Introduction
Pregnant women constitute a uniquely challenging patient population as clinical decisions cannot be made solely on the basis of the woman’s needs but must also consider the impact of any therapeutic intervention on the developing fetus. As the health of the woman and that of her fetus are intertwined, any treatment designed to benefit one will inevitably affect the other. Further complicating matters is the fact that the initiation and maintenance of a healthy, viable pregnancy induces a wide variety of physiological changes that alter plasma hormone concentrations relative to the nonpregnant state. For the clinical laboratorian and care team, it is essential to understand these changes and interpret laboratory values in the context of the appropriate reference interval for the given stage of pregnancy. What is considered “normal” changes throughout pregnancy, particularly the concentrations of steroid and protein hormones measured in the evaluation of endocrine function. This chapter will describe typical endocrine function during pregnancy, highlight endocrine changes consistent with normal and abnormal pregnancy, and suggest strategies to apply clinical laboratory testing to guide patient care.
Feto-placental unit
Establishment and progression of a healthy, viable pregnancy require coordinated and synchronized contributions from the maternal, placental, and fetal compartments. Many of the changes in maternal serum hormone concentrations observed during pregnancy can be directly attributed to physiological alterations at the interface between mother and fetus, known as the feto-placental unit. These changes are required for the successful completion of key events that include embryo implantation, establishment of the placenta, continued support for fetal development, and ultimately, parturition.
Diagnosis and dating of pregnancy
Human pregnancy is defined as the approximately 40-week period from the first day of the last menstrual period to the date of delivery, or parturition. Pregnancy is subdivided into three trimesters of 13 weeks, with the first trimester defined as weeks 0–13 6/7, second trimester weeks 14 0/7–27 6/7, and third trimester weeks 28 0/7–40 6/7. As described in Chapter 5 , ovulation typically occurs on approximately day 14 of the menstrual cycle, which means the first 2 weeks of pregnancy actually occur prior to ovulation. Deliveries before 37 weeks are considered preterm, while early term describes pregnancies that last between 37 0/7 through 38 6/7 weeks, full term 39 0/7 through 40 6/7 weeks, late term 41 0/7 through 41 6/7 weeks and postterm 42 0/7 weeks, and beyond . Unless maternal or fetal complications necessitate early delivery, most medical centers will attempt to delay delivery until at least 37 weeks to avoid neonatal respiratory distress syndrome. Similarly, it is common practice at medical centers in the United States to induce delivery if labor has not begun spontaneously by the 42nd week as postterm deliveries are associated with an increased rate of stillbirth and neonatal death.
The mainstay of pregnancy diagnosis is the measurement of human chorionic gonadotropin (hCG) in urine or serum/plasma. hCG is produced by the trophoblast layer of the developing embryo and can be detected in culture medium as early as 2 days following fertilization using highly sensitive immunoassays . Once vascular communication is established between the fetus and maternal circulation, hCG can be detected in maternal urine and serum/plasma samples. Qualitative hCG urine testing can generate a positive result as early as the first missed menstrual period (approximately 2 weeks after conception), while quantitative testing in serum or plasma is substantially more sensitive. It has been reported that by 12 days post embryo transfer (approximately the first day of missed menses) the median serum hCG concentration is 120 IU/L (<1 mIU/mL nonpregnant females) . However, it is not known how well this median value translates to naturally occurring pregnancies. Following a positive urine or serum/plasma hCG result, transabdominal ultrasound may be performed to confirm pregnancy and estimate the date of conception by measuring fetal size.
Conception and implantation
Following fertilization, the embryo progresses through the fallopian tube and arrives in the uterus as a blastocyst consisting of 200–300 cells approximately 3–6 days after fertilization. This process of early embryonic differentiation appears to occur independently of external hormonal control as blastocysts can develop successfully in vitro. Relatively little is known about the hormone products secreted by the developing embryo during this process but it is thought that embryo-secreted progesterone may help facilitate passage from the fallopian tube to the uterine cavity . As described in Chapter 5 , Endocrine Disorders of the Reproductive System, progesterone produced by the corpus luteum increases vascularization of the endometrium, helping to induce a favorable environment for embryo implantation. The window of maximal endometrial receptivity occurs approximately 4–5 days after progesterone stimulation and remains open for 9–10 days . During this time, ordered expression of chemokines and removal of adhesion-inhibiting mucin encourage blastocyst attachment to particularly receptive areas of the endometrium. Local embryo-endometrium paracrine signaling further strengthens the initial attachment and invasive trophoblasts ultimately connect to maternal circulation through the endometrial epithelium. Embryonic hormones produced prior to implantation and biomarkers of endometrial receptivity are not routinely monitored in the clinical laboratory but progesterone is frequently measured to assess ovulation. Serum progesterone concentrations ≥5.0 ng/mL on or around day 21 of the menstrual cycle are consistent with ovulation .
Placenta
The placenta is the main interface between the developing fetus and the mother. It constitutes a selective barrier that allows for the exchange of nutrients and fetal waste products and carries out numerous functions that support and maintain pregnancy and the fetus. To accomplish this, the placenta is a major site of hormone production throughout gestation and its hormone products include proteins normally produced in the pituitary or hypothalamus as well as steroid hormones produced in the adult ovaries.
The placental cytotrophoblast and syncytiotrophoblast cell layers take on the roles of the hypothalamus and pituitary, with the hypothalamic hormones produced by the cytotrophoblasts and pituitary hormones produced by the syncytiotrophoblasts. The concentrations of hormones produced by the placenta change throughout gestation, as the role of the placenta matures from its early invasive stage to one that supports the nutritional needs of the fetus.
