Introduction
The interaction of physical activity, exercise and physical fitness with health and ageing is complex and multifaceted. Although many questions remain about mechanisms of effect and dose–response curves, a synthesis of the literature indicates many potentially positive effects of participation in physical activity on the ageing process. Most recent position stands and policy recommendations include physical activity prescriptions for health promotion and disease prevention and also chronic disease treatment in older adults. However, there is still scepticism among some clinicians and investigators as to the actual potency of exercise for disease and/or disability prevention and treatment, particularly in already frail or near frail adults. Exercise has not become fully integrated into usual geriatric medicine practice and is still virtually absent from the core training of most geriatric medicine physicians and healthcare professionals. Therefore, this chapter attempts to provide a rationale for the use of exercise and physical activity for health promotion and disease prevention in older adults. Exercise is discussed in terms of the specific modalities and doses that have been studied in randomized controlled trials for their role in the physiological changes of ageing, disease prevention and treatment of older persons with chronic disease and disability. Recommendations will be offered to address gaps in knowledge and also clinical implementation needs in this field.
What is Exercise?
Any discussion of these issues must begin with definitions of the terminology. Physical activity has traditionally been defined as any bodily movement produced by contraction of skeletal muscle that significantly increases energy expenditure, although the intensity and duration can vary substantially. It should be noted that some forms of physical activity which may have particular relevance to an ageing population (e.g. balance training) may not conform to this standard definition. This activity may be performed in leisure or occupational hours and surveys for the older adult should capture both paid and unpaid (volunteer) work. Exercise is a subcategory of leisure time physical activity in which planned, structured, repetitive bodily movements are performed, with or without the explicit intent of improving one or more components of physical fitness.
Recently, efforts have been focused on merging these formerly distinct entities in order to promote ‘lifestyle integration’ of exercise as a means to enhance long-term adherence. For example, taking the stairs instead of the elevator, standing on one leg while doing the dishes or slowly standing and sitting without use of the arms represent ways of incorporating aerobic, balance and strengthening exercises, respectively, into everyday activities. Current investigations are exploring whether such prescriptive techniques are superior to standard approaches for the promotion of behavioural change in older adults.
Physical fitness, by contrast, is defined as a set of attributes that contribute to the ability to perform physical work (e.g. cardiorespiratory endurance, muscle power, balance, flexibility and body composition) or influence health status. ‘Metabolic fitness’ has been advanced more recently as a term that encompasses a range of biologically important traits (increased insulin sensitivity, lipoprotein lipase activity, endothelial cell reactivity, heart rate variability, etc.) which may contribute to health status, but do not directly affect exercise capacity. Both genetic predisposition and lifestyle factors contribute to physical and metabolic fitness and the extent to which they are modifiable with exercise training.
Dose–response relationships between changes in fitness and better health outcomes have been defined for some, but certainly not for all, diseases and syndromes. Some modalities or doses of exercise that are promoted for older adults (mild calisthenics, slow-paced walking) have little or no discernible effects on physical fitness, but may possibly yield benefits in some domains. This area of investigation is critical for defining threshold and optimal levels of activity that are necessary for health promotion and disease management. It should be recognized that what is suitable for prevention may be entirely inadequate for treatment, as is also the case with pharmacological management of chronic diseases. For example, aspirin may reduce the risk of ischaemic heart disease, but a host of potent agents may be required once coronary occlusive disease is present and symptomatic.
Does Exercise Increase Life Expectancy?
The effects of exercise on total mortality are unlikely ever to be substantiated via randomized controlled clinical trials, given the impossibility of random assignment to various physical activity regimens over many decades. However, there is clear evidence of an inverse, linear dose–response relationship between the volume of physical activity reported in epidemiological studies (with sample sizes ranging from less than 500 to over 2.5 million individuals) and all-cause mortality rates. These relationships are demonstrable for both men and women and for both older and younger adults. Volumes of energy expenditure during exercise of at least 1000 kcal per week reduce mortality by about 30%, whereas reductions of 50% or more are seen with volumes closer to 2000 kcal per week, when more precise measures or estimates of physical activity participation incorporating fitness assessments are utilized instead of surveys. These changes in all-cause and cardiovascular mortality translate to an increase in life expectancy of ∼2 years for those exercising at such volumes. Despite the consistency of the data from well-designed observational studies, many questions still remain regarding the minimum threshold for efficacy, the effect of exercise intensity, duration and frequency (apart from contributions to overall volume), the effect of non-aerobic modalities of exercise and the mechanism of benefit. From a public health perspective, if small, effective doses of moderate-intensity activity are found to be as beneficial as longer bouts of vigorous activity, adoption of mortality-reducing physical activity recommendations by sedentary middle-aged and older adults may be more successful. Of particular relevance to the geriatric exercise prescription are studies which have demonstrated that a change from a sedentary to more active lifestyle in midlife or beyond is associated with a reduction in mortality. In the sections that follow, the focus is on changes in physical fitness and body composition, quality of life and disease burden, rather than on changes in longevity itself. It is in these domains that the centrality of physical activity patterns to optimal ageing is perhaps most relevant to the concerns of the healthcare professional and the older individual.
