Chapter Outline
Rare bleeding disorders consist of inherited quantitative or functional deficiencies of factors I (fibrinogen), II, V, VII, X, XI, and XIII; combined factor deficiencies, particularly of factors V and VIII; inherited quantitative or functional deficiencies of vitamin K–dependent factors; and disorders of excessive fibrinolysis. Rare bleeding disorders constitute about 3% to 5% of all inherited bleeding disorders. Many of these disorders, which are variable in their bleeding phenotype, may be encountered in the neonatal period and early childhood, whereas others present later in life. Each of these disorders is reviewed in this chapter; however, the reader is referred to reviews or hemostasis texts in the references for a more complete description of these abnormalities.
Fibrinogen Disorders
Fibrinogen is the most abundant clotting factor in human plasma, with normal concentrations ranging between 200 to 450 mg/dL. The hereditary fibrinogen deficiency states (afibrinogenemia and hypofibrinogenemia) and functional abnormalities (dysfibrinogenemias), which account for only 7% of the rare bleeding disorders in the most recent World Federation of Hemophilia annual survey, are among the rarest of the rare bleeding disorders. These disorders should be considered when a prolonged thrombin time (TT) is found in a patient with a history of bleeding who is otherwise essentially healthy. Prolongations of the prothrombin time (PT) and activated partial thromboplastin time (APTT) are found in patients with afibrinogenemia and variably in patients with severe hypofibrinogenemia and some types of dysfibrinogenemia, with PT being more sensitive to a moderate decrement in plasma fibrinogen levels.
Hypofibrinogenemia and Afibrinogenemia
Genetic abnormalities that cause decreased fibrinogen synthesis may occur either as a heterozygous deficiency (hypofibrinogenemia) or as a homozygous deficiency (afibrinogenemia). Clinical findings include ecchymoses, subcutaneous hematomas, hemarthroses, mucosal bleeding, menorrhagia, and postoperative bleeding. Because bleeding severity is strongly associated with fibrinogen activity, patients with fibrinogen activity levels of greater than 100 mg/dL seem to be completely protected from bleeding symptoms, whereas patients with activity levels greater than 70 mg/dL are protected from spontaneous bleeding. Afibrinogenemia presents early in life, frequently in the neonatal period, with gastrointestinal bleeding, hemorrhage from delivery-associated trauma, and bleeding with circumcision. In contrast, patients with hypofibrinogenemia may remain undiagnosed until later in life; their condition may be detected as a result of preoperative screening or after posttraumatic bleeding, except in females, for whom menorrhagia may be the only symptom. Pregnancy complications, particularly spontaneous abortions and placental abruption, have also been reported and are known to occur in laboratory animals that have severe hypofibrinogenemia. Surprisingly, symptoms in patients with afibrinogenemia are not as severe as those seen in persons with the classic hemophilic disorders.
Laboratory evaluation of a patient with afibrinogenemia reveals prolongations of the TT, PT, and APTT (see Chapter 29 ). The best screening tests to detect fibrinogen deficiency are the TT and reptilase time, which measure the time required for conversion of fibrinogen in plasma to a fibrin clot. Reptilase is a thrombinlike enzyme obtained from snake venom. Unlike the TT, the reptilase time is unaffected by heparin treatment. Thus if one encounters a very prolonged TT, the possibility of heparinization must be considered, but marked prolongation of the reptilase time is suggestive of fibrinogen deficiency. Fibrinogen may be detected by functional, precipitation, or immunologic assays. The amount of fibrin clot needed for clot detection is greater with automated clotting instruments than with manual methods. Consequently, these tests are frequently prolonged to the limits of the instrument even though some fibrinogen may be present and a clot may be detected with use of manual methods. However, patients with afibrinogenemia generally have undetectable levels of fibrinogen. Although more than 80 distinct mutations in genes encoding fibrinogen have been identified, genetic testing of patients with afibrinogenemia and hypofibrinogenemia is of no direct value in guiding clinical management.