Placental hormones: pituitary
Human chorionic gonadotropin
The defining hormone of pregnancy is hCG. hCG is a glycoprotein hormone composed of noncovalently associated dissimilar alpha (α) and beta (β) subunits. As a member of the pituitary glycoprotein family, the α subunit is shared between hCG and the other three members [thyroid-stimulating hormone (TSH), follicle-stimulating hormone (FSH), and luteinizing hormone (LH)], while the beta subunit confers biological specificity.
In addition to intact hCG, well-characterized hCG variants circulate during pregnancy and include nicked intact hCG (hCGn), the free β-subunit (hCGβ), nicked free β-subunit (hCGβn), and the hCG β-core fragment (hCGβcf) . Only the intact hCG dimer retains hormonal activity and is the predominant circulating form in plasma after the fourth to fifth week of pregnancy . A differentially glycosylated intact hCG, termed hyperglycosylated hCG (hCG-H), predominates in the earliest stages of pregnancy and is correlated with the proliferation of the syncytiotrophoblastic cell layer’s invasion into the endometrium ( Table 15.1 ).
Isoform | Description | Relative contribution to total hCG |
---|---|---|
Intact hCG | Alpha/beta heterodimer with eight linked carbohydrate chains | Principal form in serum weeks 6–40 |
Nicked hCG | Partially degraded intact hCG, missing peptide bonds in hCG beta 40–50 region | Constitutes ~10% of total serum hCG |
Free beta subunit | Complete beta subunit dissociated from alpha subunit | Constitutes ~1% of total serum hCG |
Nicked free beta subunit | Partially degraded free beta subunit, missing peptide bonds in hCG beta 40–50 region | Constitutes <1% of total serum hCG |
Hyperglycosylated hCG | Alpha/beta heterodimer with larger and more complex oligosaccharides relative to intact hCG | Principal form in serum during early pregnancy (weeks 3–5) |
hCG beta core fragment | Degraded free beta subunit consisting only of hCG beta 6–40 and 55–92 joined by disulfide bonds | Principal form in urine weeks 6–40, not present in serum |
hCG’s purpose in early pregnancy is to maintain the corpus luteum and its production of progesterone. Until approximately the eighth week of gestation, placental production of progesterone is insufficient to prevent menstruation and pregnancy loss, and the main site of progesterone production is the corpus luteum. Early failure of the luteal body would likely result in insufficient production of progesterone and pregnancy loss.
In uncomplicated pregnancies where the embryo successfully implants into the endometrial lining of the uterus, hCG concentrations rise quickly with a doubling time of approximately 2 days. By the fifth week of gestation the doubling time increases slightly to between 2 and 3 days. Peak hCG concentrations are reached by the eighth or ninth week of gestation and decrease by 90% of peak concentrations by the end of pregnancy ( Fig. 15.1 ). The rapid increase in hCG concentrations is indicative of the rapid growth of the placenta in early gestation.
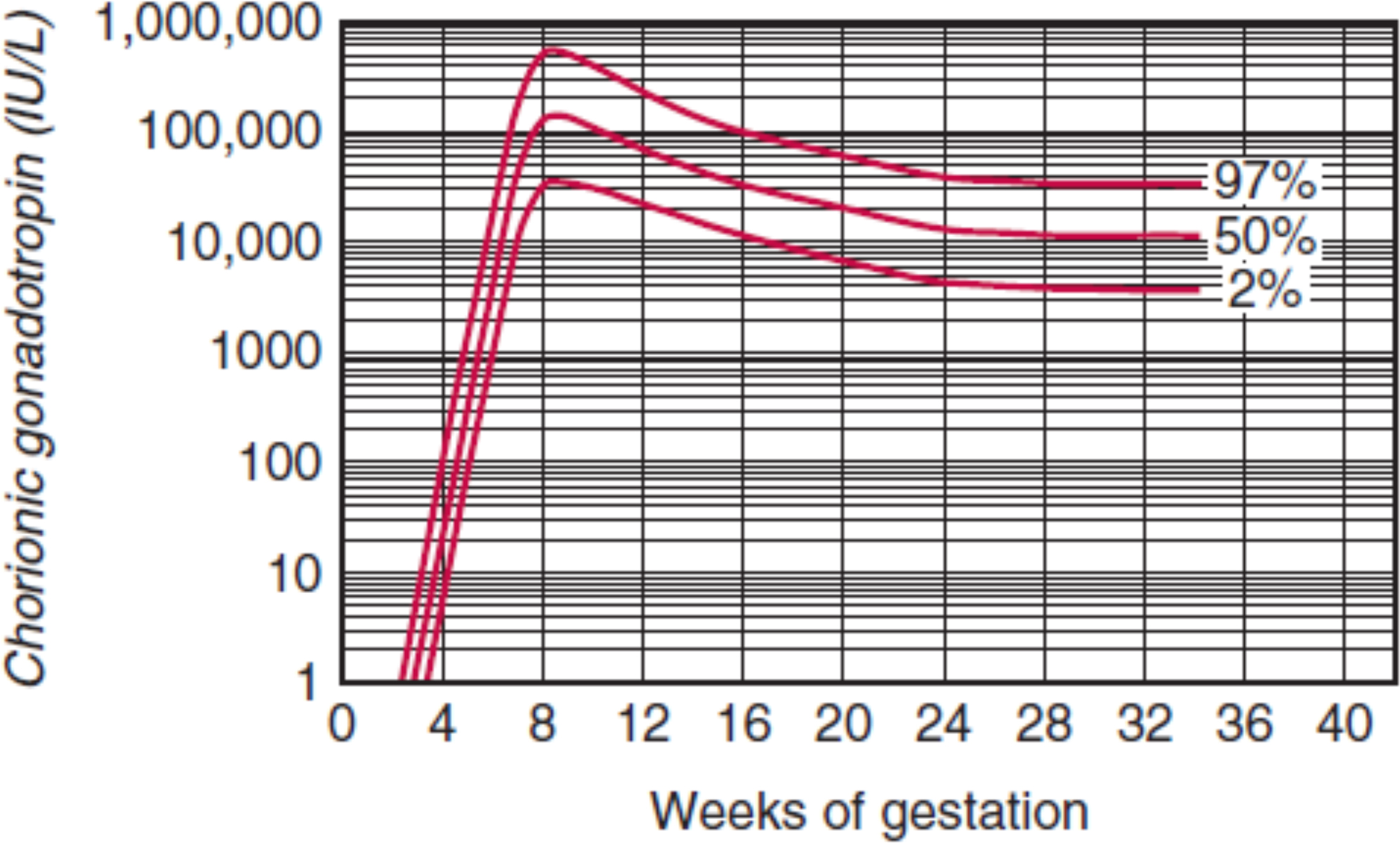
Although hCG is typically thought of as “the pregnancy hormone,” nonplacental hCG may be encountered in postmenopausal women or free beta subunit inpatients with a germ cell tumor. hCG is also produced in nonviable pregnancies, including ectopic pregnancy, molar pregnancy, and choriocarcinoma. A longer than expected doubling time may indicate ectopic pregnancy or spontaneous abortion, while a shorter than expected doubling time is indicative of choriocarcinoma.
