Disease
Defective gene
Protein
Inheritance
Protein function
FHL syndromes without hypopigmentation
FHL2
PRF1
Perforin
AR
Pore formation
FHL3
UNC13D
Munc13-4
AR
Vesicle priming
FHL4
STX11
Syntaxin11
AR
Vesicle fusion
FHL5
STXBP2
Munc18-2
AR, AD
Vesicle fusion
FHL syndromes with hypopigmentation
Chediak-Higashi syndrome
CHS1/LYST
LYST
AR
Vesicle sorting
Griscelli syndrome type 2
RAB27A
Rab27a
AR
Vesicle docking
Hermansky-Pudlak syndrome type 2
AP3B1
AP-3 complex β3A subunit
AR
Vesicle polarization
Hermansky-Pudlak syndrome type 9
PLDN
Pallidin
AR
Vesicle polarization
X-linked lymphoproliferative disease
XLP type 1
SH2D1A
SAP
XL
SLAM family receptor signaling
XLP type 2
XIAP/BIRC4
XIAP
XL
Inhibition of cell death and inflammasome activation
Based on genetics, FHL has been categorized into five subtypes. The causative gene of FHL1 is yet to be identified, and it is unknown if the patients had any defect in the lymphocyte cytolytic activities. FHL2-5 are caused by mutations in the genes encoding perforin (PRF1; FHL2), Munc13-4 (UNC13D; FHL3), syntaxin-11 (STX11; FHL4), and syntaxin-binding protein 2 (also known as Munc18-2) (STXBP2; FHL5) [5–9]. Perforin is an effector molecule contained in cytolytic granules. Munc13-4, syntaxin-11, and Munc18-2 are involved in intracellular trafficking or the fusion of those granules to the plasma membrane and the delivery of their contents into target cells (Fig. 12.1). As a result, defective cytotoxic activity of CTLs and NK cells is the hallmark findings of FHL. Because the trafficking of melanosomes shares machinery with that of cytotoxic granules, primary HLH includes disorders characterized by oculocutaneous albinism and impaired lymphocyte cytotoxicity (Fig. 12.1). Chediak-Higashi syndrome (CHS) is caused by mutations in LYST, Griscelli syndrome (GS) type 2 by mutations in RAB27A, and Hermansky-Pudlak syndrome (HPS) type 2 by mutations in AP3B1 [10–13]. Majority of patients with CHS and GS type 2 develop symptom of HLH; however, few cases of HLH in HPS type 2 have been described in literature [14–16]. PLDN mutations have recently been identified to cause HPS type 9, but it is not clear if full-blown HLH accompanies this disorder [17].


Fig. 12.1
Overview of the granule-dependent cytotoxic pathway. The granule-dependent cytotoxic pathway is a major immune effector mechanism employed by CTLs and NK cells. The pathway involves a series of steps including delivery of the lysosomal granules to the immunological synapse, exocytosis of lytic proteins such as perforin and granzymes, and induction of apoptosis in the target cells
Two X-linked disorders associated with Epstein-Barr virus (EBV)-driven HLH without direct evidence of impairment in lymphocyte cytotoxicity are X-linked lymphoproliferative syndrome (XLP) type 1 and type 2. XLP type 1 is caused by a mutation in gene coding signaling lymphocyte activation molecule (SLAM)-associated protein (SAP) (SH2D1A) and XLP type 2 by mutations in gene coding X-linked inhibitor of apoptosis (XIAP) (BIRC4) [18–20]. Recently, mutations in genes including ITK, CD27, and MAGT1 are reported to cause EBV-associated lymphoproliferation and lymphoma; however, HLH seems to be an infrequent complication in these diseases [21].
