Principles and Definitions
Barry B. Lowitz
Dennis A. Casciato
“If two treatments are equally ineffective, and one has fewer side effects, use neither.”
B.B.L.
I. SOME PRINCIPLES OF CANCER BIOLOGY AND CANCER TREATMENT
A. Normal cell reproduction
1. The cell cycle is depicted in Figure 1.1. Cell replication proceeds through a number of phases that are biochemically initiated by external stimuli and modulated by both external and internal growth controls. Certain oncogenes and cell cycle-specific proteins are activated and deactivated synchronously as the cell progresses through the phases of the cell cycle. Most cells must enter the cell cycle to be killed by chemotherapy or radiation therapy. Many cytotoxic agents act at more than one phase of the cell cycle, including those classified as phase specific.
a. In the G0 phase (gap 0 or resting phase), cells are generally programmed to perform specialized functions. An example of drugs that are active in this phase is glucocorticoids for mature lymphocytes.
b. In the G1 phase (gap 1 or interphase), proteins and RNA are synthesized for specialized cell functions. In late G1, a burst of RNA synthesis occurs, and many of the enzymes necessary for DNA synthesis are manufactured. An example of drugs that are active in this phase is L-asparaginase.
c. In the S phase (DNA synthesis), the cellular content of DNA doubles. Examples of drugs that are active in this phase are procarbazine and antimetabolites.
d. In the G2 phase (gap 2), DNA synthesis ceases, protein and RNA syntheses continue, and the mícrotubular precursors of the mitotic spindle are produced. Examples of drugs that are active in this phase are bleomycin and plant alkaloids.
e. In the M phase (mitosis), the rates of protein and RNA synthesis diminish abruptly, while the genetic material is segregated into daughter cells. After
completion of mitosis, the new cells enter either the G0 or the G1 phase. Examples of drugs that are active in this phase are plant alkaloids.
completion of mitosis, the new cells enter either the G0 or the G1 phase. Examples of drugs that are active in this phase are plant alkaloids.
2. Cyclins activate the various phases of the cell cycle. Most normal cells capable of reproduction proliferate in response to external stimuli, such as growth factors, certain hormones, and antigen—histocompatibility complexes, which affect cell-surface receptors. These receptors then transduce the signal that results in cell division. Tyrosine kinases (TKs) are an essential part of the cascade of proliferative signals, from extracellular growth factors to the nucleus. Cyclins combine with, activate, and direct the action of special TKs, called cyclin-dependent kinases.
3. Cell cycle checkpoints. Cells that are capable of reproducing are normally stopped at specific phases of the cell cycle called checkpoints. The most important of these are immediately preceding the initiation of DNA synthesis and immediately preceding the act of mitosis. These histologically quiescent periods are probably mediated by decreased activity of cyclin-associated kinases and tumor-suppressor proteins. In fact, the cells in these phases are biochemically active as they prepare proteins to enter the next phase of the cell cycle and correct any genetic defects before going on to reproduce.
a. Normal cells have mechanisms that detect abnormalities in DNA sequences. When DNA is damaged, a number of repair mechanisms replace damaged nucleotides with normal molecules. These mechanisms are most important during cellular reproduction to ensure that new genetic material in daughter cells is an exact copy of the parent cell.
b. The first checkpoint occurs in the late G1 phase, just before cells enter the S phase. Even if the proper extracellular signals are received and all of the machinery is in place for DNA synthesis, the DNA must be in an acceptable state, with no lesions, before the cell can leave G1. If lesions are detected, either they are repaired or the cell is made to undergo apoptosis. This stopping point is one of the actions of the p53 protein (see Section I.C.3.b).
c. The second checkpoint occurs just before the cell enters the M phase; the cell cycle inhibitors stop the cell until it is determined whether the new progenies are worthy successors with accurate genetic copies of the parent. A cell that has not completely and accurately replicated all of its DNA or that does not have the full complement of proteins, spindle materials, and other substances to complete mitosis is arrested at this checkpoint until everything is in order and before the M phase can begin.