Placental growth hormone
The syncytiotrophoblasts and extravillous cytotrophoblast layers of the placenta produce placental growth hormone (GH) beginning at about 5–7 weeks of gestation. Placental GH concentrations continue to rise throughout pregnancy and surpass maternal pituitary GH concentrations between weeks 15 and 20 . Placental and pituitary GH are structurally related. Both are 191 amino acids long, differ by only 13 amino acids and bind maternal GH receptors, promoting maternal expression of insulin-like growth factor I (IGF-I). In contrast to the pulsatile release of pituitary GH, placental GH is continuously released, with concentrations inversely correlated with maternal glucose and insulin concentrations.
Placental GH’s primary role is the promotion of maternal lipolysis and gluconeogenesis to increase nutrient availability for fetal growth. Placental GH mediates these actions by stimulating the production of IGF-I and IGF-II in a similar manner as pituitary GH. While the predominant targets of placental GH are maternal, GH receptors are also found on syncytiotrophoblasts indicating an autocrine/paracrine role for the hormone. Placental GH is also found in amniotic fluid; however, its role in direct fetal growth is as yet unknown.
Human placental lactogen
Human placental lactogen (hPL) is a member of the same gene family as pituitary and placental GH and shares 85% homology with pituitary GH. As with placental GH, hPL is expressed by the syncytiotrophoblasts and is similarly detected in maternal circulation by 5–7 weeks . hPL concentrations increase consistently throughout gestation, reaching peak concentrations of between 5000 and 7000 ng/mL at term.
The target of hPL appears to be predominantly the prolactin receptor, and while hPL concentrations are positively correlated with placental GH and IGF-I, hPL binds only weakly to GH receptors (2300-fold lower affinity than placental GH). hPL has been implicated in expansion of pancreatic beta-cell number and the increased maternal serum insulin concentrations observed during the third trimester . hPL is also found in the fetus, however, at much lower concentrations (20–50 ng/mL). It appears to antagonize the effects of insulin but its role in fetal development is as yet not fully elucidated.
Placental adrenocorticotropic hormone
Adrenocorticotropic hormone (ACTH) is produced by the syncytiotrophoblast cell layer of the placenta under the control of placentally produced corticotropin releasing hormone (CRH) in an autocrine/paracrine manner . ACTH concentrations increase throughout pregnancy, but contradictory data exist as to whether the concentrations are greater than or less than those of nonpregnant women. This variability could be due to differential assay recognition of proopiomelanocortin, which is also produced and secreted by the placenta .
Regulation of placental ACTH expression is fundamentally different from the regulation of pituitary ACTH, as glucocorticoids increase ACTH expression through upregulation of CRH . This positive feedback mechanism increases cortisol secretion from the maternal adrenals during pregnancy.
Placental hormones: hypothalamus
Gonadotropin-releasing hormone
Gonadotropin-releasing hormone (GnRH, LHRH, FSHRH) is a decapeptide hormone produced by the hypothalamus and also by the cytotrophoblast cell layer of the placenta . Placental GnRH stimulates hCG production by the syncytiotrophoblasts via a paracrine mechanism, and plasma GnRH concentrations parallel the growth of the cytotrophoblast cell layer as well as the rise of hCG concentrations, peaking around week 8–9 of gestation . Additional lines of evidence suggest that GnRH may mediate placental invasiveness through other signaling mechanisms but further discussion is beyond the scope of this chapter.
Corticotropin-releasing hormone
CRH produced in the cytotrophoblast cell layer of the placenta is identical to that of the hypothalamic hormone and carries out similar functions in the placenta. Maternal plasma CRH concentrations increase throughout gestation, rising substantially between the second and third trimesters. CRH is secreted into both maternal and fetal circulation and has been demonstrated to stimulate the release of ACTH from placental syncytiotrophoblasts . In the fetus, CRH is assumed to stimulate ACTH release and subsequent increases in fetal glucocorticoids as the increasing placental CRH expression correlates with increasing fetal concentrations of glucocorticoids late in gestation that promote fetal organ development and lung maturation. However, this role is complicated by the knowledge that the fetal hypothalamus is also able to produce CRH.
Thyrotropin-releasing hormone
Thyrotropin-releasing hormone (TRH) is produced in both the cytotrophoblast and syncytiotrophoblast layers of the placenta and is released into both maternal and fetal circulation . The majority of placental TRH is secreted into fetal rather than maternal circulation, where it likely stimulates the production of TSH.