Because fibrinogen is the ligand for the glycoprotein IIb/IIIa receptor that enables platelet aggregation, both the bleeding time and the results of platelet aggregation tests are usually abnormal. Interestingly, in the absence of fibrinogen, von Willebrand factor is able to bind to the glycoprotein IIb/IIIa complex on platelets and provides a backup mechanism for platelet aggregation, which may result in a reduction in symptoms associated with this disorder. In many patients a small amount of platelet fibrinogen might facilitate some of these interactions, although this fibrinogen is not believed to be derived from synthesis by megakaryocytes; rather, it is believed to be acquired from plasma.
Clinical bleeding episodes in patients with afibrinogenemia, similar to those observed in patients with hemophilia, require episodic replacement therapy. Because of the long plasma half-life of fibrinogen (about 4 days), prophylactic infusions of fibrinogen should be considered in patients with severe symptoms. With the availability of fibrinogen concentrates, fibrinogen replacement therapy has become simpler compared with cryoprecipitate and fresh-frozen plasma (FFP). RiaSTAP is the only fibrinogen concentrate available in the United States. Because it is derived from pooled human plasma, the risk of transmission of bloodborne pathogens is always a possibility, although such transmission is exceedingly rare. Compared with cryoprecipitate, which has a fibrinogen content of about 10 mg/mL, the fibrinogen content of RiaSTAP is more than 20 mg/mL. Hemorrhagic symptoms are usually controlled by initially achieving plasma levels of 80 to 100 mg/dL and achieving maintenance at a level greater than 50 to 60 mg/dL until the bleeding subsides. To raise the fibrinogen level to 100 mg/dL in an afibrinogenemic patient, approximately 0.17 unit/kg of cryoprecipitate and 60 mg/kg of RiaSTAP must be infused. Because of the prolonged half-life of fibrinogen, replacement therapy can be provided at intervals of 3 to 4 days.
Dysfibrinogenemia
Numerous dysfibrinogenemias, which are named after the city in which they were originally identified, have been reported. Most dysfibrinogenemias are associated either with no clinical symptoms or with hemorrhage, whereas others, interestingly, are associated with a predisposition to venous or arterial thrombosis (see Chapter 33 ). In one series more than half of patients experienced no clinical complications, whereas bleeding occurred in 25% and thrombosis occurred in 20%. Functional abnormalities of fibrinogen are generally inherited in an autosomal-dominant manner. Occasionally, however, index cases are associated with consanguinity and major hemorrhagic or thrombotic symptoms. Hereditary dysfibrinogenemia that is associated with hemorrhage usually results in a prolonged TT. Laboratory evaluation for dysfibrinogenemia includes functional and immunologic assays for fibrinogen, which should show a normal level of fibrinogen antigen in the presence of reduced functional fibrinogen. The ratio of functional to antigenic fibrinogen is usually less than 0.5. The reptilase time may also be prolonged. An occasional person with dysfibrinogenemia may have a prolonged PT or APTT. The inability of some abnormal fibrinogen to clot completely in vitro can result in false-positive results in fibrin(ogen) degradation product tests. TT and reptilase time are often prolonged in persons who have dysfibrinogenemia associated with thrombosis, though the TT is substantially shortened in some variants. Molecular testing may be of clinical significance in persons with dysfibrinogenemia because some mutations may be predictive of a bleeding phenotype and other mutations may be predictive of thrombosis, but currently molecular testing is only available through research laboratories. Infusion of fibrinogen in the form of cryoprecipitate may be indicated in patients who have clinical bleeding, with a normal rate of survival of this fibrinogen to be expected. Fibrinogen concentrates are not approved for use in persons with dysfibrinogenemia but may be used off-label in patients with a severe bleeding diathesis.
Severe liver disease is occasionally associated with acquired dysfunction of fibrinogen. Studies usually demonstrate that this disease is not hereditary in nature, and the liver disease is generally overt.