4. Normal populations of cells have a small component of “immortal cells” that, when called on by signals from other parts of the organism, can replenish themselves and also supply daughter cells that mature and differentiate into specialized tissue cells necessary for the function of the whole organism. Although a few types of tissue can dedifferentiate, most cell types lose their vitality as they differentiate, enter senescence, and eventually die. The following four populations of normal cells can be identified in eukaryotes:
a. Germ cells, which are capable of reproducing themselves indefinitely, possibly as a result of going through meiosis. Unlike cancer cells, these cells must undergo a meiotic “event” to produce an immortal cell line.
b. Stem cells, whose only two functions are to reproduce and to produce cells destined to differentiate and perform specialized functions for the host. Unlike cancer cells, these cells have a limited biologic number of reproductive cycles.
c. Partially differentiated cells, which have limited capability to reproduce and whose progeny eventually become fully differentiated nonreproducing cells
d. Fully mature specialized cells, which cannot reproduce further generations
5. Differentiation is inversely related to immortality. Unlike cancer cell lines that are immortal by definition, differentiated normal cells have a biologic “clock” that counts the number of times the cell can divide, after which no further division is possible. For example, a human fibroblast in culture can divide about 50 times, no matter what it is fed or the conditions of its culture, and then it and its progeny can divide no further.
B. Characteristics of cancer cells. Cancer can be defined as a cellular disorder characterized by progressive accumulation of a mass of cells, as a result of excessive reproduction of cells not compensated by appropriate cell loss; these cells progressively invade and damage the tissues and organs of the host. Although cancer cells are abnormal and die at a faster rate than their normal counterparts, the death rate is not able to keep up with the formation of new cells. This imbalance is the result both of genetic abnormalities in cancer cells and of the inability of the host to detect and destroy such cells. Some of the unique characteristics of cancer cells follow.
1. Clonal origin. Most cancer cells appear to originate from a single abnormal cell. Some cancers arise from multiple malignant clones either as a result of a field defect, in which multiple cells of a tissue are exposed to a carcinogen (such as the upper airways in smokers), or as a result of inherited defects in certain genes.
2. Immortality and telomeres. Most normal cells have limits on the number of reproductive cycles that a cell can have as it matures. Cancer cells, on the other hand, can proliferate indefinitely, providing an inexhaustible pool of precursor cells. One mechanism for immortality involves telomeres, the ends of chromosomes. Telomeres of most types of normal cells progressively shorten as the cells differentiate. In contrast, the telomeres of cancer cells and stem cells are replenished by the enzyme telomerase. This enzyme normally progressively decreases in a programmed manner as cells differentiate; the fully differentiated cell becomes senescent and eventually dies as it loses its ability to reproduce. In contrast, telomerase production is preserved or activated in many types of cancer cells; consequently, the length of the telomeres remains intact, and the cell remains “immortal.”
3. Genetic instability due to defects in DNA repair and in detection of DNA mismatches leads to heterogeneity of cancer cells. The cancer cells produce clones that become progressively less responsive to control mechanisms of proliferation and have an increased capacity to survive in “foreign environments” as metastases.
4. Loss of contact inhibition and anchorage-dependent growth. Normal cells grown in tissue culture do not divide unless they become anchored to a solid substratum to which they can adhere. Normal cells also stop dividing when they attain a confluent monolayer, even if the culture medium contains all the growth factors and nutrients necessary for further division. Cancer cells can grow independently in a semisolid medium without the requirement for substrate adherence; they continue to proliferate beyond a confluent monolayer in cell culture.
5. Progressive independence of proliferation from growth factors and nutrients is noted in cancer cell cultures. Cancer cells can actually self-destruct by continuing to divide even after they have consumed the nutritional factors in the culture media necessary for their survival.