Preserving Exercise Capacity with Age via An Active Lifestyle
There is a great similarity between the physiological changes attributable to disuse and those which have been typically observed in ageing populations, leading to the speculation that the way in which we age may be modulated with attention to activity levels.1 The most important physiological changes associated with ageing or disuse that impact upon exercise capacity are presented in Tables 10.1–10.4. In most physiological systems, the normal ageing processes do not result in significant impairment or dysfunction in the absence of pathology and under resting conditions. However, in response to a stress or significant disuse, the age-related reduction in physiological reserves (‘homeostenosis’) may result in difficulty in completing a task requiring near-maximum effort.
Table 10.1 Changes in exercise capacity due to ageing or disuse potentially modifiable by physical activity.
Component of exercise capacity | Effect of ageing or disuse |
Maximum aerobic capacity | Decrease |
Tissue elasticity | Decrease |
Muscle strength, power, endurance, mass | Decrease |
Oxidative and glycolytic enzyme capacity, mitochondrial volume density | Decrease |
Gait speed, step length, cadence, gait stability | Decrease |
Table 10.2 Changes in cardiorespiratory function due to ageing or disuse potentially modifiable by physical activity.
Cardiorespiratory function | Effect of ageing or disuse |
Heart rate and blood pressure response to submaximum exercise | Increase |
Maximum heart ratea | Decrease |
Resting heart rate | No change |
Maximum cardiac output, stroke volume | Decrease |
Endothelial cell reactivity | Decrease |
Heart rate variability | Decrease |
Maximum skeletal muscle blood flow | Decrease |
Capillary density | Decrease |
Arterial distensibility | Decrease |
Vascular insulin sensitivity | Decrease |
Plasma volume, haematocrit | No change, decrease |
Postural hypotension in response to stressors | Increase |
Total lung capacity, vital capacitya | Decrease |
Maximum pulmonary flow ratesa | Decrease |
aNo evidence yet that exercise can prevent or reverse these changes of ageing.
Table 10.3 Changes in metabolism and body composition due to ageing or disuse potentially modifiable by physical activity.
Metabolic/body composition change | Effect of ageing or disuse |
Resting metabolic rate | Decrease |
Total energy expenditure | Decrease |
Thermic effect of meals | Decrease, no change |
Total body water | Decrease |
Total body potassium, nitrogen, calcium | Decrease |
Protein synthesis rate, amino acid uptake into skeletal muscle, nitrogen retention, protein turnover | Decrease |
Gastrointestinal transit time | Increase |
Appetite, energy intake | Decrease, no change |
Glycogen storage capacity, glycogen synthase, GLUT-4 transporter protein content, translocation to membrane | Decrease |
Lipoprotein lipase activity | Decrease |
Total cholesterol, LDLacholesterol | Increase |
HDLacholesterol | Decrease, no change |
Hormonal and sympathetic nervous system response to stress | Increase |
Growth hormone, IGF-1b | Decrease |
Heat and cold tolerance, temperature regulatory ability | Decrease |
aLDL, low-density lipoprotein; HDL, high-density lipoprotein.
bMost training studies show no change in growth hormone or circulating IGF-1 although tissue levels of IGF-1 may increase.
Table 10.4 Changes in central and peripheral nervous system due to ageing or disuse potentially modifiable by physical activity.
Function | Effect of ageing or disuse |
REMaand slow-wave sleep duration | Decrease |
Cognitive processing speed, accuracy | Decrease, no change |
Attention span | Decrease, no change |
Memory | No change, decrease |
Executive function | Decrease, no change |
Motor coordination, force control | Decrease |
Neural reaction time, neural recruitment | Decrease |
Autonomic nervous system function | Decrease |
aREM, rapid eye movement.