Prothrombin Disorders
Prothrombin, or factor II, is a vitamin K–dependent glycoprotein with a plasma concentration of approximately 100 µg/mL. The hemostatic level of prothrombin is around 20% to 40%. Prothrombin deficiency is exceedingly rare, with a prevalence of about 1 in 1 to 2 million people, but in a few North American series it has been noted to disproportionately affect Latinos. Similar to congenital abnormalities of fibrinogen, defects in prothrombin present either as quantitative (hypoprothrombinemia, or type 1 disorders) or functional deficiency (dysprothrombinemia, or type 2 disorders). Aprothrombinemia is incompatible with life. Hypoprothrombinemia is inherited as autosomal-recessive defects in the prothrombin gene, leading to decreased production of protein. The mutations resulting in dysprothrombinemia either cause defective activation of the prothrombin molecule by factor Xa or result in its altered interaction with fibrinogen. Occasionally prothrombin deficiency can occur in association with combined deficiency of other vitamin K–dependent factors because of mutations in either the γ-glutamyl carboxylase gene or the vitamin K epoxide reductase gene.
Clinical presentation is strongly associated with the level of functional protein. Heterozygotes are usually asymptomatic, although postoperative bleeding has been reported. Clinical bleeding in homozygotes ranges from easy bruising and mucosal bleeding to severe posttraumatic or postoperative bleeding. Hematomas, umbilical cord bleeding, intracranial bleeding, and major hematomas after vaccination have been reported in the neonatal period. Although the APTT may be slightly prolonged, the hallmark of disorders of prothrombin is usually a prolonged PT and a normal TT. In some cases of dysprothrombinemia, such as prothrombin Cardeza, the PT may be nearly normal even though prothrombin activity is markedly reduced, and thus specific prothrombin assays comparing immunologic levels with functional levels are required to diagnose dysprothrombinemias. The lack of deficiencies of other vitamin K–dependent factors (i.e., factors VII, IX, and X) and liver-dependent factors (i.e., factor V and fibrinogen) usually directs attention to the diagnosis of a hereditary deficiency of prothrombin. Immunoassays and assays of electrophoretic mobility help identify dysprothrombinemia. Molecular testing is only available in the research setting and does not provide much clinical utility.
Treatments currently available for prothrombin deficiency include FFP and prothrombin complex concentrates (PCCs). Acute bleeding episodes can be easily treated with FFP. A dose of 15 to 20 mL/kg will usually raise the factor level by about 25%. Given the long half-life of prothrombin (approximately 60 hours), transfusion volume is usually not an issue. Three-factor PCCs contain factors IX, X, and prothrombin and a small amount of factor VII; the amounts vary from product to product and from lot to lot. Four-factor prothrombin factor concentrates contain all four vitamin K–dependent procoagulant factors and the antithrombotic proteins C and S; a description of the actual units of each factor in each vial accompany the product. Four-factor PCCs are indicated for the urgent reversal of acquired coagulation factor deficiency induced by vitamin K antagonist (e.g., warfarin) therapy. These products are labeled and dosed on the basis of factor IX units. These products are not approved for prothrombin deficiency, but a dose of 20 to 30 units/kg will usually be hemostatic. Thrombotic complications resulting from supratherapeutic levels of other factors remain a potential problem. Antifibrinolytic agents are a useful adjunct to factor replacement but are best avoided in combination with PCCs.
Factor V Deficiency
Factor V deficiency, which was first reported by Owren in 1947, has also been termed parahemophilia . Its prevalence has been estimated to be 1 in 1 million people with no ethnic predisposition. It is transmitted autosomally and is generally symptomatic only in the homozygous or compound heterozygous state. Factor V activity has limited correlation with bleeding severity. Mucocutaneous bleeding and hematomas are the most common symptoms, but hemarthroses, gastrointestinal tract bleeding, and central nervous system bleeding are rarely encountered. Menarche is frequently associated with severe menorrhagia. Both the PT and the APTT are prolonged, which usually prompts specific factor assays. Immunoassays for factor V are not readily available, and thus most cases are defined by identification of functional deficiencies of factor V with the use of clotting assays. Liver disease, disseminated intravascular coagulopathy, a factor V inhibitor, and combined factor V and VIII deficiency states must be ruled out before diagnosing factor V deficiency.