12.3 Pathophysiology
In addition to its central role in inducing apoptosis in targets such as cells infected with intracellular pathogens and tumor cells, the granule-dependent cytotoxic pathway is involved in inducing apoptosis in antigen-presenting cells (APCs) and possibly in CTLs themselves. In healthy individuals, CTL reaction subsides with a small number of residual memory cells as the antigen is cleared. In FHL patients, impaired clearance of antigens and defective suppression of antigen presentation, together with the inhibition of apoptosis in CTLs themselves, result in hyperactivation of CTLs and excessive production of inflammatory cytokines such as interferon-γ (IFN-γ) (Fig. 12.2). This leads to secondary activation of other cells including macrophages to produce additional inflammatory cytokines and results in “cytokine-storm” state characteristic of HLH.


Fig. 12.2
Defective cytotoxic activity causes dysregulated CTL activation. In addition to its central role in inducing apoptosis in target cells, the granule-dependent cytotoxic pathway is involved in inducing apoptosis in APCs and in CTLs themselves. In healthy individuals, CTL reaction subsides with a small number of residual memory cells as the antigen is cleared. In FHL patients, impaired clearance of targets and defective suppression of antigen presentation, together with the inhibition of apoptosis in CTLs themselves, result in hyperactivation of CTLs and excessive production of inflammatory cytokines such as IFN-γ
In XLP type 1 patients, HLH development is often associated with EBV-infection. SAP-deficient CTLs from patients with XLP type 1 display a specific lytic defect against autologous and allogeneic EBV-infected B cells, as well as an impairment in activation-induced apoptosis of their own [22, 23]. These observations suggest that the pathogenesis of HLH development after EBV-infection in XLP type 1 is similar to that of FHL syndromes (Fig. 12.3).


Fig. 12.3
Hyperactivation of CTLs against EBV-infected B cells in SAP-deficient patients. SAP-deficient CTLs from patients with XLP type 1 display a specific lytic defect against EBV-infected B cells, as well as an impairment in their own activation-induced apoptosis
In contrast to XLP type 1, no evidence of cytolytic defect is reported for CTLs from XLP type 2 patients, and XIAP deficiency is associated with increased apoptosis of activated CTLs. Therefore, the mechanism behind HLH development in XLP type 2 seems to differ from that of FHL and XLP type 1. Recently, XIAP is shown to suppress inflammasome activity, and sustained elevation of serum IL-18 is reported in patients with XLP type 2 [24–26]. It is possible that pathophysiology of HLH in XLP type 2 may be similar to that of secondary HLH complicating auto-inflammatory disorders.
12.4 Clinical Manifestations
Primary HLH predominantly manifests in young infants but can potentially affect all age groups. Rare cases with fetal and elderly onset have been reported. Clinical presentation in these cases may be atypical and the diagnosis is often delayed [27, 28].
Classical manifestations of HLH include prolonged fever, progressive cytopenias, hepatosplenomegaly, liver dysfunction, and coagulopathy. Central nervous system (CNS) symptoms can be found in one- to two-thirds of FHL patients and include decreased level of consciousness, cranial nerve palsies, and seizures [29, 30]. FHL3 patients are more prone to have CNS symptoms compared to FHL2 [31]. FHL4 and XLP type 2 patients are reported to have milder and recurrent episodes of HLH compared to patients with other forms of primary HLH. It has recently been proposed that graded defects in lymphocyte cytotoxicity determine the severity of HLH associated with each subtype [32].
Beside symptoms of HLH, each subtype of primary HLH is characterized by specific symptoms. Colitis is seen in a significant proportion of patients with FHL5 and XLP type 2, and sensorineural hearing deficit is a common finding in FHL5 patients [33, 34]. Hypogammaglobulinemia is reported in patients with FHL5 and XLP types 1 and 2 [33, 35]. Lymphoma develops in one-third of XLP type 1 but not in type 2 patients [35]. Partial albinism accompanies CHS, GS type 2, and HPS type 2, and recurrent pyogenic infections are frequently observed in these patients. Bleeding diathesis is commonly seen in patients with CHS and HPS type 2 but usually requires no specific treatment. Progressive motor and sensory neuropathies and low cognitive abilities develop in CHS patients who survive into adulthood [36].