Although changes in maximum work capacity (aerobic fitness or maximum oxygen consumption) will be immediately noticeable and disastrous for an elite athlete, they may accrue insidiously in non-athletic populations because most sedentary individuals rarely call upon themselves to exert maximum effort in daily life. Women are particularly susceptible here, because their initial reserve of muscle mass is so much lower than that of men, owing to gender differences in anabolic hormonal milieu and also lifestyle/occupational factors. They will therefore cross this threshold where losses of musculoskeletal capacity impact on functional status at least 10 years before men do on average.
Another important consequence of age-related changes in physiological capacity is the increased perception of effort associated with submaximum work (a lowering of the anaerobic threshold or the approximate level at which significant dyspnoea occurs). This changing physical capacity has the unfortunate negative side effect of increasing the tendency to avoid stressful activity. Such behavioural change compounds the sedentariness caused by changing job requirements or retirement, societal roles and expectations and other psychosocial influences. Thus, a vicious cycle is set up: ‘usual’ ageing leading to decreasing exercise capacity, resulting in an elevated perception of effort, subsequently causing avoidance of activity and finally feeding back to exacerbation of the age-related declines themselves secondary to the superimposition of disuse on biological ageing.
Many studies suggest that chronic adaptation to physical activity can markedly attenuate decrements in exercise capacity that would otherwise occur with ageing (see Tables 10.1–10.4), with the notable exception of maximum heart rate (due to declining sensitivity to β-adrenergic stimulation in the ageing heart).2 Although the peak exercise workload achievable is therefore always lower in aged individuals, the cardiovascular and musculoskeletal adaptations to chronic aerobic exercise enable the trained individual to sustain higher submaximum workloads with less of a cardiorespiratory response (heart rate, blood pressure and dyspnoea), and also less overall and musculoskeletal fatigue.
Musculoskeletal function (strength, power, muscle endurance) in ageing is dictated largely by the size of the muscle mass which is contracting and to a lesser extent by changes in surrounding connective tissue in the joint (cartilage, tendons and ligaments) and neural recruitment, conduction velocities, glycolytic and oxidative enzyme capacities and fatigue patterns. Sedentary individuals lose large amounts of muscle mass over the course of adult life (20–40%) and this process, termed sarcopenia, plays a major role in the similarly large losses in muscle strength observed in both cross-sectional and longitudinal studies.3 However, unlike many other changes which impact on exercise capacity, muscle mass cannot usually be maintained into old age even with regular aerobic activities in either general populations or master athletes. Only overloading of muscle with weight-lifting exercise (resistance training) has been shown largely to avert losses of muscle mass (and also strength) in older individuals. For example, Klitgaard et al.4 found that elderly men who swam or ran had similar measures of muscle size, strength and metabolism as their sedentary peers, whereas the muscle of older men who had been weight-lifting for 12–17 years was almost indistinguishable, and even superior in some aspects, to that of healthy men 40–50 years younger than them.
Clearly, habitual exercise has the potential to lessen the impact of biological ageing on two of the major elements of exercise capacity: aerobic fitness and muscle strength. Similarly, there is evidence that balance training and flexibility training5 induce adaptations in associated declines in these areas.
Optimization of Body Composition with Ageing
‘Usual ageing’ is associated with significant losses of bone and muscle (lean mass) and increases in adipose tissue, along with central and visceral shifts in the regional distribution of adipose tissue stores. The extent to which these changes occur in an individual depends upon a combination of genetic-, lifestyle- and disease-related factors that are all interrelated. All of these body composition changes may impact negatively upon metabolic, cardiovascular and musculoskeletal function,6 even in the absence of overt disease, and therefore it is important to anticipate them and optimize lifestyle choices and other treatments which can counteract the negative effects of ageing and/or disease on body composition. As detailed in the sections that follow and outlined in Table 10.5, one of the most potent pathways from physical activity to health status involves the modulation of these age-related shifts in body composition by habitual exercise patterns.
Table 10.5 Exercise recommendations targeting optimal body composition for older adults.