Other placental hormones
Pregnancy-associated plasma protein A
Pregnancy-associated plasma protein A (PAPP-A) is a zinc-containing metalloproteinase produced by the syncytiotrophoblasts whose concentrations increase rapidly in early pregnancy with a doubling time of 3 days before slowing to a more gradual increase until term. PAPP-A regulates the concentration of free IGF-II by cleaving insulin-like growth factor binding proteins (IGFBP), predominantly IGFBP-4 . PAPP-A circulates as a large heterotetramer composed of two subunits of PAPP-A and two subunits of pro-major basic binding protein, which is produced by the cytotrophoblast cell layer of the placenta. PAPP-A measurements are performed exclusively as part of first trimester aneuploidy screening. A more detailed discussion of maternal serum screening is included later in this chapter.
Insulin-like growth factors I and II
IGF-I and IGF-II are stimulated by GH but IGF-I is produced by both syncytiotrophoblasts and cytotrophoblasts, whereas IGF-II is produced by cytotrophoblasts only. IGF-I concentrations in maternal serum initially decrease during the first trimester, but steadily increase during the second and third trimesters with mean concentrations exceeding those found in early gestation ( Fig. 15.2 ) . There are conflicting data as to whether IGF-I concentrations are associated with newborn birth weight.
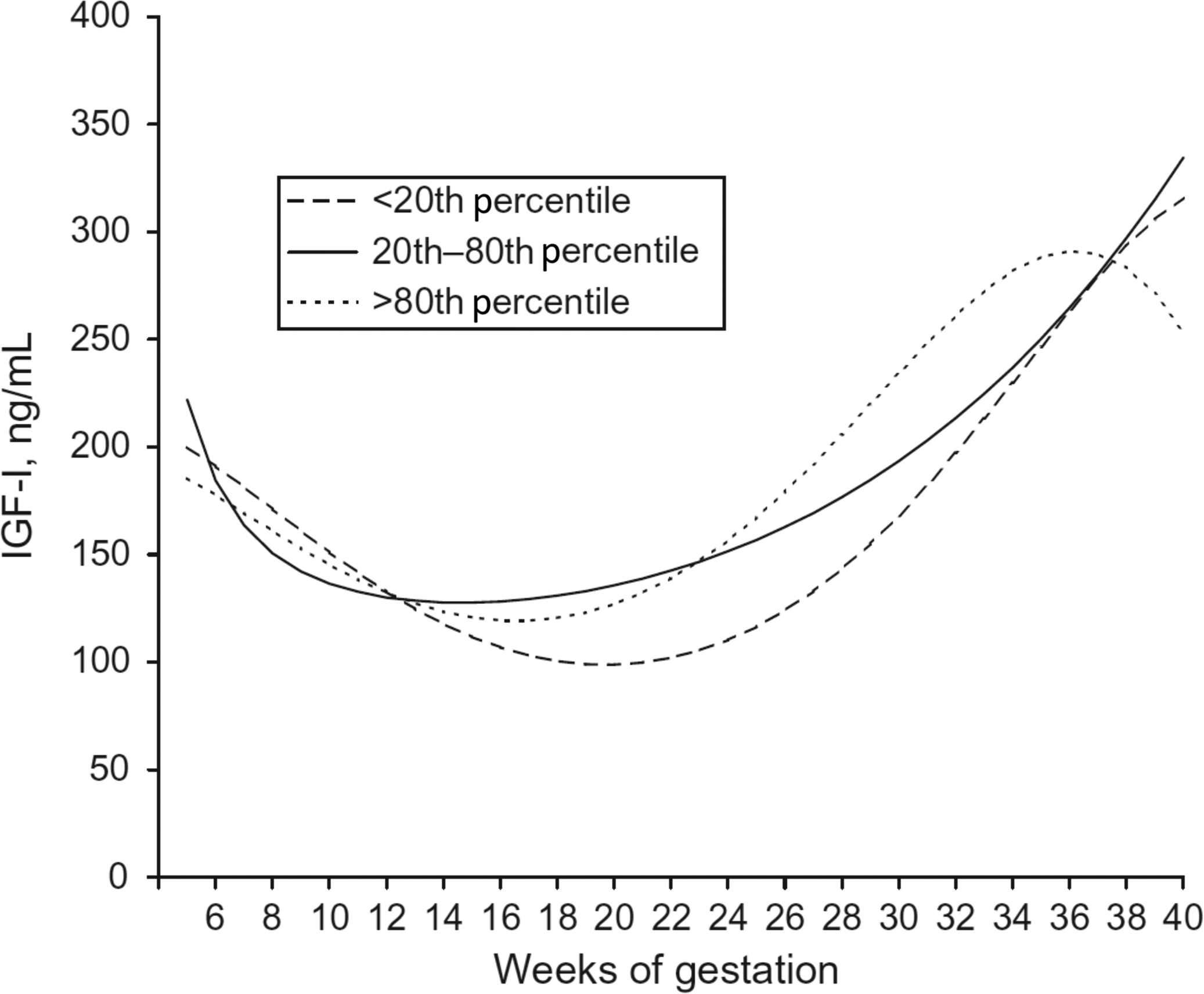
In contrast to IGF-I, whose concentrations do not greatly exceed those of nonpregnant women, IGF-II concentrations in pregnant women are markedly elevated. At term, the concentration of IGF-II is approximately sixfold the concentrations of IGF-I in cord blood . IGF-II plays an important role in regulating fetal growth, as shown by Beckwith–Wiedemann Syndrome (biallelic expression, large for gestational age) and Russell–Silver Syndrome (biallelic loss, small for gestational age), two conditions characterized by abnormal methylation of the IGF-II promoter .
It is worth noting that placental IGF-I and IGF-II are secreted into maternal circulation, not fetal. The concentrations of IGF-I and IGF-II during pregnancy are thought to influence fetal size via an indirect effect on placental size and nutrient delivery to the fetus.
