammasomes such as Nalp3 and other, potentially related, signalling pathways such as the TNF receptor and the IL-1 receptor antagonist. Crucially, most of these mutations result in gain of function, rather than being mutations that lead to deficiencies of the components involved. Mutations in the IL-1 receptor antagonist illustrate the importance of this molecule in regulating inflammation. These diseases involve only innate immunity and the adaptive response does not appear to play any part in their pathology. Although rare, these diseases give important insights into the regulation of inflammatory processes.
4.2.2.5 Cellular Distribution of PRRs
If PRRs are to act as alarm molecules, on which cells should they be expressed? Clearly the closer they are to the sites of infection, the earlier they will be activated. It is thus not surprising that some epithelial cells, such as those in the intestine, express PRRs. The PRRs expressed by epithelial cells are not uptake receptors; epithelial cells have no known defence role in phagocytosing pathogens. Interestingly, in the intestine, the expression of these PRRs is polarized to the basal, rather than luminal, surface of the cells and this presumably enables them to discriminate between infectious microbes that have penetrated the epithelium and the commensals situated above. Stimulation of these receptors results in secretion of pro-inflammatory cytokines into the underlying connective tissues (and of antimicrobial peptides into the lumen).
Connective tissues contain resident cells of innate immunity that also express PRRs – primarily macrophages and mast cells – that are ideally placed to detect infection. Macrophages are phagocytic cells that express high levels of certain C-type lectins and scavenger receptors, and they also express the PRRs associated with signalling to the nucleus. In contrast, mast cells may only express the latter. There is, in addition, another cell type present in connective tissues which expresses PRRs, the DC. These cells are, however, primarily thought of as being concerned with the activation and regulation of adaptive immunity, and we discuss them in Chapter 5. Endothelial cells also express some TLRs, and mice that have been engineered to express TLR4 exclusively on endothelial cells can recruit neutrophils to inflammatory sites as efficiently as normal mice and can clear a peritoneal infection with Gram-negative bacteria at least as effectively as normal mice. This indicates that endothelial cells may sometimes play a very significant, direct role in host defence, in addition to being regulated by other cells in the vicinity.
To summarize, in all tissues there are cells that can respond quickly to the presence of a pathogen, and the end result of these responses is the activation of innate immune effector mechanisms (and usually also adaptive immunity). How the activity of these different cells and signals is coordinated is far from clear. For instance, what is the relative contribution of signals generated by epithelial cells and macrophages to innate immune activation? What is the relative importance of macrophages and mast cells in connective tissues? Do these differ in different types of infection, and so on?
4.2.3 Cytokines in Innate Immunity
Signalling through PRRs such as TLRs alters the properties of the cells expressing the PRR, but crucially also enables these cells to modulate the properties of other cells through their secretion of cytokines. Here, we will discuss the roles of some of these cytokines in host defence and inflammation.
4.2.3.1 Interferons (IFNs) and Anti-Viral Resistance
As we have seen (above), Type I IFNs can be produced when viruses are detected in endosomal or cytoplasmic compartments by different PRRs. This occurs, for example, when phagocytes internalize viruses or when viruses infect cells. Type I IFNs are of two main types, α and β (there are others). Both IFN-α (which is of multiple types, and IFN-β bind to a single dimeric receptor. This receptor signals through the JAK–STAT pathway, common to many cytokine signalling pathways, and additionally signals through an IRF (above). Transcription of IFN-stimulated genes leads to the coordinated transcription of a wide variety of genes that confer anti-viral resistance on the cell. Their actions include degradation of viral RNA, suppression of protein translation, inhibition of viral transcription and trafficking, and RNA editing which effectively scrambles the genomes of retroviruses (APOBEC3G). They also induce the synthesis of RLHs, further enhancing the detection of viral presence. Type I IFNs are different from IFN-γ (also termed Type II IFN) that signals through an entirely different dimeric receptor (IFNG1 and 2, although also linked to the JAK–STAT pathway) and which has relatively weak anti-viral activity, but other crucial functions in host defence such as macrophage activation. See Figure 4.9.
Figure 4.9 Type I interferons. Type I IFNs (e.g. α and β) are potent anti-viral molecules. They are secreted by cells containing viruses or other microbes for example, after recognition of viral nucleic acids by cytoplasmic PRRs. They are also secreted by cells of the innate immune system such as macrophages and plasmacytoid dendritic cells (pDCs) after PRR ligation. Type I IFNs act on other cells through a single plasma membrane receptor to induce synthesis of a variety of proteins that collectively act to inhibit viral replication.