Clinical bleeding can be controlled with FFP, which is the only currently available source of factor V. Normal hemostasis is achieved with levels greater than 20%. In a patient with severe factor V deficiency, hemostasis can be achieved with a loading dose of 15 to 20 mL of plasma per kilogram of body weight. The half-life of factor V is 12 to 36 hours, and hence daily infusions of plasma are sufficient. Factor V is more labile in FFP compared with other hemostatic factors, and thus it is important to use FFP that is less than 1 to 2 months old. Mild mucocutaneous bleeding can be treated with antifibrinolytic agents. In rare instances, alloantibodies to factor V can develop in patients with congenital factor V deficiency who are, of course, quite symptomatic, but their condition can generally be managed by infusing fresh platelets that contain normal platelet factor V and account for about 20% of circulating factor V.
Occasionally one may encounter a patient who has an acquired inhibitor to factor V. These patients have usually undergone surgical procedures in which topical bovine thrombin has been used. A mixing study can be performed to confirm the presence of an inhibitor. Even though the PT is markedly prolonged, some of these patients do not have major hemorrhagic symptoms.
Combined Deficiency of Factor V and Factor VIII
More than 50 pedigrees featuring an autosomal-recessive bleeding disorder characterized by a combined deficiency of both factor V and factor VIII have been reported. In some cases the mutations responsible for this disorder are in the “lectin mannose-binding 1” (LMAN1) gene (also known as endoplasmic reticulum–Golgi intermediate compartment protein, or ERGIC-53), which functions as a molecular chaperone from the endoplasmic reticulum to the Golgi apparatus in the biosynthesis of both factor V and factor VIII. In other kindreds, this disorder is associated with mutations in the multiple coagulation factor deficiency 2 (MCFD2) gene. MCFD2 encodes a protein that forms a calcium-dependent stoichiometric complex with LMAN1 and acts as a cofactor in the intracellular trafficking of factors V and VIII. The disease is associated with a moderate bleeding tendency and plasma factor V and factor VIII levels of 5% to 30% of normal. Combined factor V and factor VIII deficiency is usually accompanied by fewer symptoms compared with hemophilia A, but when hemostasis is required, it generally can be achieved with FFP.
Factor X Deficiency
Factor X deficiency is a rare autosomal disorder estimated to have a worldwide incidence of about 1 in 1 million people; it is more prevalent in populations that practice consanguineous marriage, such as in Iran. Factor X is a vitamin K–dependent factor produced by the liver, and activation of factor X by the “tenase” complexes is considered the first step in the common pathway of coagulation. Similar to other coagulation factors, deficiencies of factor X can also be classified as type 1, in which both antigenic and functional activity are proportionally decreased, and type 2, characterized by near-normal antigenic levels but reduced functional activity. A reduced factor X level is identified by a factor X clotting assay. In patients with dysfunctional factor X, the factor X clotting assay is prolonged, but results of the immunoassay are normal. Mucocutaneous bleeding together with posttraumatic bleeding are the most frequent bleeding symptoms. Hemarthrosis and intracranial bleeding are reported in persons with functional levels of less than 1%. The diagnosis is usually suspected when both PT and APTT are prolonged. A factor X functional assay and immunologic assays to measure factor X antigen confirm the diagnosis. Additional assays include the Russell viper venom (RVV) time. RVV is a metalloproteinase that directly activates factor X, and the RVV time will be abnormal in persons with factor X deficiency, although this assay may be normal in persons with some type 2 factor X deficiencies. Factor X levels are low at birth and reach adult levels by about 6 months of age. Factor X levels may also be low in persons with liver disease, accompanied by low levels of factors II, V, VII, and IX.
