17 Stereotactic Radiosurgery
Over the past 25 years, stereotactic radiosurgery (SRS) has changed the management of most brain tumors. Through precise, conformal delivery of ionizing radiation to an imaging-defined target volume in a single procedure, the biological effect of radiosurgery can lead to cessation or inhibition of tumor cell division, cause neoplastic blood vessels to occlude, induce apoptosis or necrosis, and modify the blood–brain barrier around the tumor.1–6
Radiosurgery was conceived to destroy elements of brain circuits for functional disorders. The initial use preceded modern tumor imaging with computed tomography (CT), and thus utilization and acceptance was slow. The second-generation Gamma Knife unit (1975) incorporated a more spherical dose distribution that was better suited to neoplastic or vascular mass lesions. The third generation increased the number of sources and beam diameters, used greatly improved dose planning systems, and integrated CT and magnetic resonance imaging (MRI) for target definition. Later units facilitated cobalt reloading and added robotics. In 2006 the Perfexion® model (Elekta, Stockholm) expanded robotics, enlarged the potential treatment range, and eliminated helmet changes.7,8 Although most published clinical and basic science research stems from studies using Gamma Knife® (Elekta, Stockholm) radiosurgery technique, modified linear accelerators and cyclotrons producing charged particles have been used for radiosurgery, including devices with robotics and image-based target localization (Cyberknife®, Accuray, Sunnyvale, California).9–12
Stereotactic radiosurgery for the management of tumors has been enhanced greatly by the introduction of MRI, which facilitated high-resolution brain tissue imaging and precise target delineation. In the 1980s, an increasing number of patients with benign tumors underwent radiosurgery, and, in the 1990s, treatment evolved to alter the management of malignant neoplasms. Stereotactic radiosurgery offered an alternative treatment modality with advantages over surgical intervention or radiation therapy. Typically radiosurgery has been used for smaller mass lesions. Older teaching noted that the “upper size limit” for radiosurgery was 3 cm in diameter. Although there was never such a specific cutoff, the teaching reflected the concept that larger tumor volumes, above 4 cm in diameter, would necessitate a dose reduction for safety. Such a dose reduction might be ineffective on the tumor. Although the generalization persists that radiosurgery has a lesser role for larger tumors, careful consideration of the clinical scenario including the degree of mass effect, tumor location, and the burden of systemic disease must also be considered. What also changed was the understanding that lower doses could be effective, and that brain location was critical. For example, a tumor located along a dural/skull surface has less circumferential radiation dose falloff into surrounding brain than the same tumor volume completely surrounded by brain.
As computer speed increased, so did the ability of radiosurgery clinicians to efficiently create highly conformal and selective volumetric dose plans for irregularly shaped lesions. The ability to do this is technology dependent. With the Gamma Knife delivery system, multiple isocenters using narrow radiation beams, or multiple delivery angles, are used to create a three-dimensional radiation volume that matches the imaging-defined tumor margin. The steep falloff of radiation into the surrounding normal structures maintains safety as radiation tolerance within the brain is location and volume dependent.
Because many targets are adjacent to critical brain and nerves, conformal radiosurgery is required to maintain low morbidity rates with high tumor control. In instances where a large decrease in dose is necessary to limit normal tissue toxicity, alternative modes of radiation delivery may be necessary. For instance, in proton radiosurgery, dose is delivered with a sharp beam profile and deposited at a determined depth with minimal exit dose, a radiobiological process termed the Bragg peak. What may suffer is the entrance dose, and the ability to create a truly conformal volume. Additionally, fractionated stereotactic radiotherapy (FSR) may be used in this scenario to provide a higher dose per fraction compared to conventional radiotherapy while maintaining some of the potential benefits of normal tissue repair observed with fractionated regimens.13,14 As for any approach, “potential benefits” must be supported by actual clinical outcomes. It is clear that many aspects of radiobiological teaching proved to be unreliable when the real clinical outcomes of brain tumor radiosurgery were realized. Slowly responding tissue could respond to radiosurgery with tumor volume regression. The singlesession radiobiological power of the procedure transcended the estimated radiobiological power of “equivalent” fractionated doses that had never been used in patients, or delivered with imaging-based precision.