6. Metastasis is a feature of cancer that is not found in normal tissues or benign tumors. The ability to metastasize results from the loss of or abnormalities of
cellular proteins responsible for adhesion to the extracellular matrix, abnormalities in the interaction between cells, abnormal attachment to basement membrane, abnormal production of basement membrane, destruction of basement membrane by enzymes such as the metalloproteases (collagenases), and many other factors.
cellular proteins responsible for adhesion to the extracellular matrix, abnormalities in the interaction between cells, abnormal attachment to basement membrane, abnormal production of basement membrane, destruction of basement membrane by enzymes such as the metalloproteases (collagenases), and many other factors.
C. Causes of overproduction of cancer cells
1. Failure of abnormal cells to undergo apoptosis. Apoptosis is programmed cell death. An initial stimulus sets off an extremely complex cascade of events eventually resulting in apoptosis.
a. Apoptosis occurs in normal tissue reabsorption; the classic example is the disappearance of tadpoles’ tails. Apoptosis also results in the disappearance during embryogenesis of webs between fingers of primates, allowing the formation of individual digits. Apoptosis results in the elimination of normal senescent cells when they become old and useless and of thymic T cells that recognize “self” and thereby prevent immune attack by these cells on the host.
b. Apoptosis eliminates cells with abnormal DNA caused by either irreparable DNA damage or by inaccurate, incomplete, or redundant transcription of DNA. This is a major mechanism for maintaining chromosome number in cells of a particular species and in preventing aneuploidy. The process ensures that only cells that have fully and accurately replicated their entire DNA can enter mitosis.
c. Apoptotic cells can be recognized microscopically. Apoptotic cells show clumps of intracellular organelles in the absence of necrosis. The nuclei are condensed and fragmented; intracellular structures are degenerated and compartmentalized. As the cell falls apart, phagocytes take up the fragments. Unlike the process of cell necrosis, apoptosis does not cause an inflammatory response. Apoptosis requires synthesis of specific proteins that have been highly conserved throughout evolution.
d. Apoptosis is genetically regulated and may be perturbed in malignant cells. Cancer cells and some immunologic cells produce substances that promote inappropriate apoptosis in normal tissues (and may contribute to the cachexia of malignancy). For example, the p53 tumor-suppressor oncogene stimulates apoptosis. The Bcl-2 oncogene inhibits apoptosis, decreases normal cell death, and increases cell populations. Apoptosis may be the major mechanism by which tumor cell populations are decreased by hormones, cytotoxic chemotherapy, and radiation therapy.
e. Caspases. The final stage of the various death pathways is mediated through activation of the caspases, which represent a family of cysteine proteases. The activation of caspases is determined by the intrinsic and extrinsic pathways of apoptosis.
The intrinsic pathway is a mitochondrial-dependent pathway mediated by the Bcl-2 family of proteins. Exposure to cytotoxic stress results in disruption of the mitochondrial membrane, which then leads to release of protease activators. Caspase-9 is subsequently activated, setting off a cascade of events that commits the cell to undergo apoptosis.
The extrinsic pathway is mediated by ligand binding to the tumor necrosis factor (TNF) family of receptors, which includes TNF-related apoptosis-inducing ligand and others, and certain essential adaptor proteins. These adaptor proteins recruit various proteases that cleave the N-terminal domain of caspase-8, which leads to activation of the caspase cascade.
2. Genetic abnormalities that inappropriately stimulate cell proliferation, independent of normal proliferation signals, occur through a variety of mechanisms. Mutations or overproduction of receptors or transducing proteins can cause the cell to become independent of growth factor or other triggers and to initiate cell division independently. These gene abnormalities are usually dominant (i.e., normal cells hybridized with abnormal cells become phenotypically malignant).