The biologic half-life of factor X is about 40 hours. Factor X levels of 20 to 30 units/dL are generally associated with the cessation of hemorrhagic symptoms. Infusion of approximately 20 mL of FFP per kilogram of body weight followed by 6 mL/kg every 12 hours increases the level of factor X sufficiently to achieve hemostasis for minor bleeding episodes. Although not approved for the treatment of factor X deficiency, PCC can also be used to treat major hemorrhage (see the previous discussion regarding use of PCCs in persons with prothrombin deficiency); however, their use can potentially be thrombogenic. Although factor X is a vitamin K–dependent factor, vitamin K has no therapeutic effect on factor X in patients with a congenital deficiency. Antifibrinolytic agents can also be adjunctive but are best avoided in combination with PCCs.
Although it is not usually a pediatric problem, amyloidosis may be associated with factor X deficiency because of adsorption of factor X to the amyloid material. Therapeutic infusions are not generally efficacious in patients with acquired deficiency of factor X as a result of amyloidosis.
Factor VII Deficiency
Factor VII deficiency has an estimated incidence of 1 per 500,000 in the general population worldwide. Inherited as an autosomal-recessive disorder, the hemorrhagic predisposition in affected patients is highly variable and correlates poorly with plasma factor VII activity levels. Heterozygous deficiency is not usually associated with hemorrhagic symptoms. Patients with levels that are less than 1% of normal can experience severe bleeding episodes, including hemarthroses and crippling arthropathy, comparable with patients who have the classic hemophilias. Neonates who have factor VII deficiency are at increased risk for the development of intracranial hemorrhage, which is attributable to trauma at the time of delivery.
Hemostasis screening tests in patients with factor VII deficiency reveal a prolonged PT with a normal PTT and a normal TT. Heterozygotes typically have normal PTs and are identified only after specific factor VII assays are performed in family members of patients who have homozygous or compound heterozygous factor VII deficiency. Laboratory evaluation of factor VII deficiency relies on specific assay of plasma factor VII coagulant activity with the use of animal or human thromboplastin, as well as immunologic quantitation of factor VII antigen. Patients have been categorized by their plasma level of factor VII antigen or cross-reacting material (CRM; CRM−, low or absent antigen; CRM R , reduced antigen; CRM+, normal antigen). CRM− defects result from defective factor VII biosynthesis or accelerated clearance in vivo, CRM+ defects are structurally abnormal factor VII molecules, and CRM R defects may result from a combination of these mechanisms. Some CRM R or CRM+ factor VII molecules give variable plasma factor VII coagulant activity results when assayed with tissue factor from different animal species (e.g., rabbit brain, ox brain, simian brain, or human brain). As anticipated, the molecular basis of factor VII deficiency is heterogeneous, and several different types of mutations have been described in patients with factor VII deficiency. Most mutations have been missense mutations, but nonsense mutations, small deletions, splice site abnormalities, and promoter mutations have also been identified.
Factor VII is a vitamin K–dependent factor, but vitamin K administration is of no therapeutic benefit in the treatment of hereditary deficiencies. Although commercial PCCs may contain some factor VII, the amount is highly variable among the different commercial preparations. The factor VII content also varies between different lots produced by a single manufacturer. Because the plasma half-life of factor VII is short (approximately 3 to 6 hours), plasma infusions may not achieve adequate levels for normal hemostasis. Factor VII concentrates have been available for patients with factor VII deficiency in clinical trials or on a compassionate-use basis. The use of activated recombinant factor VII, which has a half-life similar to that of factor VII, has also been shown to provide effective hemostasis in patients with factor VII deficiency and has been approved for this indication in many countries, including the United States. Anecdotally, the therapeutic effect of factor VII infusions appears to be longer than the time during which measurable factor VII levels can be identified in plasma. In one patient treated with PCCs on a regular basis, mucocutaneous bleeding was controlled for 3 to 4 days after a single infusion, even though factor VII was not measurable after 24 hours. It was not clear whether this control of mucocutaneous bleeding resulted from surface binding of factor VII to endothelial cells or platelets, removal of it from plasma, or some nonspecific effect from the concentrate infusion.