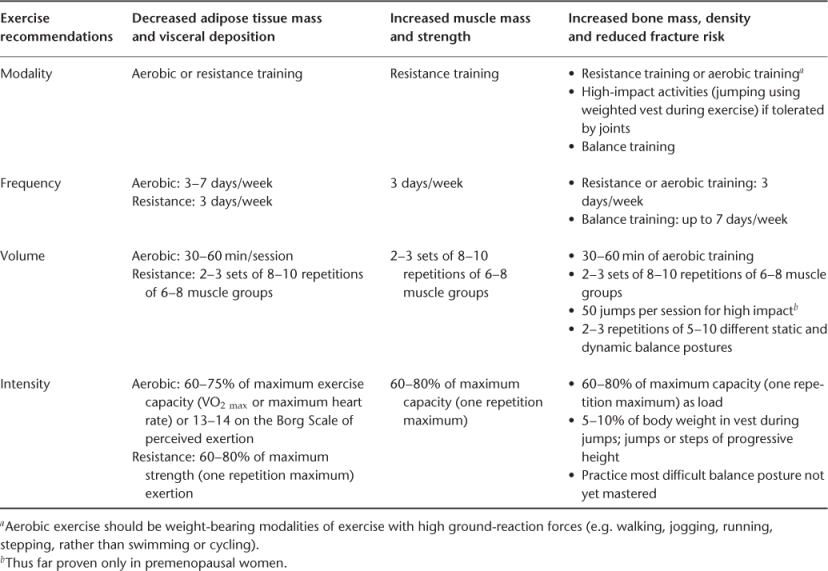
Role of Exercise and Physical Activity in Bone Health and Fracture Risk
Age-related Changes in Bone
Bone mass begins to decrease well before the menopause in women (as early as the 20s in the femur of sedentary women) and accelerates in the perimenopausal years, with continued declines into late old age. Similar patterns are seen in men, without the acceleration related to loss of ovarian function seen in women. As with losses of muscle tissue (sarcopenia), many genetic-, lifestyle-, nutritional- and disease- and medication-related factors enter into the prediction of bone density at a given age.
Physical Activity and Bone Health
A wealth of animal and human data provide evidence for a relationship between physical activity and bone health at all ages. Mechanical loading of the skeleton generally leads to favourable site-specific changes in bone density, morphology or strength, whereas unloading (in the form of bed rest, immobilization, casting, spinal cord injury or space travel) produces rapid and sometimes dramatic resorption of bone, increased biochemical markers of bone turnover, changes in morphology such as increased osteoclast surfaces and increased susceptibility to fracture.
Comparative studies of athletic and non-athletic populations usually demonstrate significantly higher bone density in the active cohorts, ranging from 5 to 30% higher, depending on the type, intensity and duration of exercise training undertaken and the characteristics of the athletes studied. Exceptions occur with non-weight-bearing activities such as swimming or amenorrhoeic or competitive distance runners, who appear similar to controls. Similarly, on a smaller scale, differences are often observed between habitually active and sedentary non-athletic individuals.
Experimental evidence in animal models and also some human data suggest that changes in bone strength not directly correlated with density may contribute to the overall benefits of mechanical loading for skeletal integrity and resistance to fracture (e.g. increased bone volume or altered trabecular morphology), so that evaluating bone density changes alone likely underestimates the skeletal effects of loading.
Consistent with bone density findings noted above, hip fracture incidence has been observed to be as much as 30–50% lower in older adults with a history of higher levels of physical activity in daily life, compared with age-matched, less active individuals. For example, in the prospective epidemiology of osteoporosis study (EPIDOS) study of 6901 white women over the age of 75 years followed for 3.6 years, investigators found that a low level of physical activity increased the risk for proximal humerus fracture by more than twofold. The relative risk of fracture in sedentary women (RR = 2.2) was greater than that attributable to low bone density (RR = 1.4), maternal history of hip fracture (RR = 1.8) or impaired balance (RR = 1.8). The interaction of these risk factors is indicated by the fracture rate, which rose from about 5 per 1000 woman-years in individuals with either bone fragility or high fall risk to 12 per 1000 woman-years for women with both types of risk factors. Such data suggest the great potential utility of multifactorial prevention programmes for osteoporotic fracture that can address both bone density and fall risk (sedentary behaviour, sarcopenia, muscle weakness, poor balance, polypharmacy, etc.) simultaneously.