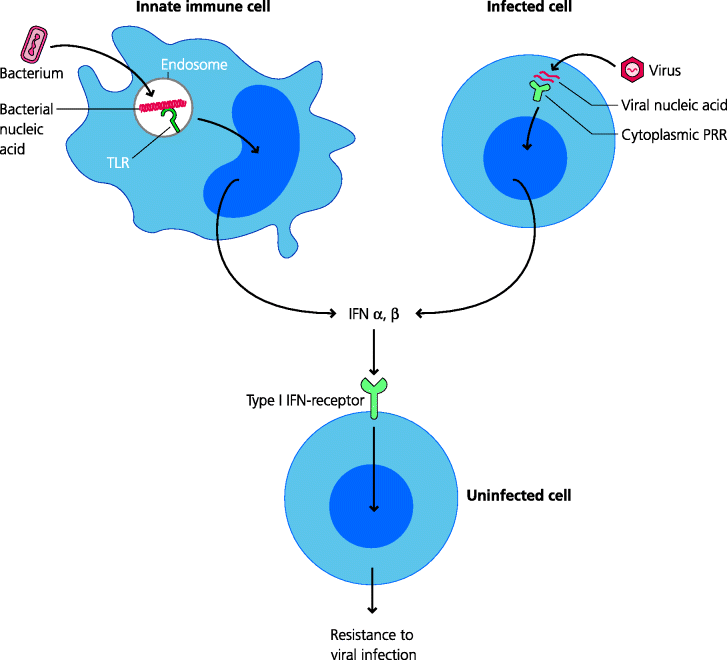
Figure 4.10 Analysis of cytokine secretion I: enzyme-linked immunosorbent assays. Cytokines in solution can be assayed by using ELISAs, as can any other molecule for which antibodies recognizing different epitopes are available. In a multi-stage procedure as illustrated for IL-12, a specific antibody can be attached to a surface to capture the cytokine, and a second antibody to a different epitope on the cytokine can be added to detect the bound cytokine. Binding of the second antibody can be detected by adding a third, enzyme-labelled, anti-Ig antibody. Adding an enzyme substrate leads to production of a coloured reaction product than can be measured quantitatively. By comparing this with a standard preparation of the cytokine, the concentration can be measured. This particular type of ELISA is known as a sandwich ELISA (referring to the two different anti-IL12 antibodies that are used); it is also called an antigen-capture assay.
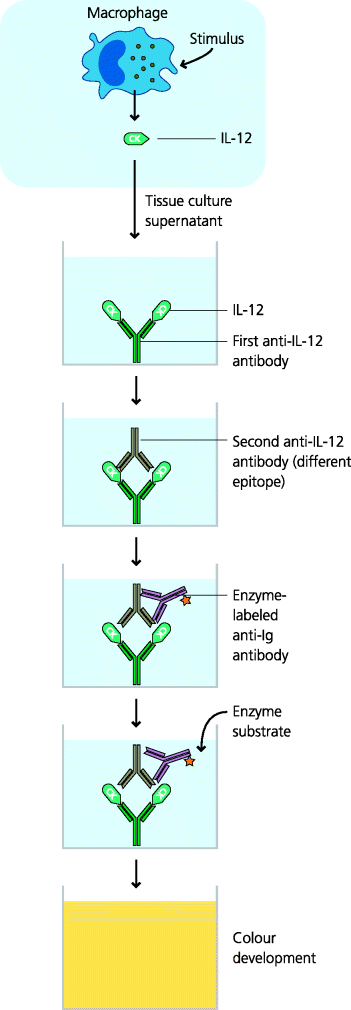
Figure 4.11 Analysis of cytokine secretion II: intracellular flow cytometry. It can be important to know which individual cells in a population are secreting a particular molecule. To determine this, cells in suspension are permeabilized, allowing antibodies to enter. Antibody binding is detected by fluorescent labelling and the cells are analyzed in a flow cytometer. This permits the identification of specific cell types by their expression of particular cell surface molecules (phenotypic markers), and determining whether that cell has synthesized the cytokine in question. This technique is illustrated for a mixed population of macrophages in which only a subset is secreting IL-12; analysis of phenotypic markers, which can be done simultaneously, is not shown.
