Primary Hypothyroidism Due to Other Causes
Peter A. Singer
Table 37.1 Other Causes of Primary Hypothyroidism | |
---|---|
|
Spontaneous primary hypothyroidism, which is nearly always due to chronic autoimmune (Hashimoto’s) thyroiditis, is the most common cause of hypothyroidism in countries where there is sufficient iodine intake. In the United States alone, cross-sectional studies of the elderly have demonstrated high serum thyrotropin (TSH) levels in from 6.7% to 19% of people, with a greater prevalence in women (1,2,3). Iodine deficiency is the principal cause of hypothyroidism in underdeveloped areas in the world, and may be present in as many as 1 billion people worldwide, although the actual prevalence of hypothyroidism in affected individuals is not known (see Chapter 11D).
This chapter will discuss other types of primary hypothyroidism, ranging from those encountered commonly, such as following radioactive iodine therapy (RAI) or thyroidectomy, as well as uncommon and even rare forms of hypothyroidism (Table 37.1). For the purpose of this discussion, hypothyroidism is defined as a sustained elevation of serum TSH, and will include subclinical hypothyroidism, characterized by normal serum levels of free thyroxine and high serum concentrations of TSH, as well as overt hypothyroidism.
Hypothyroidism Due to Thyroid Ablation
Treatment of Graves’ Disease (See Chapter 32)
Radioactive Iodine (131I Therapy)
131I therapy for thyrotoxic Graves’ disease is the most common cause of non-spontaneous hypothyroidism in the United States, probably because of physician preference for 131I, due to the relatively high relapse rate (∼70%) of thyrotoxicosis following withdrawal of antithyroid drug therapy (4). An international survey of thyroid specialists two decades ago revealed that 131I was chosen by 69% of physicians in the United States as the primary form of treatment for a typical 43-year-old woman with Graves’ thyrotoxicosis (5). In contrast, only 22% of physicians from Europe, 17% from Latin America, and 11% from Asia, selected 131I as the primary form of therapy (6,7). Most physicians in North America recommend a dose of 131I sufficient to ensure hypothyroidism, so that the need for T4 replacement will be predictable (8). Efforts to restore long-term euthyroidism with smaller doses of 131I have generally been unsuccessful, resulting in either persistent or recurrent thyrotoxicosis, or late onset of hypothyroidism. In one study of 187 patients in the United States treated with low doses of 131I, 12% became hypothyroid after 1 year, and 76% were hypothyroid at the end of 11 years of follow-up (9). A study in England yielded similar results, with 11% of patients becoming hypothyroid after 1 year, and 55% developing hypothyroidism after 15 years (10). A high-fixed dose of 131I (128 to 155 μCi/g of tissue) results in a cure of Graves’ thyrotoxicosis in 90% of patients, and the development of hypothyroidism in approximately 80% (1,2,3,11). Because hypothyroidism develops so frequently, regardless of the dose, the goal of long-term euthyroidism with 131I is not only difficult but also is probably unwise in the United States, whereas it was the preferred method of treatment by physicians in Latin America, Europe, and Asia (12).
Although euthyroidism after surgery is desirable, hypothyroidism usually develops; 59% of 81 patients who underwent subtotal thyroidectomy in the United States, and 51% of 216 patients who underwent similar surgery in Japan developed
hypothyroidism within several years (12,13). In both studies, a thyroid remnant of less than 6 g was left in order to avoid recurrent thyrotoxicosis. Other studies have yielded similar findings, including two studies from Europe, in which nearly 50% of patients who underwent subtotal thyroidectomy eventually became hypothyroid (14,15). Current recommendations are for subtotal or near-total thyroidectomy for Graves’ disease, so that the need for T4 therapy will be more predictable (15).
hypothyroidism within several years (12,13). In both studies, a thyroid remnant of less than 6 g was left in order to avoid recurrent thyrotoxicosis. Other studies have yielded similar findings, including two studies from Europe, in which nearly 50% of patients who underwent subtotal thyroidectomy eventually became hypothyroid (14,15). Current recommendations are for subtotal or near-total thyroidectomy for Graves’ disease, so that the need for T4 therapy will be more predictable (15).