3. Abnormalities of tumor-suppressor genes (genes that are responsible for suppressing cell division) probably result in cancer through failure of the host to destroy genetically abnormal cells. These genes are recessive; malignant cells hybridized with normal cells become normal.
a. Hereditary tumors. Retinoblastoma gene (RB1) was the first of these abnormal genes to be discovered. Subsequently, a number of other suppressor gene abnormalities have been found, particularly in uncommon or rare hereditary diseases. Examples include Wilms’ tumor (WT1), familial polyposis (APC), familial melanoma (CDKN20), and familial breast and ovarian cancers (BRCA-1 and BRCA-2).
b. p53 Suppressor gene. The most important example of these genes is the p53 suppressor gene. The p53 protein is a gene product that suppresses the cell cycle with multiple complex activities. It can detect DNA lesions, such as nucleotide mismatches and DNA strand breaks, including those caused by radiation and chemotherapy. This function of p53 is thought to be critical in preserving the integrity of the cellular genome.
(1) When DNA lesions are detected, the p53 protein arrests cells in the quiescent G1 and G2 phases of the cell cycle, preventing cells from entering the DNA synthetic (S) phase of the cell cycle. The p53 protein can then induce repair mechanism proteins or trigger proteins, which cause apoptosis.
(2) In the absence of intact apoptosis, cancer cells can continue through sequential cell divisions and accumulate nucleotide mismatches and progressive DNA mutations.
(3) In vitro studies have shown that chemotherapy and radiation kill cancer cells through DNA damage, which triggers p53 protein—induced apoptosis. In contrast, p53 protein—deficient mouse thymocytes and resting lymphocytes remain viable after irradiation.
(4) Many human cancers are found to have mutant p53 suppressor genes. Mutant p53 is characteristic of Li-Fraumeni syndrome, a hereditary autosomal dominant syndrome of both soft tissue and epithelial cancers at multiple sites starting at an early age.
4. Tumor angiogenesis. Cancer colonies are not larger than about 1 mm in diameter unless they have a blood supply. Colonies without adequate blood supply are not resting (not in the G0 cell cycle phase); they typically have a high rate of proliferation but a fully compensating cellular death rate. After the blood supply is established, the cellular death rate decreases, and the tumor grows rapidly.
a. Several substances are required to promote formation of new blood vessels (angiogenesis) in normal tissues. Almost all measurable cancers, however, are limited to the production of only one of these factors, called vascular endothelial growth factor (VEGF), which induces blood vessel formation. VEGF has a number of interesting properties that may be useful for cancer treatment including the following:
(1) VEGF induces receptors for itself on mature and nonproliferating blood vessel endothelial cells. These normal, resting endothelial cells do not have the receptor until they are exposed to VEGF.
(2) VEGF induces the production and activity of multiple other growth factors that contribute to blood vessel formation.
(3) VEGF can be induced by c-ras and by other oncogenes and growth factors, which then induce further production of VEGF.
(4) Unlike normal blood vessels that require other factors for normal development, blood vessels induced by VEGF are “leaky.” VEGF-induced plasma proteins, such as fibrinogen, can leak out of the new vessels, forming a spongy gel around the tumor. This gel contains VEGF that induces further angiogenesis.
(5) VEGF appears to prevent apoptosis in induced endothelial cells.
b. Tumors also elaborate angiogenesis inhibitors, which can decrease growth of tumor at distant sites. One form of murine lung cancer gives rise to metastases that elaborate such inhibitors and suppress the growth of the primary site. This mechanism may account for the difficulty in locating primary tumors that present with metastases and for the absence of a detectable primary tumor (metastases of unknown origin).