Factor XI Deficiency
Factor XI deficiency is usually inherited as an autosomal-recessive disorder. The APTT is prolonged in factor XI deficiency, whereas the PT is normal. A specific assay for factor XI coagulant activity is necessary to make the diagnosis. Factor XI assays are best performed on fresh plasma because the apparent factor XI level may be increased after freezing plasma.
Although factor XI deficiency is most frequently encountered in Jewish persons of Ashkenazi descent, it is occasionally found in other ethnic groups, and the mutations have been characterized. In Israel, 1 to 3 per 1000 Ashkenazi Jews are homozygous or doubly heterozygous for this deficiency, which calculates to a gene frequency of 4.3%. Sephardic Jews, on the other hand, are rarely affected by factor XI deficiency. Three different point mutations in the factor XI gene account for nearly all cases of factor XI deficiency in Ashkenazi Jews: (1) a splice site mutation at the last intron that disrupts normal messenger ribonucleic acid processing (type I), (2) a nonsense mutation in exon 5 resulting in a premature stop codon (type II), and (3) a missense mutation in exon 9 resulting in substitution of Leu for Phe at 238-amino acid. Although the genotype correlates well with plasma factor XI levels, there is not good correlation with the hemorrhagic tendency. In Ashkenazi Jewish patients who are homozygous or doubly heterozygous for factor XI deficiency, the APTT is often longer than it is in patients with either severe factor VIII or factor IX deficiency.
Although factor XI deficiency has been termed hemophilia C , the bleeding tendency is highly variable, and bleeding almost always occurs in association with surgery or trauma. Epistaxis, soft tissue hemorrhage, and bleeding after dental extractions may occur, but hemarthroses and concomitant arthropathy are not seen. Affected women may experience menorrhagia. Even though low factor XI levels are not always associated with bleeding, patients with a significant personal or familial history of bleeding should probably receive FFP before major surgery. In the absence of such a history, prophylaxis with FFP should probably be administered for ear, nose, and throat (e.g., tonsillectomy), neurosurgical, cardiac, or urologic procedures. Patients who have previously undergone major surgery without bleeding or who are having minor procedures that can be controlled easily by local pressure (e.g., dental extraction) may be monitored closely, with initiation of treatment only if hemorrhage occurs. Heterozygous patients with factor XI levels greater than 20% of normal also may occasionally experience bleeding.
Plasma infusions of 1 unit/kg body weight can increase the circulating factor XI concentration by about 1.5 unit/dL. A loading dose of 15 to 20 mL of plasma per kilogram will result in plasma levels of 20 to 30 units/dL, a level that is usually sufficient to control moderate hemorrhage. Additional plasma infusions of 7.5 to 10 mL/kg can be administered at 12- to 24-hour intervals until hemostasis is achieved. The half-life of factor XI is in excess of 48 hours. In factor XI–deficient patients who are deemed to be at risk for bleeding, antifibrinolytic agents such as ε-aminocaproic acid or tranexamic acid can be used to prevent hemorrhage after minor surgical procedures (e.g., dental extractions). A plasma-derived factor XI concentrate is available for use in Israel but not in most other countries. The development of inhibitors to factor XI has been reported in patients with severe factor XI deficiency who have received prior treatments with plasma.
Factor XIII Deficiency
Factor XIII (fibrin-stabilizing factor) is responsible for clot stabilization and cross-linking of the fibrin polymer, protecting it from fibrinolysis, and hence deficiency of factor XIII is characterized by delayed bleeding. In a typical patient who sustains trauma on one day, a bruise or hematoma will develop on the following day. Other symptoms of factor XIII deficiency include delayed umbilical cord hemorrhage, delayed separation of the umbilical stump in the neonatal period (beyond 3.5 to 4 weeks), intracranial hemorrhage with little or no trauma, poor wound healing, and recurrent spontaneous abortions in women. Although factor XIII is a heterodimer of two enzymatic A subunits and two carrier B subunits, most known cases are due to mutations in the A subunit. The deficiency states of factor XIII are also classified as type 1, or antigenic deficiency, and type 2, or dysfunctional protein, with the latter being exceedingly rare.