Treatment of Toxic Nodular Goiter (See Chapters 20 and 32)
Both 131I and surgery are commonly used for the treatment of patients with thyrotoxicosis due to either single or multiple autonomously functioning thyroid nodules. Because 131I is mainly concentrated in autonomously functioning tissue, extranodular thyroid tissue is for the most part spared the effects of 131I. However, between 6% and 60% of patients have been reported to become hypothyroid following 131I treatment of single autonomously funcitioning nodules, regardless of the dose of 131I administered (16,17,18). Patients who become hypothyroid are likely those in whom 131I uptake in non-nodular thyroid tissue was not completely suppressed (16,18). RAI accumulation in the contralateral lobe assumes additional importance in younger patients, who may be subject to nodule formation many years following 131I therapy; hence the common recommendation is that younger patients (perhaps <30 years of age) be treated surgically (19,20). It has been suggested that administration of low doses of 131I (5–15 mCi) to patients with autonomous nodules minimizes the likelihood of posttreatment hypothyroidism (21).
Both surgery and RAI are effective treatments for toxic multinodular goiter (TMNG). Hypothyroidism following surgery for TMNG has varied from as low as 2%, (22) to 89% where surgery consists of near-total thyroidectomy (23). Of 61 patients treated with 131I, 28% became hypothyroid within one year after therapy (23).
Treatment of Nontoxic Nodular Goiter (See Chapters 48 and 49)
Patients undergoing near-total thyroidectomy for nontoxic nodular goiter usually develop hypothyroidism, whereas partial thyroidectomy results in a lower incidence (24,25). Incomplete surgery, however, increases the possibility of recurrence, with regrowth reported to be as high as 10% to 20% of patients approximately 10 years after surgery, and 40% to 45% 30 years after surgery (25). Radioiodine treatment of nontoxic nodular goiter also appears to result in an appreciable incidence of hypothyroidism, with approximately 20% to 30% developing high serum TSH levels within 5 years after therapy (26). This form of treatment has been used in the United States less often than in Europe (27,28,29,30). Recently there has been more interest in the use of recombinant TSH to enhance 131I uptake, thereby lowering dose requirements of 131I (31,32).
Euthyroid patients undergoing lobectomy for single nodules may develop hypothyroidism when exposed to pharmacologic doses of iodides, suggesting that patients with apparent uninodular disease may have a more diffuse underlying thyroid abnormality (33).
External Radiation
Hypothyroidism is common after external radiotherapy with doses of 25 Gy (2,500 rad) or more to the head and neck area for malignant tumors, including Hodgkin’s and non-Hodgkin’s lymphoma, and solid cancers of the head and neck. Hypothyroidism may develop within the first year after radiotherapy, especially in patients under 20 years of age but it typically develops within 3 to 5 years, and it may continue to develop many years later (34). In a review of 1,677 Hodgkin’s lymphoma patients treated with external radiotherapy, the risk of hypothyroidism was 52% after 20 years, and 67% 26 years after therapy (35). This risk appears to be dose-dependent.
While hypothyroidism associated with external radiotherapy is usually due to thyroid destruction, the development of thyroid autoimmunity has been shown to be related to radiotherapy for Hodgkin’s disease, including both Hashimoto’s and Graves’ disease, and ophthalmopathy (36,37).
When laryngectomy or partial thyroidectomy is done in addition to external radiotherapy, the likelihood of hypothyroidism increases to 65% to 70% (38). Hypothyroidism occurs in 30% to 40% of children and adolescents who receive whole-body irradiation for either aplastic anemia or leukemia, and in approximately 6% of children who receive craniospinal radiation for brain tumors (39,40). Hypothyroidism also occurs in 9% to 16% of adults who undergo total body irradiation prior to bone marrow transplantation (41).
Chemotherapy also appears to be associated with the development of hypothyroidism in patients with lymphoma (35) and the combination of chemotherapy and external radiotherapy results in a greater incidence of hypothyroidism than occurs with either chemotherapy or external radiotherapy alone (36,42,43).
Because hypothyroidism may be either an early or late sequela of external radiotherapy, long-term surveillance of irradiated patients with serum TSH testing is indicated.