5. Population kinetics.
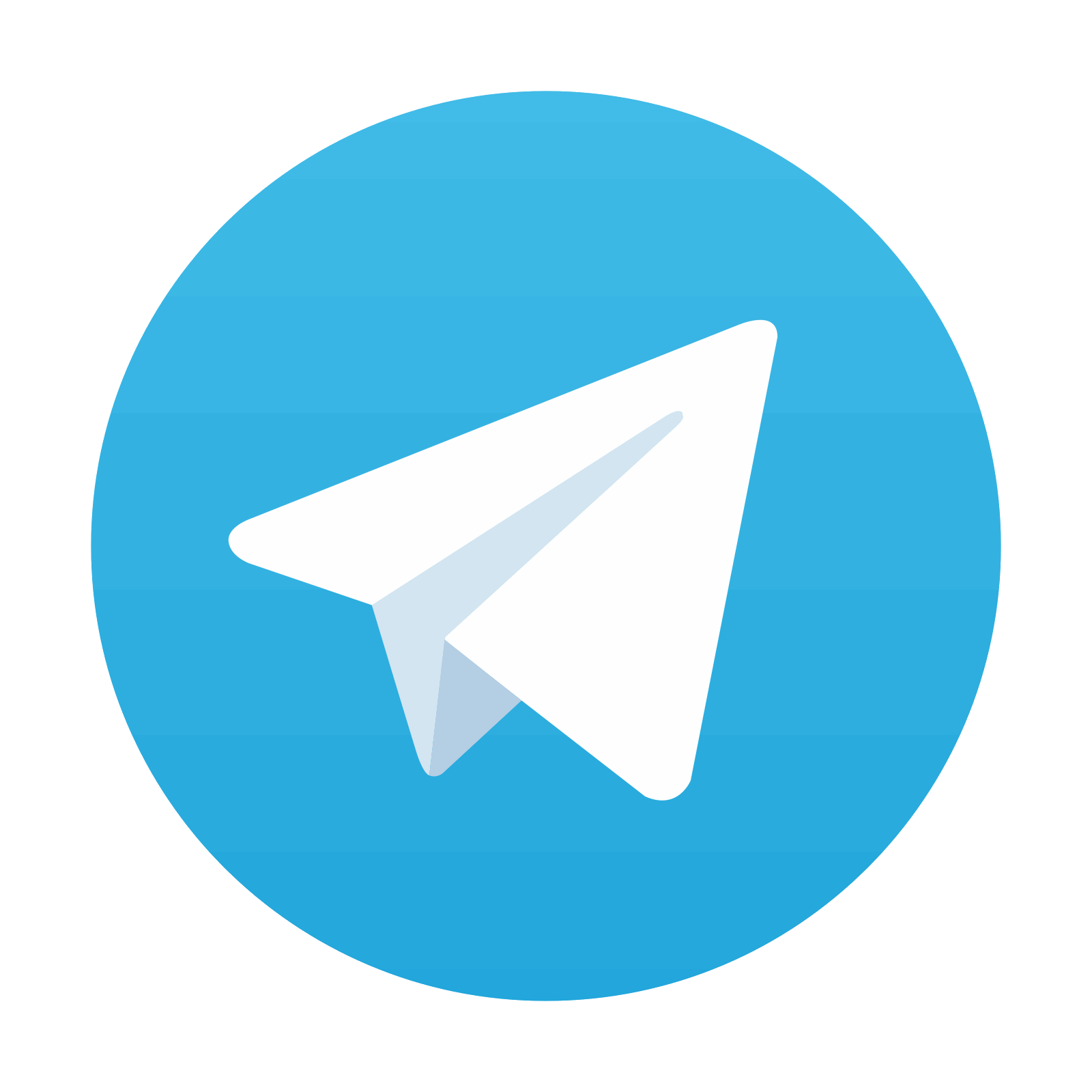
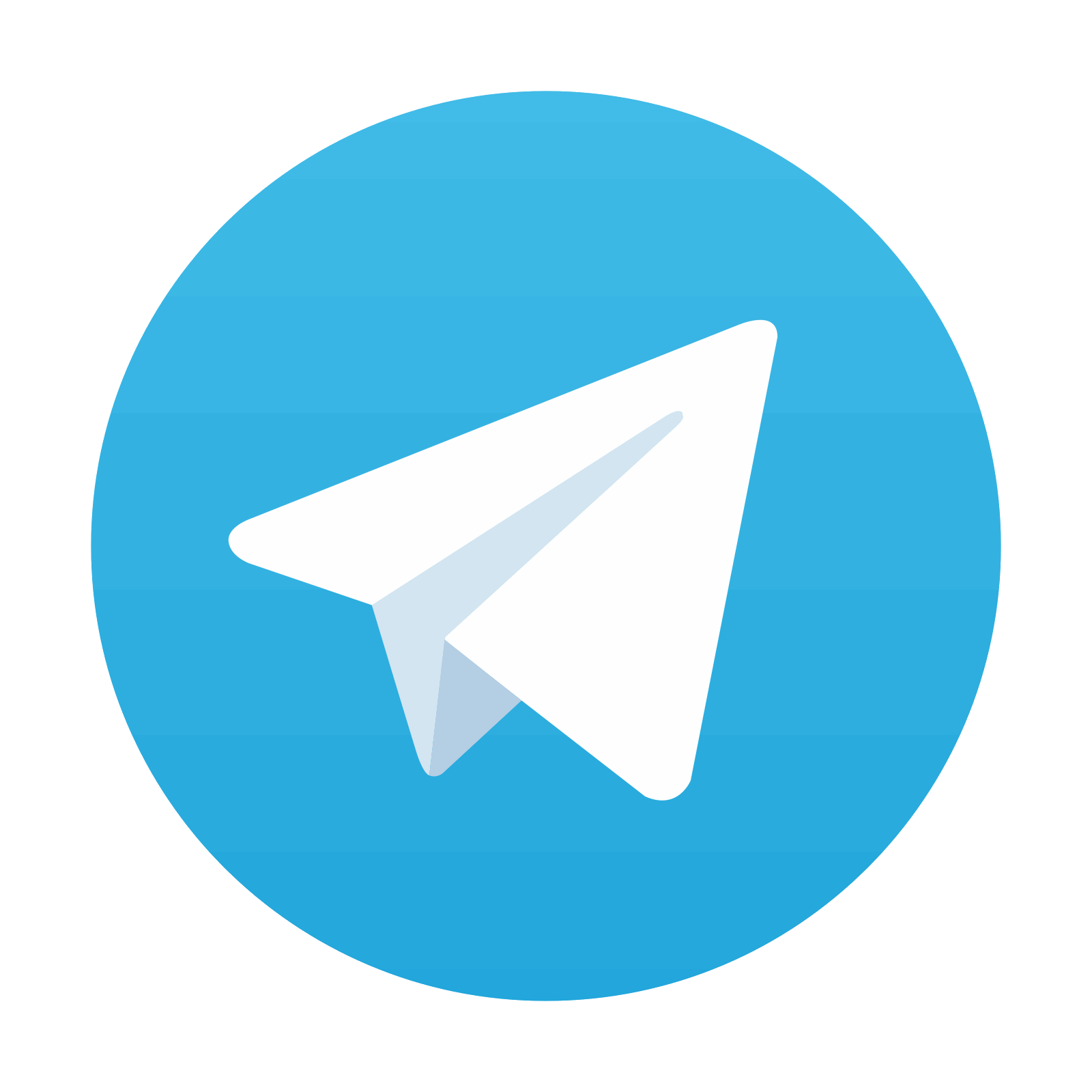
Stay updated, free articles. Join our Telegram channel

Full access? Get Clinical Tree
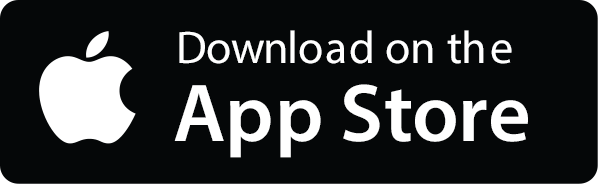
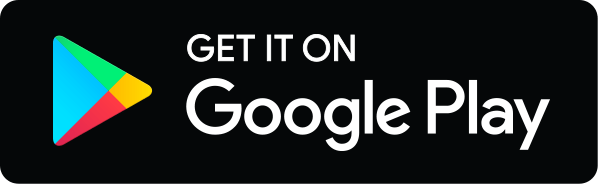