Exercise Intervention Trials in Postmenopausal Women and Older Men
Significant changes in the femur, lumbar spine and radius have been seen following aerobic training, resistance training and combined programmes of aerobic and resistive exercise. Unlike results obtained in younger women, isolated high-impact training (jumping, skipping, heel drops) has not yet been found to be effective in studies of postmenopausal women. High dropout rates (30–50%) are problematic in these trials, raising the issue of generalizability and sustainability of the outcomes observed. This is particularly relevant to fracture prevention efficacy of exercise, as several studies have shown complete or partial reversal of gains in bone mineral density (BMD) after the cessation of training.
For older men and women, a combination of decreased anabolic hormones (estrogen, testosterone, growth hormone), increased catabolic milieu (higher leptin and cortisol associated with visceral adipose tissue), the emergence of musculoskeletal and other diseases, retirement and reduced recreational activities have a major negative impact on both bone and muscle tissue. The majority of studies demonstrating the efficacy of aerobic or resistive exercise on bone density have been conducted in women between 50 and 70 years of age and it is not yet known if efficacy would be similar in older women with multiple comorbidities, who have usually been excluded from such trials. Both types of exercise have approximately equivalent effects on bone health in postmenopausal women of about 1–1.5% per year between exercisers and non-exercisers in meta-analyses of well-designed trials.
Optimal Exercise Modality and Intensity for Bone Health
The predominant exercise training factor that influences bony adaptation is the intensity and novelty of the load, rather than the number of repetitions, sets or days per week or even total duration of the programme. This observation is also true for animal models of mechanical loading, in which bone is most sensitive to short periods of loading characterized by unusual strain distribution, high strain magnitudes and rapid rate of loading.
The relative efficacy of aerobic versus resistive exercise regimens for postmenopausal women may perhaps be best assessed via studies that have directly compared various intensities of these two exercise modalities in randomized subjects. Kohrt et al.7 found that both aerobic activities with high ground-reaction forces (walking, jogging, stair climbing) and exercises with high joint-reaction forces (weight-lifting, rowing) significantly increased the BMD of the whole body, lumbar spine and Ward’s triangle, whereas only the ground-reaction group increased BMD at the femoral neck.7 The weight-lifting group preserved femoral-neck BMD relative to controls, as has been seen in other resistance training studies. However, lean mass and muscle strength increased only in the weight-lifting group, leaving overall benefits of these two types of exercise for ultimate fall and fracture prevention still unresolved. Kerr et al.8 randomized 126 postmenopausal women to 2 years of high-intensity weight-lifting exercise, moderate-intensity aerobic training (circuit training and stationary cycling) or sedentary control condition. Total hip and intertrochanteric BMD was improved only by strength training and was significantly different from aerobic training or control groups (+3.2% at 2 years). As most comparative studies other than those of Kohrt et al.7 and Kerr et al.8 have not sought to optimize both modalities, it is still not possible to choose definitively one best modality for all bone sites. In general, the older the individual, the more favourable the resistance training appears, due to its broader benefits on muscle, bone, balance and fall risk, relative to aerobic training. If aerobic training is chosen, however, activities that are weight bearing and higher impact have a greater efficacy than non-weight-bearing or low-impact aerobic activities.
It is important to consider not only the optimal modality of exercise, but also the relative intensity, as the skeletal adaptation is critically linked to the intensity of the loading (whether due to increased amount of weight lifted during resistance training or higher ground-reaction forces during aerobic/jumping activities). Interesting results have been reported by Cussler et al.9 in a randomized trial of 140 postmenopausal women participating in a multimodal exercise programme (high-intensity resistance training and a weight-bearing circuit of moderate-impact activities including walking/jogging, skipping, hopping, stair climbing/stepping with weighted vests). Bone density improvements at the femoral trochanter were significantly and linearly related to total weight lifted during the 12 months, and also total weight lifted in leg press, squats and military press exercises, but not to volume or quality of the non-resistance training components of the programme. High-intensity resistance training is also more beneficial than low-intensity training for muscle strength gains and muscle hypertrophy, in addition to associated gait disorders, functional impairments and disability, making it ideal as a multiple risk factor intervention strategy for injurious falls in osteopenic women.