Hypothyroidism Due to Therapeutic Pharmacologic Agents
Iodides, thionamide drugs, and other agents used in the treatment of thyrotoxicosis are discussed in Chapter 32. This section will focus on agents not used primarily for the treatment of thyroid disease.
Lithium Carbonate
Lithium carbonate is usually prescribed for the treatment of manic-depressive disorders, and may be associated with the development of both goiter and hypothyroidism. Goiter has been reported in 4% to 60% of patients receiving lithium, and
is due primarily to its inhibitory effect on thyroid hormone secretion, resulting in TSH stimulation of the thyroid (44,45,46). The goiter is typically diffuse. A review of 4,681 lithium-treated patients revealed the overall prevalence of hypothyroidism to be 3.4%, with a range of 0% to 23.3% (47,48). Risk factors for the development of hypothyroidism include female sex and the presence of thyroid antibodies; patients who develop hypothyroidism during lithium therapy likely have underlying chronic autoimmune (Hashimoto’s) thyroiditis (49,50). Lithium-treated patients with no thyroid antibodies may have transient increases in serum TSH (51). Most lithium-treated patients who develop hypothyroidism have subclinical hypothyroidism, with only mild to moderate increases in serum TSH and normal serum thyroid hormone concentrations, although overt hypothyroidism may occur (52,53). Exogenous iodides act synergistically with lithium, resulting in greater serum TSH increases than with lithium alone (54). Because of lithium’s effects on the thyroid, patients for whom the drug is prescribed should have a serum TSH determination at the time of initiation of therapy, and every 6 to 12 months thereafter, or more frequently as the clinical situation dictates. Lithium therapy is also associated with a greater prevalence of painless thyroiditis than in the general population (55). Although the mechanism for this is unclear, it might be due to induction of an autoimmune process, or to a direct toxic effect on thyroid cells (56) Treatment for lithium-induced hypothyroidism is levothyroxine replacement.
is due primarily to its inhibitory effect on thyroid hormone secretion, resulting in TSH stimulation of the thyroid (44,45,46). The goiter is typically diffuse. A review of 4,681 lithium-treated patients revealed the overall prevalence of hypothyroidism to be 3.4%, with a range of 0% to 23.3% (47,48). Risk factors for the development of hypothyroidism include female sex and the presence of thyroid antibodies; patients who develop hypothyroidism during lithium therapy likely have underlying chronic autoimmune (Hashimoto’s) thyroiditis (49,50). Lithium-treated patients with no thyroid antibodies may have transient increases in serum TSH (51). Most lithium-treated patients who develop hypothyroidism have subclinical hypothyroidism, with only mild to moderate increases in serum TSH and normal serum thyroid hormone concentrations, although overt hypothyroidism may occur (52,53). Exogenous iodides act synergistically with lithium, resulting in greater serum TSH increases than with lithium alone (54). Because of lithium’s effects on the thyroid, patients for whom the drug is prescribed should have a serum TSH determination at the time of initiation of therapy, and every 6 to 12 months thereafter, or more frequently as the clinical situation dictates. Lithium therapy is also associated with a greater prevalence of painless thyroiditis than in the general population (55). Although the mechanism for this is unclear, it might be due to induction of an autoimmune process, or to a direct toxic effect on thyroid cells (56) Treatment for lithium-induced hypothyroidism is levothyroxine replacement.
Cytokines
Interferon-α
Interferon-α (IFN-α) is a human recombinant cytokine used for the treatment of chronic hepatitis carcinoid, Kaposi’s sarcoma, chronic myelogenous leukemia, and hairy cell leukemia (57). It is also used in combination with interleukin-2 (IL-2), a cytokine sometimes used in the treatment of tumors (58).
Hypothyroidism was initially described as a side effect of IFN-α therapy in patients receiving long-term IFN-α treatment for breast cancer (59). IFN-α-associated abnormalities include both thyrotoxicosis and hypothyroidism, with the latter being much more common (60). A biphasic pattern of thyroid dysfunction, with thyrotoxicosis followed by hypothyroidism, is often seen, similar to that observed with silent thyroiditis (61). The observed incidence of IFN-α-associated hypothyroidism in patients with HCV has been reported to vary from 7% to 39% (58,61,62,63,64). The addition of ribavirin to IFN-α therapy may increase the risk of hypothyroidism (65).