Pearl
• The radiobiology of radiosurgery is different from that of fractionated radiation therapy.
Vestibular Schwannoma
The evolution of SRS has impacted the management algorithm of cranial base tumors such as schwannomas, meningiomas, pituitary tumors, craniopharyngiomas, and other lesions. Rather than simply recommending surgical resection, observation, or fractionated radiation therapy, the multidisciplinary team can now offer radiosurgery as primary or in some cases as adjuvant care. Indeed, for some tumors this has become the preferred approach for many clinicians.
The goals of vestibular schwannoma radiosurgery are to prevent further tumor growth, to preserve cochlear and other cranial nerve function, to maintain or to improve the patient’s neurologic status, and to avoid the risks associated with open surgical resection. Long-term results have established radiosurgery as an important minimally invasive alternative to microsurgery. Initially, radiosurgery was attractive to patients who were elderly or medically infirm, but was later offered to patients of all ages eligible for stereotactic frame fixation. We have found that the results have been consistent across age groups.15,16
During the past decade radiosurgery has emerged as an effective alternative to surgical removal of small to moderatesized vestibular schwannomas. Initially, patients had radiosurgery as an alternative to microsurgical resection due to one or more of the following criteria: advanced patient age, poor medical condition for surgery, recurrent or residual tumor after prior surgery, neurofibromatosis type 2, or patient preference.15,17,18 Currently, most patients select radiosurgery because the literature states that it has superior clinical outcomes that established its long-term safety and efficacy. Data from the University of Pittsburgh included the care of 1,500 patients with vestibular schwannomas using Gamma Knife stereotactic radiosurgery. The mean patient age was 57 years (range, 12 to 95). Eight percent had neurofibromatosis.18,19
Symptoms before radiosurgery included hearing loss (92%), balance symptoms or ataxia (51%), tinnitus (43%), or other neurologic deficit (19.5%). Thirty-four percent of our patients had useful hearing, Gardner-Robertson grade I (speech discrimination score ≥ 70%; pure tone average ≤ 30 dB) or grade II (speech discrimination score ≥ 50%; pure tone average ≤ 50 dB).
Since 1992, the average dose prescribed to the tumor margin was 12.5 Gy, with the 50% isodose line being used in 90% of patients. Lower or higher doses can be used depending on cranial nerve function, tumor volume, and clinical history. In the past decade or so, there has been increasing emphasis on dose to the cochlea in order to maximize hearing preservation. Techniques that reduce cochlear dose below 4 Gy appear to have higher rates of useful hearing preservation.20,21 These can include beam blocking, use of the smallest collimators, or perhaps reducing the dose to the most lateral part of the tumor within the auditory canal.
Pearl
• Techniques that reduce cochlear dose below 4 Gy appear to have higher rates of useful hearing preservation.
Special Consideration
• In some patients, there can be transient expansion of the tumor after radiosurgery, which usually can be observed without further treatment.
Long-term follow-up documented a 98% clinical tumor control rate with no requirement for surgical intervention at 5 to 10 years.17 Patients managed from 1992 to 1997 had a similar success rate.15 Between 1987 and 1992 there were significant modifications in the technique of radiosurgery, including a change from CT- to MRI-based planning, improved computer workstations, conformal dose planning, the use of more isocenters of radiation, the use of smaller irradiation beams, and a reduction in the average margin dose to 12 to 13 Gy. Since modification of these techniques beginning in 1991, there has been a significant reduction in the morbidity of radiosurgery.21,22 Currently, the risk for any grade delayed facial nerve dysfunction is below 1%.21–23 Patients with useful hearing before radiosurgery continue to report an approximate 60 to 85% overall rate for maintenance of useful hearing, depending on tumor size.24 For patients with intracanalicular tumors, the rate of hearing preservation is above 80%.22,25 Radiosurgery has been shown to be a cost-effective, low risk, and an effective alternative to microsurgery for vestibular schwannoma patients.