Exercise in the Treatment of Osteoporotic Fracture
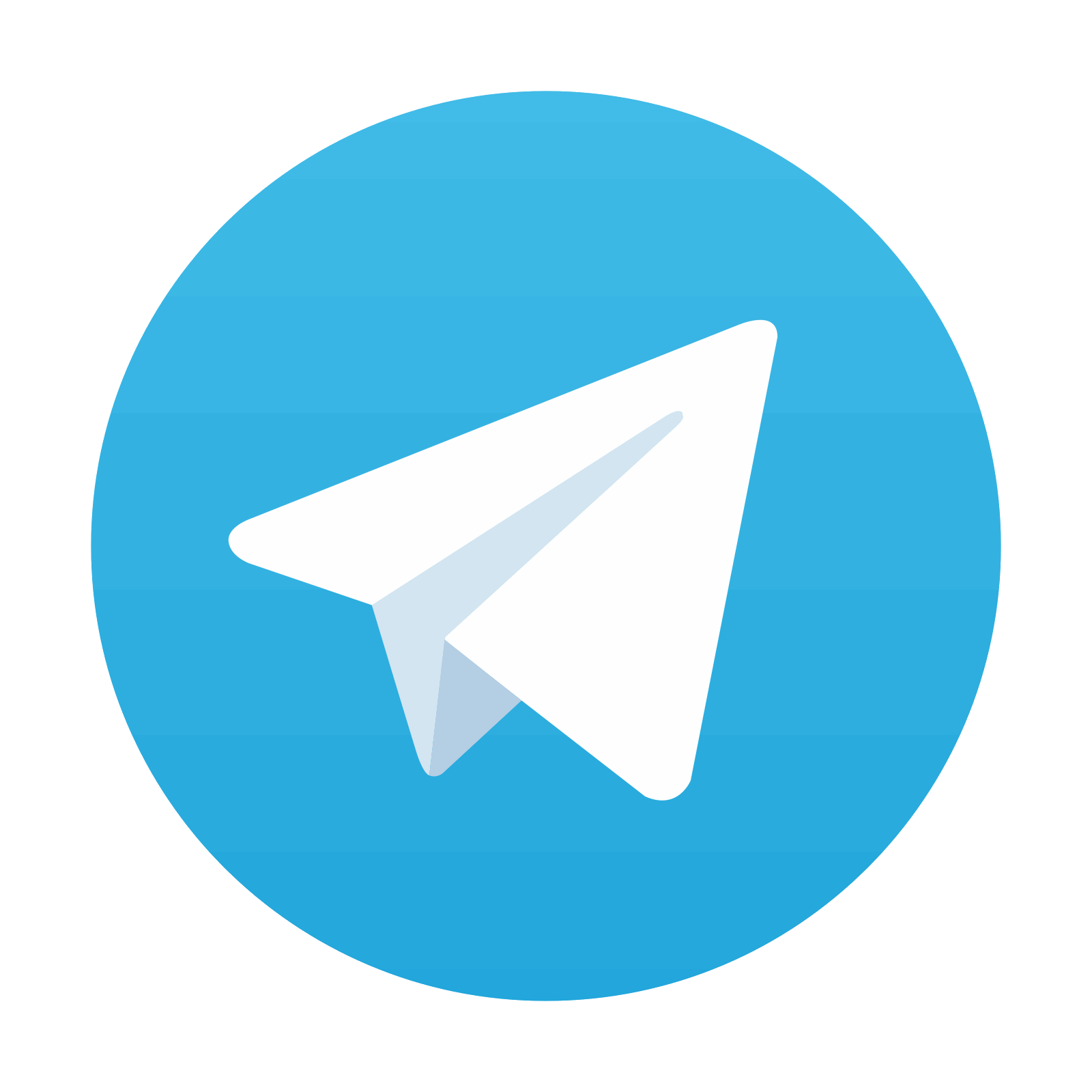
Stay updated, free articles. Join our Telegram channel

Full access? Get Clinical Tree
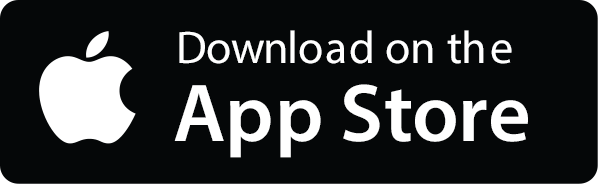
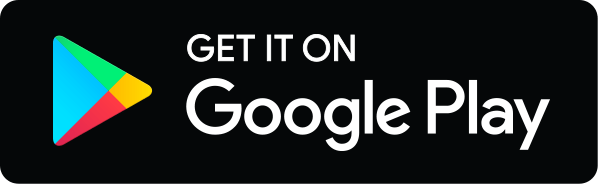