Risk factors associated with the development of hypothyroidism include female sex, longer duration of IFN-α treatment, presence of HCV, older age, and the preexisting presence of thyroid peroxidase (anti-TPO) antibodies (61). Asian origin is an independent risk factor for IFN-α-induced hypothyroidism (66). The presence of anti-TPO antibodies may be the most important risk factor; in one study, thyroid dysfunction occurred in 60% of patients receiving IFN-α who had anti-TPO antibodies before therapy, compared with only 3.3% of patients who did not have anti-TPO antibodies at that time (67). Of note, however, is that anti-TPO antibodies may develop in between 10% and 20% of IFN-α treated patients, who were previously antibody negative (68). Pegylagted interferons have a similar propensity to develop thyroid dysfunction (69).
Transient hypothyroidism occurs more commonly with IFN-α therapy than does persistent hypothyroidism, although data from several series indicate that 30% to 44% of patients who develop hypothyroidism during IFN-α treatment have persistent thyroid failure (58,61). The presence of thyroid antibodies at the end of IFN-α treatment is a positive predictive factor for persistent hypothyroidism (70).
The precise mechanism of IFN-α-induced thyroid dysfunction is not known, although it is probably related to activation or enhancement of the autoimmune process. Patients with HCV also have a higher baseline prevalence of anti-TPO antibodies, suggesting an association between HCV and an underlying thyroid autoimmunity (64,71).
Interleukin-2
Patients treated with IL-2 for tumors, especially melanoma and renal cell cancers, have an increased risk of hypothyroidism. In one series, 32% of 111 patients with cancer developed hypothyroidism during IL-2 treatment, and 14% had hypothyroidism post therapy, persisting from 44 to more than 149 days (median 54 days) (72). As with IFN-α-treated patients, positive thyroid antibodies and female sex are risk factors for thyroid dysfunction, whereas the type of tumor is not a predisposing factor (73). Patients who developed persistent hypothyroidism had more severe biochemical hypothyroidism during IL-2 treatment than those who had transient hypothyroidism (74). The likelihood of developing hypothyroidism is increased if therapy with IL-2 is combined with lymphokine-activated killer cells (75).
As with IFN-α, IL-2 treatment likely activates the autoimmune process. Administration of IL-2 is associated with both the development of thyroid antibodies and increased titers of thyroid antibodies in patients who had antibodies at baseline (73). Combination therapy with IFN-α and IL-2 leads to an even greater prevalence of thyroid dysfunction than with either alone (76).
Because thyroid dysfunction is so common in patients treated with IFN-α or IL-2, measurements of serum TSH and anti-TPO antibodies before initiation of therapy is indicated, as well as during the course of therapy.
Amiodarone
The antiarrhythmic drug amiodarone is discussed in detail elsewhere (see Chapters 11B and 11E). Suffice it to say, this heavily iodinated compound (a 300-mg dose provides 11 mg of inorganic iodine) (77) results in hypothyroidism from ∼5 to 15% of patients taking the drug (78). Risk factors for the development of hypothyroidism include female gender, the presenceof thyroid antibodies before initiation of treatment, and residence in an iodine repleted area (78). Amiodarone is lipophilic, and has a prolonged half-life (up to 2 months), so its effects may be sustained. It is recommended that patients in whom amiodarone is prescribed have serum TSH measured at initiation
of therapy, and at periodic intervals thereafter. Treatment of hypothyroisim is L-T4; discontinuation of amiodarone would not be indicated.
of therapy, and at periodic intervals thereafter. Treatment of hypothyroisim is L-T4; discontinuation of amiodarone would not be indicated.