For smaller tumors, it is likely that more patients now receive radiosurgery as primary management. Although there has not been a randomized trial providing level 1 evidence comparing radiosurgery with resection, and one is not likely to be performed, there are now five matched cohort studies. These studies reported on patients with similar-sized tumors, and evaluated clinical, imaging, and quality-of-life outcomes. All these reports consistently showed better results after radiosurgery for most clinical measures, similar results for the preoperative symptoms of tinnitus and imbalance, and similar freedom from tumor progression rates.23,26–28 In some patients, there can be transient expansion of the tumor after irradiation, which usually can be observed without further treatment.29,30 Based on these data, the remaining indications for surgical resection in a patient with a small to moderatesized tumor are brainstem compression causing disabling imbalance, intractable trigeminal neuralgia or headache, hydrocephalus, an unclear diagnosis, or patient choice.
Stereotactic Radiotherapy
Fractionated delivery of ionizing radiation is likely to result in radiobiological processes that are different from radiosurgery particularly from the standpoint of tissue repair. Fractionated stereotactic radiotherapy may or may not allow for a higher dose of conformal radiation to be delivered to the target in contrast to standard external beam techniques. It can allow for some degree of normal tissue sparing due to interfractional repair. Whether this makes a clinical difference remains to be documented. Fractionated regimens have varied from extended 20- to 30-fraction regimens, to 25.0 Gy provided in five fractions, and 21.0 Gy in three fractions to 18.0 Gy in three fractions. The Stanford group has reported their Cyberknife FSR experience with encouraging local control and hearing preservation.31 The use of FSR may prove to be of value in the management of larger, asymptomatic lesions that are in close proximity to the vestibular or trigeminal system. A linear accelerator (LINAC)-based FSR and SRS comparison of 25.0 Gy provided in five fractions versus 12.5 Gy in one fraction demonstrated similar rates of local control but 5-year trigeminal nerve preservation in favor of the fractionated regimen, an outcome highly dependent on tumor volume.32 Additional insight into the role of fractionated radiotherapy was established by a study of outcomes using stereotactic radiotherapy at a total dose of 50.4 or 46.8 Gy. Unfortunately, the hearing preservation results were not as good as those reported with other techniques, and the follow-up was relatively short (in patients with grades I or II hearing, the median follow-up was 65 weeks). At 3 years, the hearing preservation rate was 55 to 60%, and no patient with grade 2 hearing preserved it at the 50.0-Gy dose.33 As dose becomes standardized in FSR and long-term results are obtained, comparisons between the differing delivery systems will be better appreciated. As with all forms of therapeutic radiation delivery, analysis of outcomes helps to refine techniques.
Although other intracranial schwannomas are less common, the value of stereotactic Gamma Knife radiosurgery has been evaluated in patients with facial, trigeminal, oculomotor, and jugular foramen region schwannomas (Figs. 17.1 and 17.2).
Pitfall
• Hearing outcomes after conventionally fractionated radiation therapy do not show improved outcomes when compared with radiosurgery.
Meningioma
Radiosurgery has proven an effective strategy for patients with benign meningiomas. Initially, radiosurgery was only considered for residual or recurrent tumors after prior resection.34 Two decades ago the use of radiosurgery expanded to the care of patients with small to medium-sized tumors, where the risks of resection were high. Meningiomas are especially suitable for radiosurgery because the tumors are usually well demarcated and rarely invade the brain.35 The steep radiation falloff can be directly conformed to the imaging-defined tumor margin while limiting the dose to normal tissue.36 The problems of delayed tumor recurrence after surgery, surgical morbidity, and surgical mortality, especially in the elderly, increasingly led to consideration of radiosurgery as primary tumor management, particularly when a potentially curative grade 1 resection was not feasible.37,38
Pearl
• Meningiomas are especially suitable for radiosurgery because the tumors are usually well demarcated and rarely invade the brain. For benign tumors, the long-term control rate exceeds 90% in most studies.