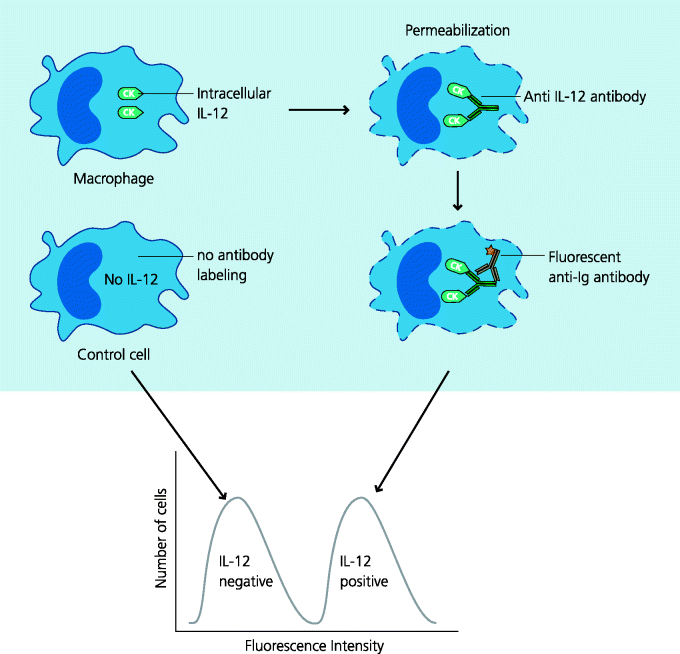
4.2.3.2 Pro-Inflammatory Cytokines
These molecules can be secreted by resident cells such as macrophages, mast cells, and in some cases epithelial cells (e.g. keratinocytes) and even endothelial cells. Their production is largely dependent on the transcription factor NF-κB. As noted earlier, typical cytokines in this group include IL-1, IL-6 and TNF-α.
The IL-1 family of cytokines includes IL-1α and -1β, and IL-18. The cytoplasmic domains of the IL-1 receptor molecules are very similar to those of TLRs and they also act via Myd88. They stimulate a variety of pro-inflammatory responses such as leukotriene and prostaglandin secretion, nitric oxide secretion, and increasd of adhesion molecule expression. They act on the hypothalamus to cause fever and on the liver to stimulate the increase in protein synthesis seen in the acute-phase response. The activity of IL-1 is in part regulated by natural antagonists; the IL-1 receptor antagonist is a “decoy” molecule that is now used therapeutically in a variety of arthritic conditions.
IL-6 signals through the JAK–STAT pathway. This cytokine has multiple effects on inflammation, immune responses, the bone marrow and the nervous and endocrine systems. The use of IL-6 gene knock-out mice has demonstrated its central roles in stimulation of the acute-phase response, induction of fever and mucosal IgA synthesis. Two other cytokines, IL-12 and IL-23, are closely related to IL-6 and also signal through the JAK–STAT pathway. As we shall see (below), IL-12 is probably crucial for acute responses against intracellular pathogens, because it can trigger the production of IFN-γ by natural killer (NK) cells, leading to macrophage activation. In contrast, IL-23 may play a role in the induction of chronic inflammation and the formation of granulomas.
TNF-α was initially discovered in two separate ways: as a molecule that could stimulate the destruction of some tumours in mice, and as the molecule causing the wasting syndrome (cachexia) seen in chronic infections and late-stage malignant tumours. Neither of these relate to the important roles of TNF-α in defence against infection. TNF-α is produced primarily by macrophages and mast cells, but many other cell types can be stimulated to secrete TNF-α. TNF-α signals through two TNF receptors. Such signalling can lead to activation of the cell, with cytokine secretion, or in some cases to apoptosis of the cell (as is seen with some tumours). At low concentrations TNF-α acts locally to increase expression of adhesion molecules on leukocytes, and to stimulate a variety of cell types to secrete pro-inflammatory molecules. At high concentrations TNF-α enters the blood and stimulates many cell types to make excess IL-1 and IL-6. It also stimulates endothelial cells to synthesize nitric oxide, causing venous dilatation. Together these effects can induce potentially fatal cardiovascular shock. One of the main agents inducing TNF secretion is LPS from Gram-negative bacteria. The importance of TNF-α in defence against intracellular bacteria is illustrated by rheumatoid arthritis patients treated with an anti-TNF monoclonal antibody – this can lead to re-emergence of active tuberculosis.