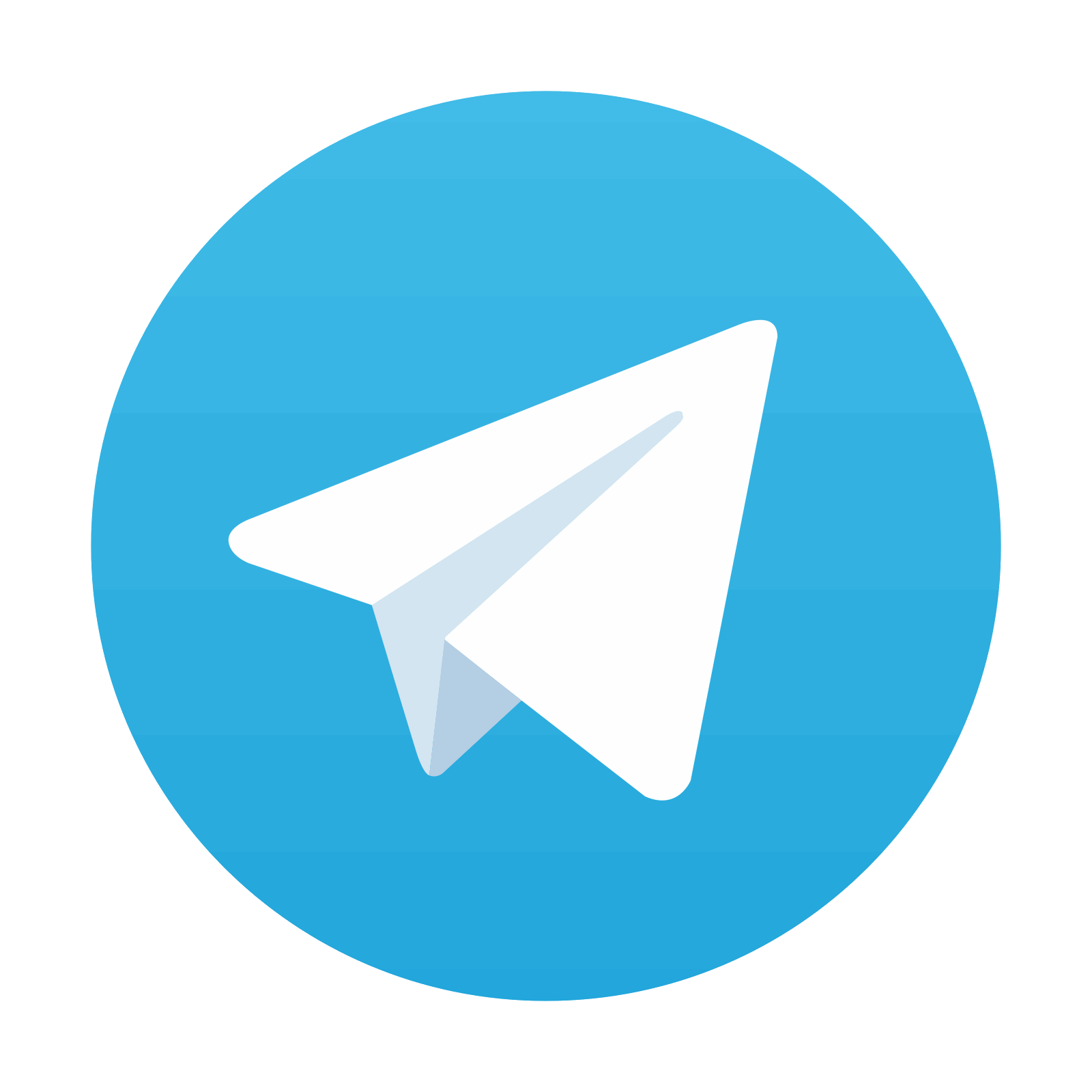
Stay updated, free articles. Join our Telegram channel

Full access? Get Clinical Tree
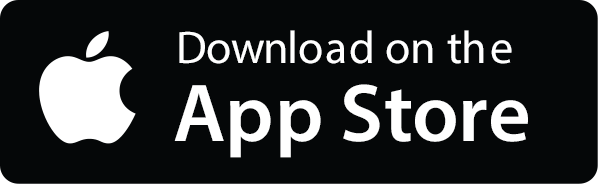
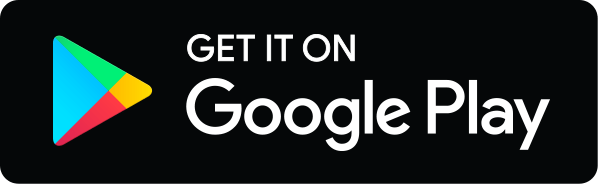