Laboratory evaluation reveals normal hemostasis screening test results, including bleeding time, APTT, PT, and TT. For a patient with normal screening test results but clinical symptoms of a bleeding disorder, one must perform a factor XIII assay to avoid a misdiagnosis. Screening tests such as clot solubility tests have poor sensitivity but may be the only option in a resource-poor setting.
Recently a heat-treated, lyophilized, plasma-derived factor XIII concentrate, Corifact, has become available. Because factor XIII has a very long half-life of 5 to 7 days and a level of 2 to 3 units/dL is hemostatic, the recommended dose of Corifact is 40 international units/kg every 28 days. When needed, an infusion of 5 to 10 mL/kg of FFP is also effective. Cryoprecipitate may also be used. One bag of cryoprecipitate contains 75 units of factor XIII.
Rare cases of acquired factor XIII deficiency as a result of autoantibody against factor XIII-A has been reported. This deficiency is usually associated with autoimmune conditions, and neutralizing antibodies can be detected with mixing studies. Treatment is usually immunosuppression.
Contact Factor Deficiencies
Although factor XI deficiency may be associated with bleeding symptoms, deficiency in any of the other contact factors (i.e., factor XII, prekallikrein, and high-molecular-weight kininogen) is not associated with bleeding symptoms, and thus these factors do not appear to play a major physiologic role in normal hemostasis (see Chapter 27 ). Because these contact factors function at the initiation step of the intrinsic system, the APTT in severely deficient individuals (i.e., persons with contact factor activity less than 1% of normal) frequently demonstrates prolongations beyond those seen with hemophilia A and hemophilia B. As a result, one encounters the paradoxic situation in which an extremely prolonged PTT is not associated with a bleeding disorder.
Clinicians usually encounter contact factor deficiency when they are asked to evaluate a patient without bleeding symptoms who is found to have a markedly prolonged APTT on a preoperative screening test. It is frequently advisable to carry out these same screening tests for other family members once the specific contact factor deficiency is determined to identify affected family members who could have future surgery inappropriately delayed because of the need for such clotting studies.
Paradoxically, in a number of case reports, venous thromboembolism or myocardial infarction has been noted in factor XII–deficient patients, including the initial patient described with the abnormality. This thrombophilic tendency has been attributed to reduced plasma fibrinolytic activity. In a literature review of 121 patients with factor XII deficiency, an 8% incidence of thromboembolism was found, including several myocardial infarctions in relatively young individuals. Interpretation of such data is difficult because unlike in asymptomatic patients with factor XII deficiency, these complications are likely to be reported in the literature. This situation led some groups to perform cross-sectional analyses of thromboembolic events in larger numbers of unselected families with factor XII deficiency. In Swiss families with factor XII deficiency, 2 of 18 homozygous or doubly heterozygous patients had experienced deep venous thrombosis, although each episode occurred at a time when other predisposing thrombotic risk factors were present. Among heterozygotes with factor XII deficiency, only 1 in 45 had a possible history of venous thrombosis. These investigators concluded that heterozygous factor XII deficiency does not constitute a major thrombotic risk factor, although a severe deficiency may predispose some affected persons to venous thrombosis. Other groups have found a 10% to 20% incidence of thrombotic episodes in heterozygotes. Thus it remains uncertain whether an increased thrombotic risk is associated with factor XII deficiency.