4.2.3.3 Anti-Inflammatory Cytokines
Inflammatory responses are central to defence against infection but can, and do, cause tissue damage. It is thus crucial that inflammatory responses are regulated to minimize such damage. One of the most important ways in which inflammation is regulated is via the secretion of counter-acting, anti-inflammatory cytokines. These include the following examples:
- IL-10. This cytokine is secreted by many cell types including macrophages (particularly alternatively activated macrophages) and Th2 T cells in mice (in humans both Th1 and Th2 cells secrete IL-10). IL-10 acts on macrophages, neutrophils and other leukocytes, largely by inhibiting the actions of NF-κB and thus preventing the synthesis of pro-inflammatory cytokines and preventing biasing of CD4 T cell activation to Th1; in fact, it promotes Th2 biasing. The importance of IL-10 in regulating inflammation is illustrated by IL-10 knock-out mice, which develop a severe inflammatory bowel disease.
- Transforming growth factor (TGF)-β. TGF-β can be secreted by many haematopoietic cells, such as macrophages, lymphocytes and NK cells, but also by some other cells present in the CNS and kidney, for example. TGF-β acts via a receptor expressed on many cell types. Downstream signalling involves interactions with a family of transcription factors called Smads. TGF-β inhibits macrophage activation, cytokine synthesis by macrophages and lymphocytes, and can block the effects of pro-inflammatory cytokines on endothelial cells and neutrophils. It also stimulates angiogenesis and activates fibroblasts, thus having an important role in the induction of healing and repair.
4.3 Tissue-Resident Cells of Innate Immunity
In peripheral tissues, both resident immune cells, and component cells of the tissues, are crucial for innate defence against infection. See Figure 4.12.
Figure 4.12 Tissue-resident cells of innate immunity. Tissue-resident cells can detect the presence of a microbe or its products. Many cells in peripheral tissues such as macrophages and mast cells in connective tissues, and epithelial cells in mucosal tissues, express PRRs that recognize PAMPs from infectious agents. PRR agonists trigger different cellular responses, often including cytokine secretion that triggers inflammation and initiates innate immune responses. DCs, responding to the PAMPs or the signals delivered by other resident cells, become activated and start to trigger adaptive immune responses. Other tissue-resident cells, such as fibroblasts, are involved in repair of tissues damaged by the microbe.
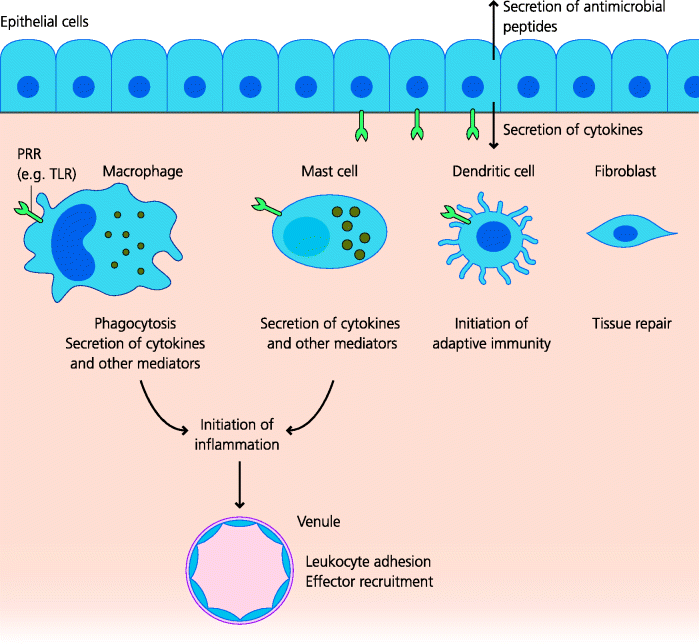
4.3.1 Epithelial Cells
How does activation of the alarm system lead to the generation of effector mechanisms that can kill or limit the growth and spread of an infectious agent? Some effects are very direct. For example activation of epithelial cells stimulates the release of small proteins with direct anti-microbial effects; these include the defensins and other families of molecules. Many of these molecules appear to work by inserting pores into the microbial surface, causing osmotic lysis. It has proved difficult to define the role of defensins in immunity because there are so many of them that their genes cannot all be knocked out in transgenic mice. One example is provided by Paneth cells in the crypts of the small intestine that synthesize defensins following TLR stimulation. To become microbicidal these defensins need to be activated by an enzyme called matrilysin and, if the gene encoding this enzyme is knocked out in mice, they become more susceptible to intestinal Salmonella infection. Epithelial cells are not the only cells that make defensins. For example, these molecules are also stored in neutrophils and are released on recognition of agonists (below).