Angiogenic factors
The placenta is heavily vascularized and in early gestation is characterized by vigorous angiogenesis, during which time the placenta invades the endometrial lining. During later gestation, there is continued remodeling of the decidual spiral arteries. The regulation of angiogenesis and remodeling is complex, and dysregulation during pregnancy can lead to preeclampsia. Placental growth factor (PlGF), a member of the vascular endothelial growth factor (VEGF) family, is expressed throughout pregnancy by the villous trophoblasts and is suggested to have a role in early placental vascularization and invasiveness. Soluble Fms-like tyrosine kinase (sFlt-1) is a splice variant of the VEGF receptor Flt-1 that lacks anchoring transmembrane and intracellular domains, but retains VEGF binding ability. sFlt-1 is secreted from the placenta into maternal circulation and binds VEGF, preventing its binding to membrane bound Flt-1. Disruption of VEGF signaling favors continued support of the placenta at maternal expense. sFlt-1 concentrations are increased by placental stress and increased concentrations have been shown to precede the clinical symptoms of preeclampsia (see further discussion later in chapter).
Inhibin and activin
The inhibins and activins are hetero- or homodimeric glycoprotein hormones composed of either an α and β chain (inhibin) or two β chains (activin). There are two separate β chains (β A and β B ), giving the possible combinations of inhibin A (α-β A ), inhibin B (α-β B ), activin A (β A -β A ), activin AB (β A -β B ), and activin B (β B -β B ). Commercial assays are available for the measurement of inhibin A and B as tumor markers used to monitor ovarian granulosa cell tumors. No commercial assays are available for activin measurement, as it has limited clinical utility.
The inhibins and activins are secreted into maternal circulation by the syncytiotrophoblasts and have also been localized to the cytotrophoblast layer. Early in the first trimester, the majority of inhibin A and activin A are produced by the corpus luteum with a transition to placental production occurring around 12 weeks of gestation . The concentrations of inhibin A and activin A increase throughout gestation, reaching their highest concentration at term. In contrast, inhibin B does not significantly increase relative to nonpregnant concentrations. Further discussion on dimeric inhibin A (DIA) and aneuploidy screening is included later in this chapter.
Placental steroid hormones
Progesterone
As mentioned earlier in this chapter, progesterone serves an important role in pregnancy by promoting the maintenance of the decidua and prevention of menstruation. Progesterone has been demonstrated to mediate maternal immune tolerance and prevent uterine contractions. Progesterone also stimulates growth of mammary tissue necessary for lactation and prevents onset of lactation by opposing the effects of prolactin. Following labor and delivery, progesterone concentrations rapidly decrease, allowing for the effects of prolactin to prevail.
Prior to the luteal-placental transition, the majority of progesterone in early gestation is derived from the corpus luteum . In early gestation, progesterone concentrations are maintained near those observed in the luteal phase (~20 ng/mL) but continue to rise through pregnancy to a peak at term that is approximately 10-fold that of the luteal phase .
Estrogens
Similar to progesterone, the corpus luteum is the primary source of estrogens in early pregnancy. Placental 17β-estradiol is the primary estrogen throughout pregnancy and the concentration of all the estrogens [estrone (E 1 ), 17β-estradiol (E 2 ), and estriol (E 3 )] increase throughout gestation, peaking at term. Unconjugated estriol concentrations are lower in pregnancies affected by Down Syndrome, as discussed later in this chapter.
