19 Systemic Chemotherapy
The Challenge
The use of systemic chemotherapy for central nervous system (CNS) tumors presents a significant challenge for neurooncologists. These tumors represent a biologically diverse group of diseases with unique growth characteristics and variable response to treatment. Inherent or acquired mechanisms of resistance play an important limiting role in drug development and clinical trial design. Drug delivery is especially problematic, particularly to areas of microscopic invasive disease distant from the main tumor bed. Assessment of the response to treatment is difficult due to the limited ability of imaging to specifically distinguish tumor from areas of injured brain tissue, or to identify microscopic tumor within normal-appearing brain. In addition to these inherent biological, pharmacological, and radiological challenges, the perceived lack of successful treatment in the setting of recurrent malignant glioma often contributes to the nihilistic approach of many physicians caring for these patients, with the result that few patients enter clinical trials.
Despite these problems, however, a concerted effort continues in this field of research that is now largely based on scientific models rather than on the empiricism of the past. Clinical trials serve as the cornerstone of the research effort in neuro-oncology. In recent years several new approaches to trial design have been developed to enhance the traditional model of phase 1, 2, and 3 investigations. New strategies such as the continual reassessment method and “phase 0” testing may optimize early-phase evaluation of dosing and toxicity. Randomized phase 2 trials and adaptive randomization may improve later-phase evaluation of antitumor efficacy. This chapter reviews the principles of traditional clinical trial design and highlights these new approaches.
Progress in the development of effective chemotherapy for CNS tumors has been modest. The use of systemic chemotherapy for CNS tumors first entered routine clinical practice in the late 1960s. Numerous types of cytotoxic chemotherapy agents were used initially, and favored regimens became the standard of care based largely on empiric observation, with some input from prospective trials. In the late 1970s the nitrosoureas became the first cytotoxic chemotherapy agents approved for use in malignant glioma by the United States Food and Drug Administration (FDA). The nitrosoureas remained the most widely used agents in brain tumors until temozolomide was approved by the FDA in 2005 and subsequently became the most commonly used first-line agent. More recently, molecularly targeted agents such as monoclonal antibodies and small molecular tyrosine kinase inhibitors have demonstrated promise in CNS tumors, as in other cancers; however, their role in standard treatment remains to be established. In 2008, the FDA gave accelerated approval status to bevacizumab (a monoclonal antibody) for recurrent glioblastoma based on an uncontrolled randomized phase 2 trial, although final approval is pending subsequent phase 3 trials, and its approval status can be reversed if these trials are not positive. At the time of this writing, it is not clear whether bevacizumab will remain FDA approved.
Aside from bevacizumab, the only approved agents for malignant glioma are the cytotoxic agents (temozolomide and the nitrosoureas). There are no FDA-approved drugs for adult low-grade glioma, but nitrosoureas and temozolomide are frequently used off-label. There is one approved agent (everolimus or RAD-001) for a rare pediatric low-grade glioma called subependymal giant cell astrocytoma (SEGA). There are no approved agents for primitive neuroectodermal tumors (PNETs) or medulloblastomas, although by consensus and based on phase 3 studies, children with newly diagnosed PNET and high-grade glioma typically receive adjuvant chemotherapy, most commonly using multiple agents with or without nitrosoureas or temozolomide. Chemotherapy is also frequently used in ependymoma, although in general it has not proven to be effective. This chapter highlights major clinical issues surrounding the use of cytotoxic chemotherapy in CNS tumors and briefly describes the types of commonly used drugs, including the FDA-approved agents (Table 19.1) and several other classes that are frequently used in the “off-label” setting. A description of newer molecularly targeted agents (monoclonal antibody and small molecular tyrosine kinase inhibitors) is presented in Chapter 22; these agents are not discussed here.
Goals and Structure of Clinical Trials
One goal of clinical trials is to measure response to therapy. Response may be an assessment of drug toxicity or some direct or surrogate measure of antitumor efficacy. Phase 1 studies are done to establish a maximum tolerable drug dose as well as describe pharmacokinetic aspects of the drug in question. A specific goal is to establish a favorable starting dose for later phase 2 trials based on the maximum toxicity and pharmacokinetics of the drug. Thus these studies emphasize toxicity as an important treatment end point. In most cases, patients enter into these trials having previously failed standard treatment regimens or prior phase 2 or 3 studies.