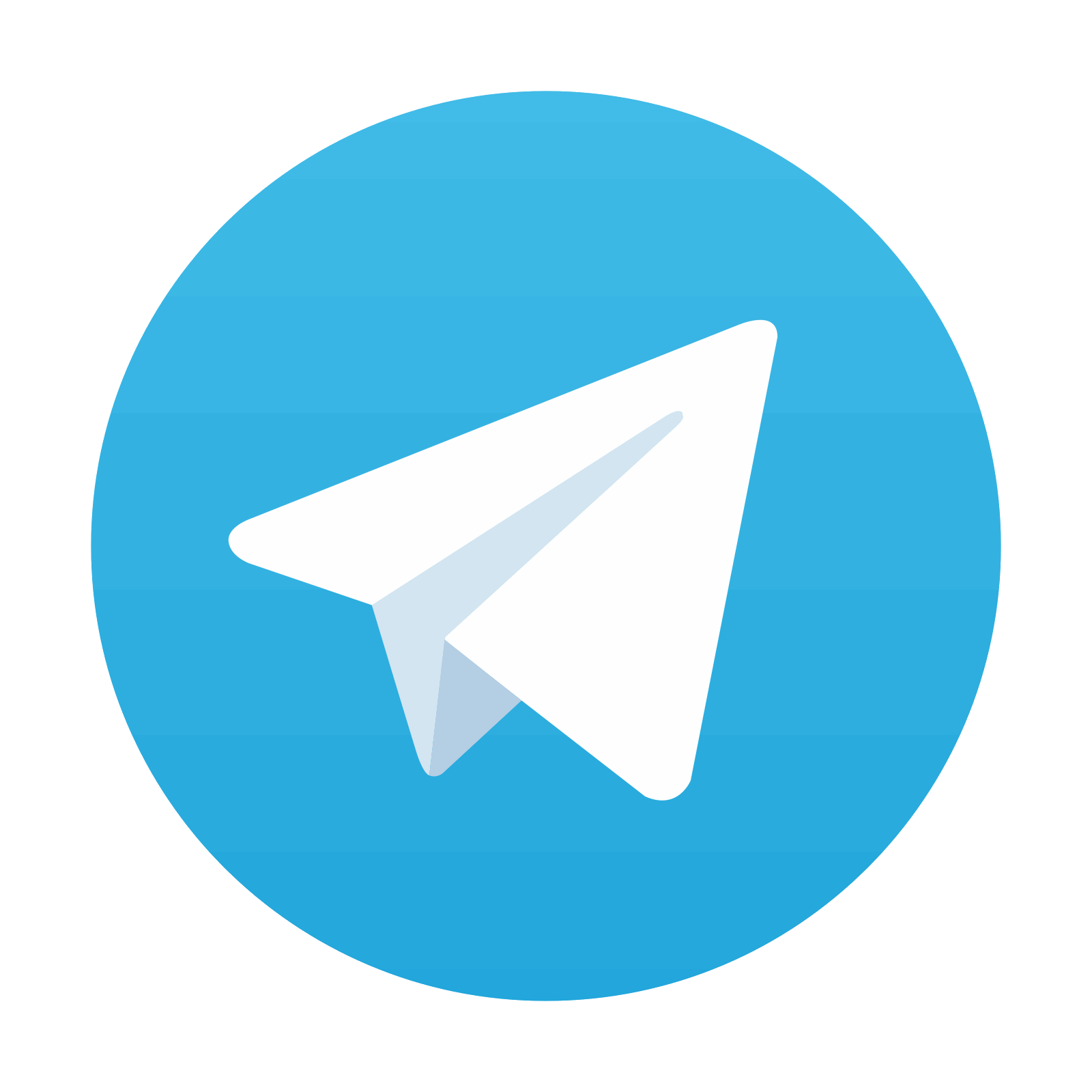
Stay updated, free articles. Join our Telegram channel

Full access? Get Clinical Tree
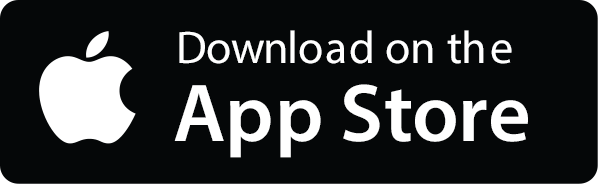
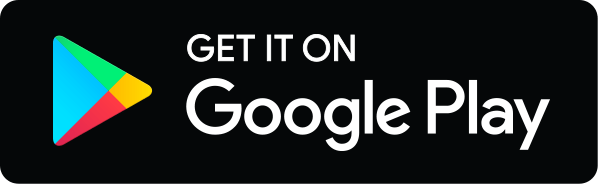