12.5 Diagnosis
Diagnostic criteria for HLH was proposed and updated by the Histiocyte Society based on studies of FHL syndromes [2]. The criteria include two clinical symptoms and six laboratory findings. Fever is the first criterion and is a common symptom of HLH; however, it is often absent in neonates. Splenomegaly with or without hepatomegaly is also on the list. Laboratory findings include cytopenias affecting at least two of three lineages in the peripheral blood; hypertrigryceridemia and/or hypofibrinogenemia; hemophagocytosis in the bone marrow, spleen, or lymph nodes; low or absent NK-cell activity; hyperferritinemia; and high levels of sIL-2R. HLH is considered present if at least five out of eight features are positive.
Leukocyte count at diagnosis is variable among patients; however, neutropenia and thrombocytopenia are typical findings of FHL patients from the early course of the disease. Analysis of bone marrow aspirates is commonly performed for diagnosing HLH, but hemophagocytosis is detected in only half to two-thirds of patients with FHL. Thus, a negative result should not preclude initiation of therapy when there is high clinical suspicion of FHL. Serum levels of sIL-2R and ferritin are elevated in HLH and primarily reflect activation of T lymphocytes and macrophages, respectively. Since the activation of CTLs precedes that of macrophages in FHL, sIL-2R elevation is more prominent compared to that of ferritin in early course of the disease [37]. Low or absent NK cell activity is a common finding of FHL. However, transient impairment of NK cell activity is also a frequent finding in patients with secondary HLH [38].
Several rapid screening tests are available to identify FHL patients (Fig. 12.4). Detection of perforin expression in NK cells with flow cytometry is a reliable method to screen for FHL2 in a given patient [39, 40]. FHL3 can be screened by detecting intra-platelet expression of Munc13-4 protein [41]. XLP types 1 and 2 can be identified by detecting the intracellular expression of SAP and XIAP proteins, respectively [42–44]. Surface expression of CD107a on NK cells and CTLs reflects the integrity of cytotoxic granular release and is useful for identification of FHL3-5, CHS, GS type 2, and HPS type 2 [38, 45]. Some patients with secondary HLH may have a defect in resting NK cell degranulation; however, these patients show normal degranulation of NK cells after culturing with IL-2 [38, 45].


Fig. 12.4
Flow cytometry-based screening tests to identify patients with primary HLH. (a) FHL2 screening by detecting perforin expression in NK cells. (b) Screening of XLP type 2 patients by detecting XIAP expression in lymphocytes (Courtesy of Dr. Hirokazu Kanegane). (c) Intra-platelet Munc13-4 expression assay identifies FHL3 patients. Lysosomal degranulation assay using (d) NK cells and (e) CD57 + CTLs
Genetic analysis confirms the diagnosis of primary HLH. The incidents of gene defects responsible for primary HLH subtypes vary among countries and ethnic groups. Yet, up to 70% of cases are due to mutations in two genes, PRF1 and UNC13D [46, 47].
FHL2 accounts for 20–50% of all FHL cases depending on the cohort studies. It is most common among African-Americans and mutation c.50delT is found very frequently. FHL2 is also the most common subtype of primary HLH in Japan, and c.1090-1091delCT mutation has been reported only in Japanese patients [48]. FHL3 covers 30–40% of FHL patients based on geographic and ethnic groups. FHL3 is the most common form of primary HLH in Korea and among Caucasians in the US [46, 49]. In Japan, it accounts for one-third of FHL cases [48]. It has become evident in 2011 that deep intronic mutation c.118-308C>T and the 253-kb inversion affecting the 3′-end of UNC13D transcript abolish Munc13-4 expression and account for significant proportion of FHL3 in northern European populations [50]. Original reports of FHL4 were restricted to families of Turkish/Kurdish origin, but patients of different origin have been reported recently [51, 52]. FHL4 patients seem to have a late onset and a milder clinical presentation compared to FHL2 and FHL3. FHL5 is reported to account for 20% of FHL cases in German cohort and seem to be found in every geographic region [33]. Clinical findings of FHL5 include colitis, bleeding tendency, and hypogammaglobulinemia in addition to typical presentation of HLH. A recent study described two unrelated FHL5 patients with mono-allelic dominant-negative mutations, c.193C>T and c.194G>A, in STXBP2 gene [53].