Table 19.1 Chemotherapy Agents Approved by the U.S. Food and Drug Administration for Central Nervous System Tumors
Drug | Class | Indication |
Lomustine | Nitrosoureas | • 1970s: newly diagnosed GBM and recurrent glioma |
Polifeprosan 20 with carmustine implant | Nitrosoureas | • 1997: newly diagnosed GBM and recurrent glioma |
Temozolomide | Methylating agent | • 1999: recurrent grade 3 astrocytoma • 2005: newly diagnosed GBM |
Bevacizumab | Monoclonal Ab to VEGF | • 2008: recurrent GBM |
Everolimus | mTOR inhibitor | • 2010: subependymal giant cell astrocytoma |
Abbreviations: GBM, glioblastoma multiforme; mTOR, mammalian target of rapamycin; VEGF, vascular endothelial growth factor.
Pearl
• Phase 1 trials that test cytostatic or biological therapies with minimal or no expected toxicity may require the use of surrogate biological or pharmacological end points.
Because a treatment benefit is not the primary goal of these early phase 1 studies, they may present an ethical challenge to the physician treating the patient and a difficult dilemma for the patients and their families. Often these patients are desperate for any treatment and may not recognize the true intent of the study and the risks associated with drug escalation schemas. Patients who enroll early in the studies will probably have little chance of any potential therapeutic benefit because of the very low initial starting doses. Traditional phase 1 studies enroll small numbers of patients into each dose level in an attempt to minimize the number of patients exposed to either too low or too high a dose of the drug. As a consequence, these studies are at best an estimate of expected toxicity or therapeutic benefit.
Novel phase 1 trial strategies are being developed, such as continual reassessment method (CRM), that may allow even smaller numbers of patients to be enrolled in the lower drug dose levels and larger numbers in the likely phase 2 drug dose level. Newer agents that do not generate the traditional cytotoxic end points of myelosuppression or end-organ damage, such as cytostatic or biological agents, pose a challenge in phase 1 design and determination of study end points. Several new agents have been in phase 1 testing for long periods of time because either toxicity does not occur despite a very high drug dose, or anticipated biological end points are not reached. In these settings, the phase 2 dose is estimated based on preclinical models. Pharmacological or biologically predetermined surrogate end points are being developed to address this problem. In a new model of early-phase testing called “phase 0,” an extremely low dose of an investigational drug is administered purely for the purpose of determining a pharmacodynamic end point, including specific interaction with a known molecular target such as a tumor-specific cell surface receptor.1 Evaluation of therapeutic efficacy or toxicity in the traditional sense is not the goal of these phase 0 studies. Few patients are needed, the risks are assumed to be very low, and long-term exposure to the agent is not typically planned so that subsequent treatment with other agents can be used in a short time interval.
Phase 2 studies apply the dosing information gathered from phase 1 trials and attempt to measure response to the agent in a larger group of patients. Ideally, patients who enter into these trials will be homogeneous relative to important prognostic clinical factors such as age, performance status, and tumor histology. Unfortunately, many phase 2 trials treat small sample sizes of only 30 to 50 patients, and response is estimated based on a best- or worst-case response scenario without tight confidence intervals around the observed end point. In some studies a drug is deemed worthy of further phase 2 or 3 testing if an “observed” response rate of 20 to 30% is seen. The “true” response rate may be greater or less than the observed response rate because of the limited number of patients available for assessment of response. In addition, patient selection factors may significantly influence this early look at observed response. Younger, healthier patients with good performance status are often overrepresented in these trials because of investigator or patient bias.
When larger cohort, less-selective patient entry occurs in later phase 2 or 3 trials, the “true” response rate will often be significantly less than that observed in early pilot phase 2 testing. One way to mitigate this bias is to conduct a randomized phase 2 trial comparing patients treated with the investigational agent to a matched control group treated concurrently with the standard of care. The sample size needed for a randomized phase 2 trial is smaller than that needed for a more definitive phase 3 study and inadequately powered to prove superior efficacy. Thus, although there is a strong tendency to assume the best arm in these smaller phase 2 trials should become the new standard of care, a subsequent phase 3 trial still must be conducted. Another new strategy for phase 2 trials called adaptive randomization uses data gathered from patients treated early in the trial to inform decision making for subsequent patients as the trial proceeds. With this iterative approach, several hypotheses can be tested within the context of a single study, using multiple agents or combinations of drugs. As with the continual reassessment method for earlier phase studies, adaptive randomization requires realtime statistical analysis as the trial proceeds, rather than after the trial ends.
Controversy
• A treatment benefit is not the specific goal of phase 1 research trials and may pose an ethical challenge for physicians who conduct these studies.
• An intention-to-treat analysis should always be done in the setting of phase 3 trials.