Unlike estrogen synthesis from ovarian tissues, placental estrogens are not derived from pregnenolone and progesterone, as the placenta lacks the necessary 17α-hydroxylase enzyme. Instead, placental estrogens are synthesized from dehydroepiandrosterone sulfate (DHEA-S) originating from the maternal and fetal adrenals. To produce E 2 , DHEA-S is first converted to androstenedione by 3βHSD, then to testosterone by 17β-hydroxysteroid dehydrogenase, and then finally to E 2 by aromatase (CYP21A2). Androstenedione can also be directly converted to E 1 by aromatase ( Fig. 15.3 ).
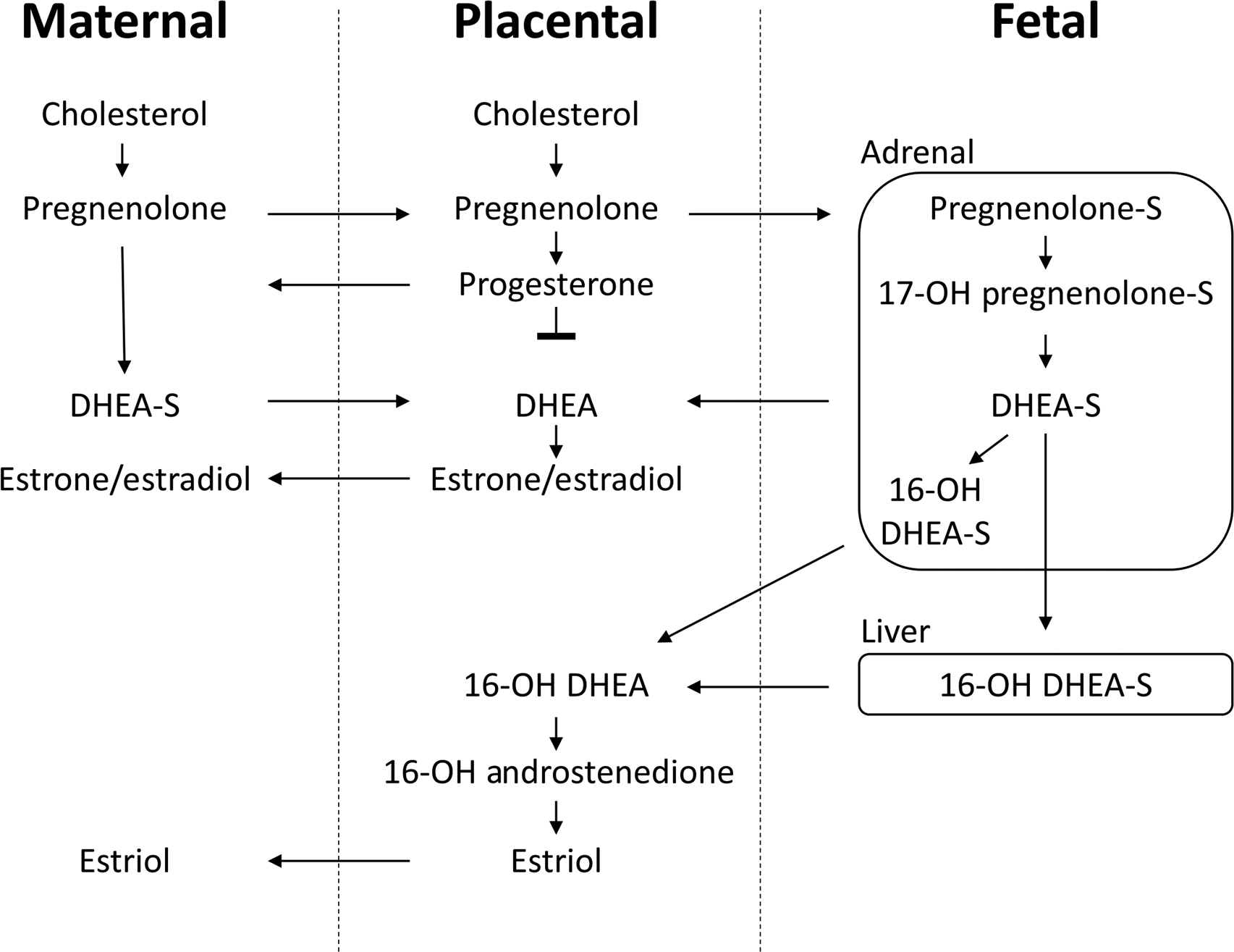
Placental E 3 production is solely reliant upon 16α-hydroxy-DHEA-S from DHEA-S in the fetal liver, as the placenta lacks the necessary 16α-hydroxylase enzyme. Fetal 16α-hydroxy-DHEA-S is transported to the placenta, where it is converted to E 3 by aromatase.
Of the three estrogens, E 2 is the most active; however, all of the estrogens promote vasodilation and angiogenesis and thus play an important role in the placental vascularization. The estrogens are also involved in the proliferation of mammary tissues in preparation for lactation.
Fetal endocrine function
Hypothalamus and pituitary
The hypothalamus/posterior pituitary and anterior pituitary are derived from separate tissues that grow together and fuse to form the highly interconnected structures . The hypothalamus, pituitary, and the portal circulation that connects them begins development in the first trimester with completion of the portal circulation by the 18th week of gestation.
The hypothalamic hormones CRH, growth-hormone releasing hormone (GHRH), GnRH, and TRH are detectable in the fetal hypothalamus by approximately the 14th–15th week of gestation .
The fetal pituitary is capable of producing ACTH, LH, FSH, TSH, and prolactin by approximately the 18th week of gestation.
Fetal thyroid
The fetal thyroid develops in the first trimester, is able to synthesize thyroxine by the 10 – 12th week of gestation and increases thyroxine production from midgestation to term. While the fetal thyroid is an important source of thyroxine, there is also transplacental transfer of thyroid hormones from maternal circulation. Supporting a role for maternal thyroxine, children affected by congenital hypothyroidism do not initially demonstrate symptoms. However, rapid recognition and treatment of congenital hypothyroidism immediately following birth are essential and have been correlated with higher intelligence . Given the importance of early detection and treatment of congenital hypothyroidism, TSH and/or total T4 are routinely measured as part of newborn screening in developed countries .
Fetal gonads
The fetal gonads are derived from a primordial germ cell pool and differentiate into morphologically distinct testes and ovaries early in gestation, between 6 and 8 weeks. The fetal testes produce two hormones that induce male sexual differentiation: testosterone and anti-Müllerian hormone (AMH). Testosterone is produced by the fetal Leydig cells and induces male sexual differentiation. Disruption of testosterone production or its interaction with the androgen receptor can result in ambiguous or female external genitalia. AMH is produced by testicular Sertoli cells and induces regression of the Müllerian ducts, which would otherwise develop into the uterus and fallopian tubes ( Fig. 15.4 ). Disruption of AMH secretion results in persistent Müllerian duct syndrome, in which the patients are phenotypically male (normally virilized) with no abnormalities to the external genitalia, but have bilateral or unilateral cryptorchidism and have a uterus and fallopian tubes .