Incidence of primary HLH syndromes with albinism is much lower than that of FHL [47]. In Japan, only one or two CHS patients are newly diagnosed every year [54]. The pathognomonic feature of CHS is the presence of giant lysosomes and related organelles in various cell types. Decreased pool of platelet dense granules is associated with bleeding diathesis, while a defect in antimicrobial activity is due to the decrease of neutrophil enzymes. In neuronal cells, giant granule formation might have a role in the pathophysiology of neurological manifestations. CHS clinical phenotypes correlate with molecular genotypes; nonsense or frameshift mutations that cause early truncation of the protein are associated with early-onset disease characterized by severe infections and HLH while missense mutations are associated with milder, late-onset course of disease with progressive neuropathies [55]. In most patients with GS type 2, HLH develops between 6 and 12 months of age, later than in patients with FHL2 but earlier than in patients with FHL4 and CHS [32]. Neurological manifestations in GS type 2 patients occur only in association with the development of HLH because RAB27A gene is not expressed in neuronal cells. In 2015, GS type 2 patients without albinism were reported. Rab27a protein interacts with Munc13-4 and melanophilin. RAB27A mutations identified in these patients selectively disrupted the interaction of Rab27a protein with Munc13-4 but not with melanophilin [56]. HPS is a group of at least nine genetic disorders characterized by oculocutaneous albinism and bleeding diathesis. Of these, HPS types 2 and 9 are associated with cytotoxicity impairment. However, only a few HPS type 2 patients with HLH development are reported in the literature [13, 16].
XLP is a rare inherited immunodeficiency affecting approximately one in 1,000,000 males. SH2D1A and BIRC4 genes, responsible for XLP types 1 and 2, respectively, are both located on Xq25 in close vicinity. However, it is now evident that two disorders are not functionally related despite their clinical similarities at first sight. SH2D1A mutation is responsible for 60–70% of XLP cases. XLP type 1 patients typically exhibit EBV-induced HLH, progressive hypogammaglobulinemia, and malignant lymphoma. BIRC4 mutation is found in 20–30% of XLP cases, and XLP type 2 patients typically present with EBV-triggered HLH, transient hypogammaglobulinemia during HLH episodes, hemorrhagic colitis, but not with malignant lymphoma [21, 57, 58].
12.6 Treatment
Current management of primary HLH involves a two-step approach. First step is a short-term immunosuppressive therapy aiming at controlling the inflammatory state. Second step aims at correcting the underlying genetic defect by allogeneic hematopoietic stem cell transplantation (HSCT). Because most HLH patients are critically ill at the time of HLH diagnosis, timely introduction of immunosuppressive therapy is required before determining if a given patient has primary or secondary HLH. Molecular screening for primary HLH is usually performed in parallel with the treatment.
Initial therapy of HLH focuses on stopping the activation of CTLs and macrophages and on blocking excessive production of inflammatory cytokines. It is also important to search for and treat underlying infectious trigger of HLH development. Early use of γ-globulin and/or corticosteroid is sometimes useful for transiently controlling the HLH activity; however, an aggressive immunosuppressive therapy is warranted for most cases.