If an investigational agent shows promise in phase 2 trials, a phase 3 study is often considered to be the definitive next step for drug development. The hallmark of phase 3 trials is randomized comparison of the new agent with the standard treatment. Where a standard does not exist, a placebo-controlled, double-blind study is preferable to an open-label study. Randomization must successfully balance patient selection factors in both arms of a phase 3 trial in order to minimize bias that may influence the final results. Intention-to-treat analysis is critical because patients will often opt out of standard treatment or observation-only arms following randomization, particularly if the standard therapy is perceived as inadequate and early phase 2 data are published demonstrating promise in the new agent. Intention-to-treat analysis avoids the misleading effects of patient dropout and crossover by analyzing results based on initial treatment assignment rather than treatment actually received.
Assessment of Response to Chemotherapeutic Drugs
The major goal of phase 2 and phase 3 clinical trials using chemotherapy is to measure the antitumor response of a treatment. The end point in phase 3 studies is typically survival time, whereas antitumor response in phase 2 is usually assessed using magnetic resonance imaging (MRI). Other end points for response could include progression-free survival, duration of objective response, median time to tumor progression, improvement in patient-reported symptoms, overall change in quality of life, or pharmacodynamic end points such as modulation of a known tumor-specific molecular target. Correlation between these surrogate end points and overall survival requires confirmation in subsequent phase 3 trials.
Objective assessment of tumor response is difficult because the actual extent of a tumor is not always clear on MRI, either in the setting of newly diagnosed disease or in disease subjected to treatment. This is particularly true following high-dose radiation therapy such as interstitial brachytherapy or radiosurgery. It may also be true following various forms of systemic high-dose chemotherapy or surgically implanted polymer-based chemotherapy placed directly into surgically created cavities or directly into tumor. Contrast-enhanced T1 and the various T2 MRI sequences are only indirect measures of tumor location or overall tumor burden and are neither specific nor sensitive enough for the investigator to be 100% confident that a positive biological change seen after treatment is caused by that treatment. Fortunately, most MRI measures of assessment are at least adequate enough to provide reasonable assurance that the extremes of a very bad or very good response to investigational treatment are measurable.2 Newer MRI sequences or nuclear imaging may overcome some of the difficulties surrounding standard MRI sequencebased assessment, but until they are validated, standard MRI is the imaging of choice for assessment of response. Section II of this book describes these newer imaging modalities as they relate to end point determination, as well as new consensus approaches to assessment of response and progression.
Currently, response to treatment is often arbitrarily defined by the degree of reduction in tumor volume, with 50% or greater reduction usually signifying response. However, limiting the definition of response to large changes in tumor volume risks deeming a chemotherapy drug inadequate or not worthy of further investigation when in fact it has antitumor activity. Alternative measures of treatment success or failure that depend somewhat less on imaging nuances include time-dependent patient variables, such as the interval of clinically stable disease or overall survival. Death, defined as disease-specific or treatment-related, is the least controversial measure of a treatment effect. Progression-free survival is more problematic because a robust clinical and imaging methodology is required to determine when tumor progression occurs. The diagnosis of progression may be biased simply by the interval of MRI scanning as well as the specific definition of clinical and radiographic progression. Some clinical trials require a certain volume change to declare treatment failure, yet few malignant gliomas are well-circumscribed lesions that are easily measured, even with sophisticated volumetric software systems. Until specific, reliable neuroimaging tools are verified, the assessment of response to treatment will have some degree of subjectivity.
Pitfall
• Chemotherapy response using MRI is difficult to assess in a consistent and reliable manner.
• Anticonvulsant drugs used for seizure control frequently alter chemotherapy drug metabolism and have a potential impact on the assessment of toxicity and response of many of these agents.