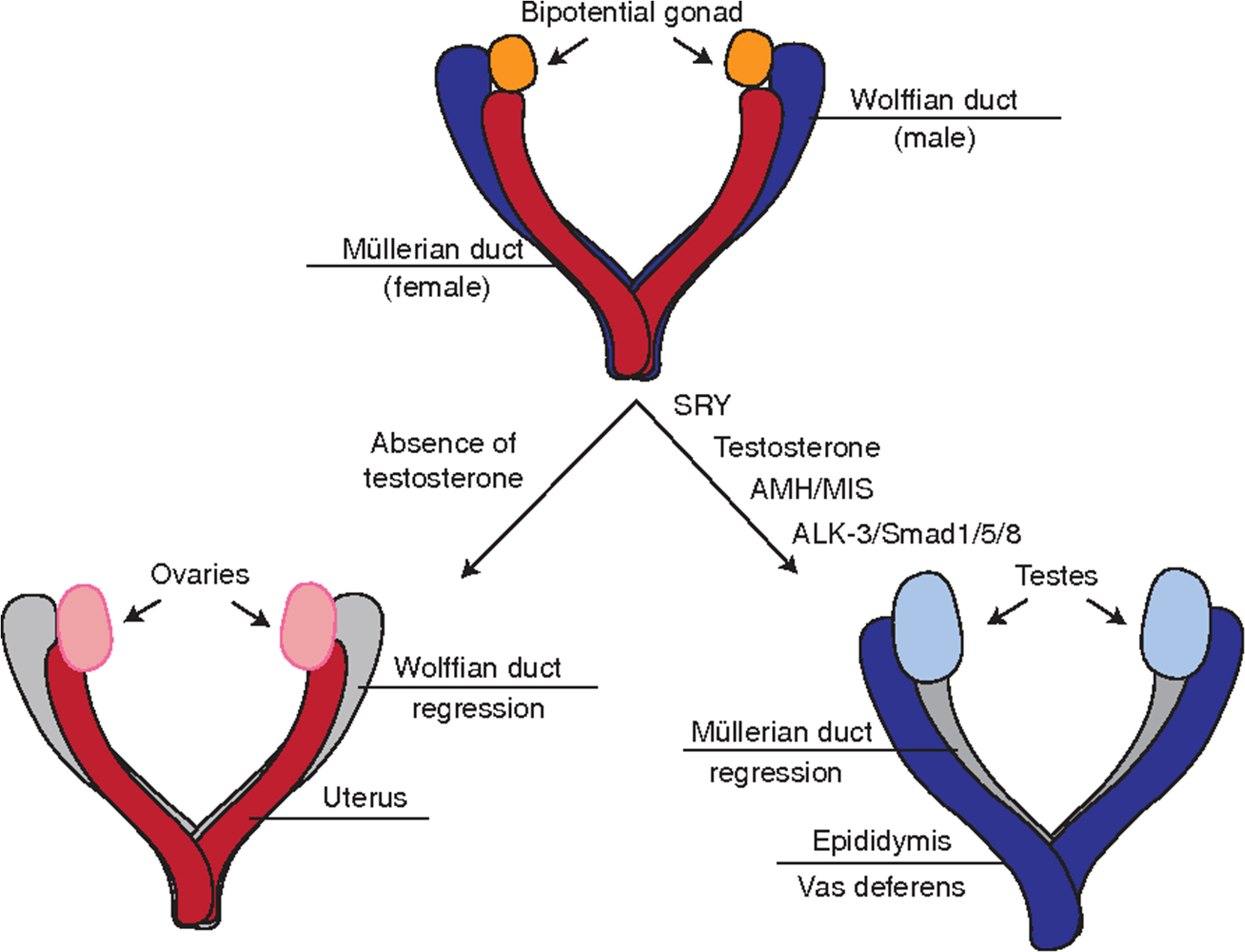
Fetal adrenal glands
The fetal adrenal undergoes impressive changes both during gestation and again after birth. While in utero the fetal adrenal grows to approximately the same size as adult adrenal glands. The fetal adrenal, however, differs in structure and function from the adult adrenals. The zones of the fetal adrenal from the innermost to outer most are the medulla, fetal zone, and definitive zone ( Fig. 15.5 ).
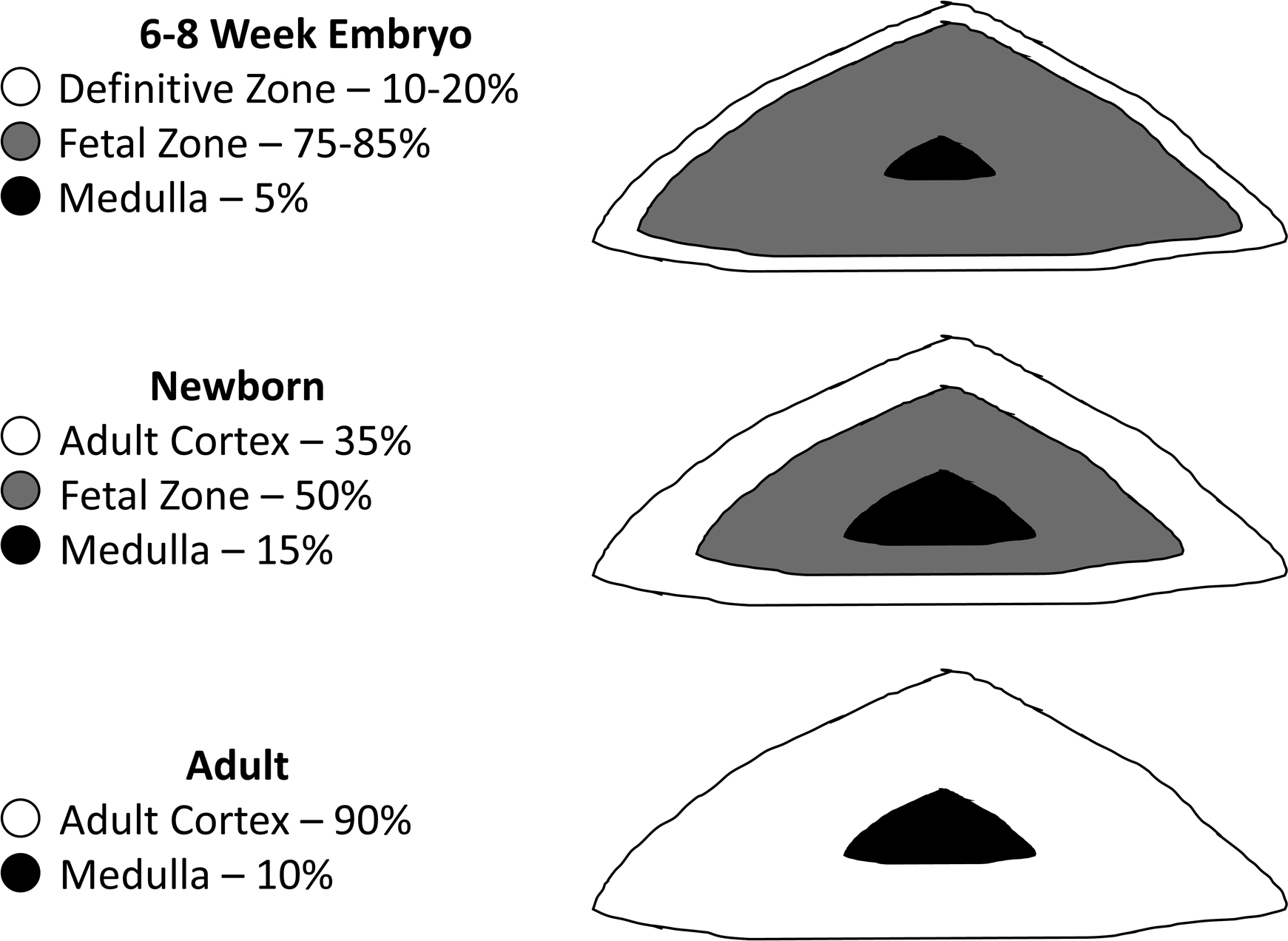
The fetal zone occupies approximately 80%–85% of the total fetal adrenal volume and this zone rapidly regresses with the fetal adrenal shrinking by 50% within the first 2 weeks of term birth . The fetal zone produces large quantities of DHEA-S from a starting pool of cholesterol but cannot process the DHEA-S to androstenedione due to a lack of 3β-hydroxysteroid dehydrogenase. Instead, the DHEA-S produced by the fetal adrenal is an important substrate for the placental production of E2 and E3 as described previously.