The first international treatment protocol for HLH (HLH-94) was organized by the Histiocyte Society in 1994 mainly aiming at treating primary HLH patients by HSCT. This protocol included an 8-week induction therapy with dexamethasone, etoposide, and intrathecal methotrexate, followed by maintenance therapy with pulse dexamethasone, etoposide, and cyclosporine A, which is intended as a bridging therapy to HSCT. The 5-year survival rate was 54 ± 6% in all patients, 66 ± 8% for those who received HSCT, and the outcomes of HSCT were better in patients with adequately controlled HLH activity before HSCT conditioning [59]. Genetic information was unavailable in HLH-94 study because the protocol started 5 years before the first FHL causative gene was reported. Intensification of induction therapy was indicated because most of the patients who did not receive HSCT and who died during the first year had active signs of HLH. Thus, the second international trial HLH-2004 was opened with major modification in induction therapy; cyclosporine A was used from the beginning and hydrocortisone was added to intrathecal therapy [2]. The result of the study is yet to be published, but cases of early death during the first 2-month period has been reduced. As a second line therapy for HLH refractory to etoposide-based therapy, Alemtuzumab, a monoclonal antibody to CD52, is reported to have induced partial response in 64% of the treated patients [60]. Beside etoposide-based regimens, an antithymocyte globulin (ATG) used in combination with corticosteroids as an initial treatment of primary HLH has been reported with comparable survival rate and acceptable toxicity [61].
For the most of patients with primary HLH, correction of genetic defects by HSCT is warranted after controlling the hyper-inflammatory state. Myeloablative conditioning (MAC) using busulfan, cyclophosphamide, and etoposide with or without ATG has long been the standard of HSCT for HLH patients [2]. However, MAC-based HSCT is associated with high treatment-related mortality [59, 62]. Recent reports suggest that reduced-intensity conditioning (RIC) can improve the outcome of HLH patients undergoing HSCT [63–65]. Still, a high incidence of mixed donor chimerism is associated with RIC-HSCT and long-term follow-up is needed to ascertain the outcome of the treatment.
The indication of HSCT for XLP type 2 depends on the clinical picture, because patients with XIAP deficiency were shown to have poor tolerance to MAC [66]. Recently reported patients survived RIST-HSCT with improvement of refractory inflammatory colitis [58, 67], and the control of posttransplant HLH with etoposide and corticosteroid seems to be an important factor for favorable outcomes [68]. The precise indication for HSCT remains also unclear for CHS patients because some patients have milder phenotype and survive into adulthood and because HSCT cannot prevent neurological dysfunction. Recent reports showed that the differences in the cytotoxic and degranulation capacity of CTLs may be predictive for the risk of HLH development [69]. Preemptive HSCT is not indicated for patients with HPS type 2 because it confers low risk of HLH [16].
12.7 Future Directions
Since the identification of first genetic defect for primary HLH in 1999, remarkable progress has been made in clinical practice and basic research. Detailed characterization of patients with primary HLH has contributed to our understanding of the granule-dependent cytotoxic pathway as well as pathophysiology of HLH. Aggressive immunosuppressive therapy followed by HSCT has greatly improved the prognosis of the patients; however, substantial risk of mortality remains. Novel therapeutic approaches with the use of ATG, Alemuzumab, and anti-IFN-γ monoclonal antibody are currently explored. Recently, PRF1 gene transfer into hematopoietic stem cells was shown to improve immune dysregulation observed in murine model of FHL2 [70]. It is possible that a gene therapy option may become available for primary HLH in the near future.