A final caveat in clinical trials of systemic chemotherapy is the now well-studied observation that patients with brain tumors demonstrate unique drug metabolism, primarily due to the concurrent use of steroids and anticonvulsant medications. These drugs may alter hepatic metabolism through various pathways, including via cytochrome P-450, such that standard dosing based on experience in other cancers will often be inadequate. Several phase 2 studies in malignant glioma have had to revert to a phase 1 study design because the expected dose based on results in other cancers did not produce significant or anticipated toxicity in patients with malignant glioma. For example, phase 2 studies of irinotecan were conducted in patients with malignant glioma using the phase 2 dose established in colon cancer patients, and minimal activity and toxicity were noted because the standard use of anticonvulsant medications altered drug metabolism. A subsequent phase 1 trial determined that the recommended phase 2 dose for patients with glioma was more than twice the dose expected for colon cancer patients.3
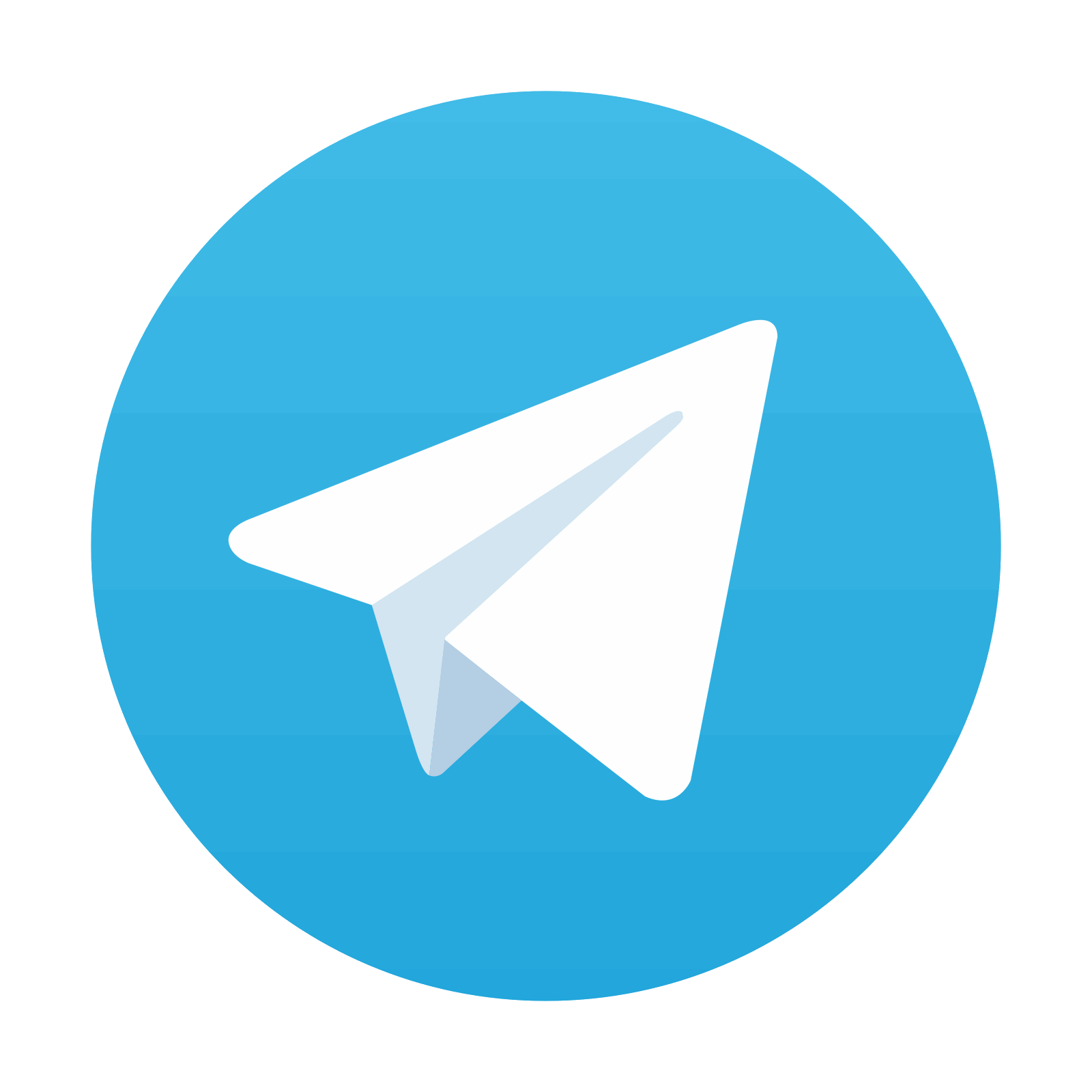
Stay updated, free articles. Join our Telegram channel

Full access? Get Clinical Tree
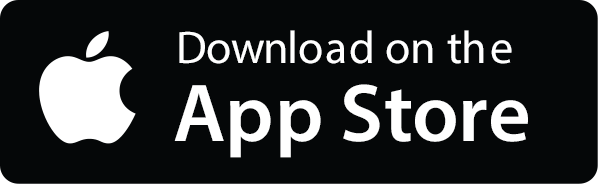
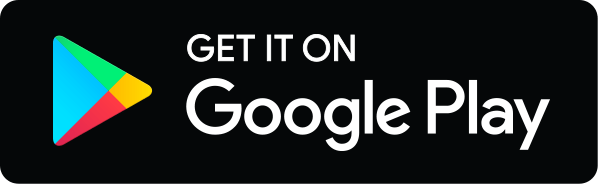