The University of Pittsburgh’s 25-year experience included 1,500 intracranial meningiomas. In the last comprehensive analysis, 972 patients with 1,045 intracranial meningiomas were evaluated.34 The series included 70% who were women, 49% who had undergone a prior resection, and 5% who received prior fractionated radiation therapy. The mean age was 57 years. Tumor locations included middle fossa (n = 351), posterior fossa (n = 307), convexity (n = 126), anterior fossa (n = 88), parasagittal region (n = 113), and other sites (n = 115). The mean tumor volume was 7.4 mL. Follow-up beyond 5, 7, 10, and 12 years was obtained in 327, 190, 90, and 41 patients, respectively.
The overall control rate for patients who had adjuvant radiosurgery for known World Health Organization (WHO) grade I meningiomas undergoing prior resection was 93%.34 Primary radiosurgery patients (no prior histologic confirmation; n = 482), had a tumor control rate of 97%. The results were poorer for adjuvant radiosurgery used in patients with WHO grades II and III tumors. In those groups tumor control rates of 50% and 17%, respectively, were found. Recent studies indicate that there may be a dose–response relationship for these more aggressive tumors, with better control rates obtained using tumor margin doses above 15 Gy. At 10 years or more, adjuvantly treated grade I tumors were controlled in 91% (n = 53), and primary tumors, 95% (n = 22). No patient developed a radiation-induced tumor. Primarily treated patients had an unchanged or improved neurologic condition in 93%, whereas those with adjuvantly managed tumors were unchanged or improved in 91%. The overall morbidity rate was 7.7%, and symptomatic imaging changes developed in 4%, at an average of 8 months. Such changes were more common in parasagittal or convexity meningiomas. By 1992, a protocol was adopted that restricted the optic apparatus dose to ≤ 8 to 9 Gy using MRI to identify the optic nerve. By doing so, the risk of delayed radiation–related optic neuropathy is minimal. Indeed this dose prescription may have been too conservative, as others have reported visual safety at 9 and 10 Gy (Fig. 17.3).