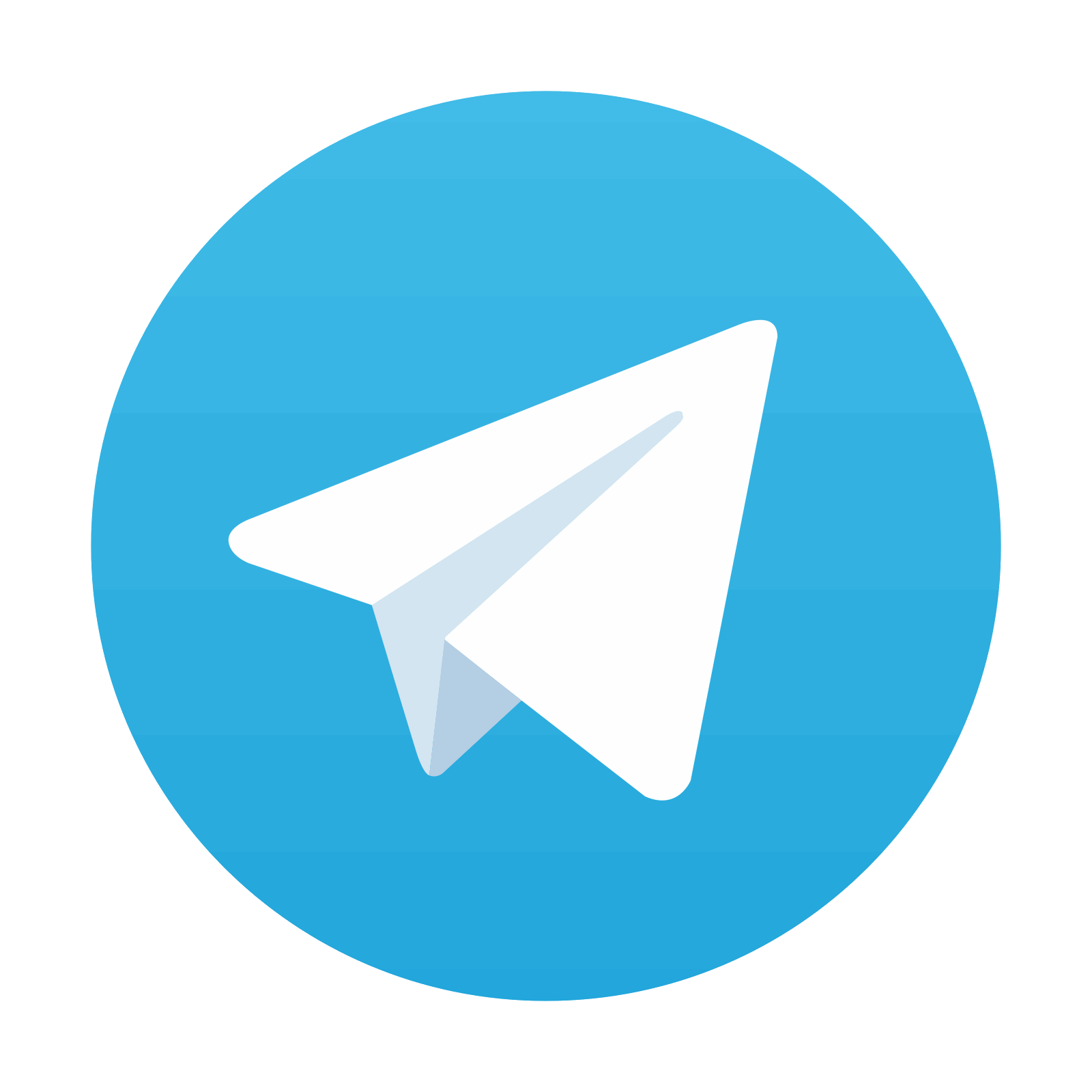
Stay updated, free articles. Join our Telegram channel

Full access? Get Clinical Tree
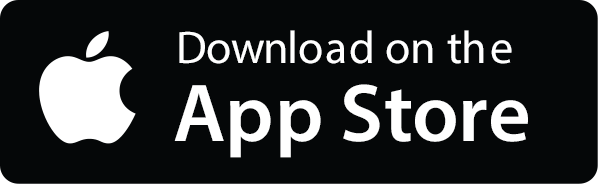
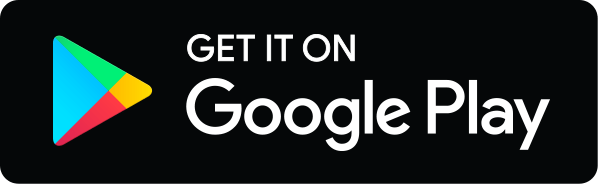