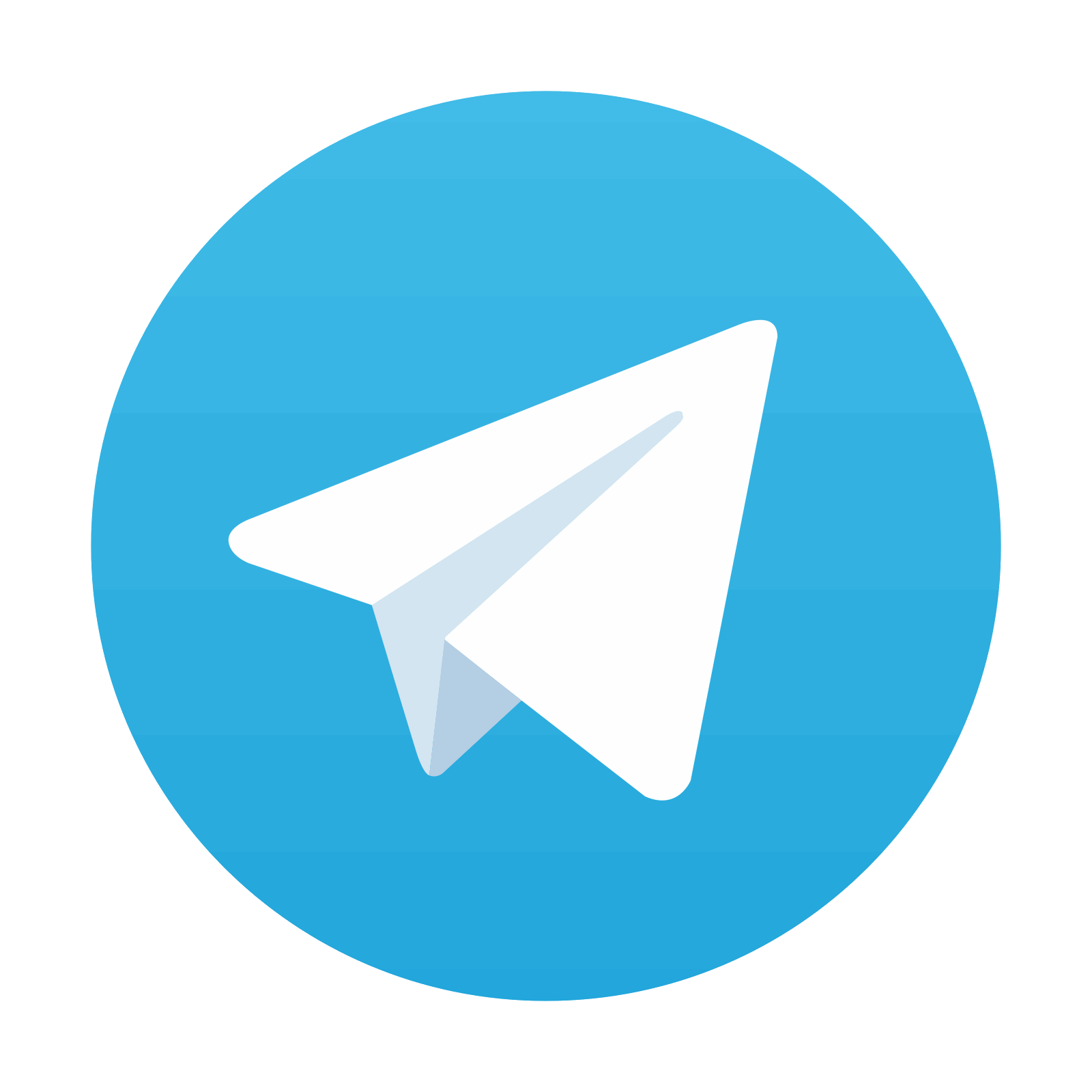
Stay updated, free articles. Join our Telegram channel

Full access? Get Clinical Tree
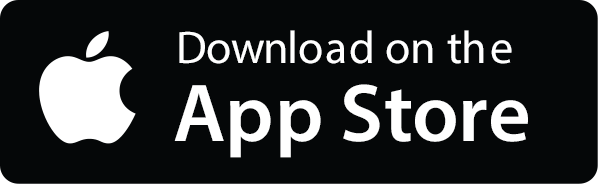
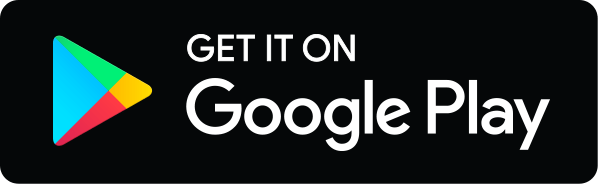