Pearl
• Although many clinicians aim to deliver < 8 Gy to the optic nerve or chiasm during radiosurgery, < 10 Gy may be a safe dose for most patients.
Stereotactic radiosurgery has changed the way many neurosurgeons and radiation oncologists manage patients with meningiomas. Rather than performing a subtotal resection and “following the patient,” postoperative radiosurgery to reduce the risk of delayed progression is a valuable option.39 This strategy is particularly valuable for patients < 75 years of age. Several longitudinal studies have shown that untreated meningiomas under observation often continue to grow over time. Radiosurgery is also arguably the preferred option for a young patient with a critically located small meningioma. Observation may no longer be the best choice for such patients, especially if they are symptomatic, but strong evidentiary support is lacking.
Atypical or anaplastic/malignant meningiomas (WHO grade II or III) are best managed with complete resection followed by fractionated radiotherapy to 55 to 60 Gy because of their tendency to extend beyond the borders seen on imaging.40 For nodular residual tumors, radiosurgery can be effective. The estimated risk of local recurrence following surgery alone is high particularly for grade III tumors where survival beyond 10 years is uncommon.41 As a result, external beam radiotherapy is often recommended to improve local control. Radiotherapy planning is based on the pre- and postoperative MRI with irradiation of the gross tumor volume (GTV) plus a margin of 1 to 2 cm.42
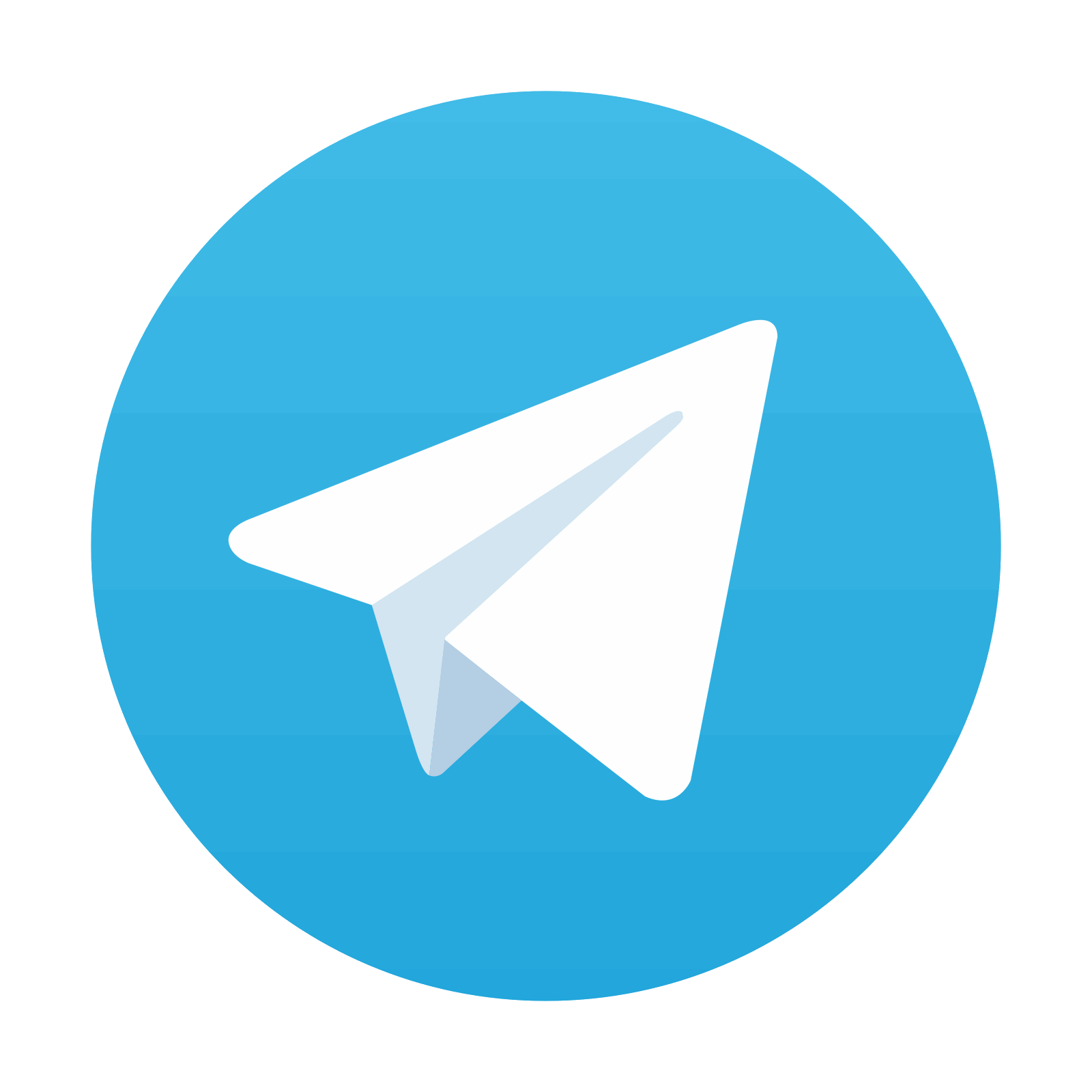
Stay updated, free articles. Join our Telegram channel

Full access? Get Clinical Tree
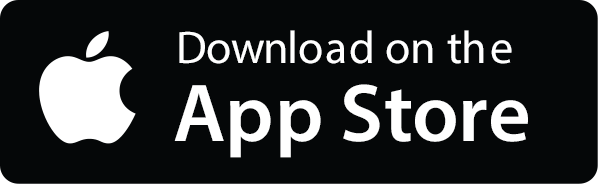
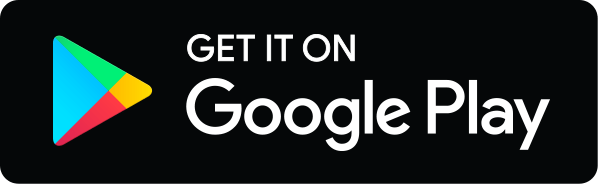