The growth of the fetal adrenal and production of DHEA-S and cortisol by the fetal adrenal are mediated in part by fetal production of ACTH. Before 10 weeks of gestation, growth of the fetal adrenal is independent of ACTH, as the adrenals of anencephalic and unaffected fetuses are similar in size. However, in later gestation the adrenals of anencephalic fetuses are markedly smaller than those unaffected by anencephaly.
Fetal ACTH and cortisol concentrations demonstrate the ACTH paradox, as fetal pituitary ACTH production is highest between 10 and 20 weeks of gestation but fetal cortisol production peaks toward the end of term. Increased sensitivity of the definitive zone to ACTH has been proposed as an explanation for the paradoxical increase in cortisol production when fetal ACTH concentrations are not at their peak. This increase in sensitivity of the fetal adrenal to ACTH may be moderated in part by CRH, which has been shown to increase ACTH receptor expression in definitive zone cells. There is also evidence in an in vitro system that CRH can directly stimulate cortisol and DHEA-S expression by fetal adrenal cells .
Maternal endocrine function
Hypothalamus and pituitary
Increasing concentrations of estrogen during pregnancy alter the secretion of pituitary hormones, as FSH and LH are suppressed, while prolactin secretion increases up to 10-fold . The pituitary exhibits a corresponding increase in size, increasing in volume by 45%, most of which can be attributed to the anterior pituitary . Growth is primarily driven by increasing numbers and size of lactotroph cells, while other pituitary cells are either unaffected or diminished. Serum prolactin concentrations parallel pituitary size through pregnancy. As discussed earlier, placental CRH drives increased ACTH production by the maternal pituitary.
The pituitary peaks in size in the immediate postpartum period and returns to prepregnancy size by about 6 months postpartum. Estrogen elevations during pregnancy also result in pituitary hyperemia, which places the pituitary at risk of infarction due to hypovolemic shock when delivery is accompanied by massive hemorrhage. The resulting scarring of the pituitary can result in postpartum hypopituitarism known as Sheehan syndrome, which is rare (1 in 10,000 pregnancies or fewer). In addition, when the pituitary hypertrophy of pregnancy occurs in the setting of a pituitary adenoma, it can (rarely) result in pituitary apoplexy, which is potentially life-threatening to both mother and child.
Parathyroid glands
Parathyroid hormone (PTH) increases during pregnancy by approximately 40%, but ionized calcium remains unchanged . The increase in PTH results in an increase in 1,25 dihydroxyvitamin D, which causes increased calcium absorption to support fetal skeletal development.
Thyroid
Maternal thyroid disease during pregnancy, especially during the first trimester, can have important consequences for both the mother and the fetus. Thyroid hormone is critically important in the neurological development of the fetus; however, the fetus is dependent on maternal T4 until the fetal thyroid becomes capable of T4 synthesis and iodine-concentrating activity around the 12th week of gestation . Therefore identification of women with thyroid disease (particularly hypothyroidism) is of critical importance.
Normal thyroid function in pregnancy
While pregnancy is in principle a euthyroid state, it does result in alterations to the maternal thyroid system, whose measured components should be interpreted in the context of pregnancy-specific reference intervals.
During pregnancy, increased estradiol production stimulates increased sialylation of thyroxine-binding globulin (TBG), prolonging its half-life, and may stimulate increased production of TBG in the liver. The net result is increasing TBG concentrations throughout pregnancy. To maintain consistent free T4 (FT4) pools, the total T4 concentration increases in parallel with TBG.
Logarithmic increases in hCG during the first trimester sufficiently stimulate TSH receptors on thyroid follicular cells to cause a transient increase in production of T3 and T4. This results in a mild suppression of TSH until hCG concentrations begin to decline around week 10–12, at which time TSH returns to prepregnancy concentrations ( Fig. 15.6 ). FT4 studies performed by reference methods have shown small declines in FT4 at the end of pregnancy . The thyroid increases in size by about 18% during pregnancy, possibly due to the thyrotropic effects of hCG in the first trimester and increased production of T4 to maintain FT4 concentrations despite increased TBG concentrations.
