Fig. 1
a Time-lapse near-IRfluorescence (NIRF) imaged mice bearing HCT-116 tumors after intravenous injections of IR-780 micelles. b NIRfluorescence intensities and contrast index (CI) values were quantified at the indicated time points in the tumor and normal regions, using the maximal NIRF signals in the nontumor regions. c Near-IRfluorescence (NIRF) images and d quantification of various organs at 24 h after intravenous injection of IR-780 micelles. Each column represents the mean (SD). The abbreviations indicate: H heart; Li liver; Sp spleen; Lu lung; K kidney; and In intestine. Adapted from Peng et al., with permission from American Chemical Society. Copyright 2011
A pH dependant doxorubicin release system was devised by Zou et al. where PEGylated superparamagnetic iron oxide (SPIO) nanoparticles were surface functionalized with cancer specific HuCC49ΔCH2 antibody and fluorescent dye 5-FAM. A modified version of doxorubicin i.e. azido-doxorubicin was entrapped in the PEG moieties attached to the surface of SPIONs. The sessile pH dependent azide bond introduced in the drug enables the theranostic system to release doxorubicin only in acidic environment prominently found in the vicinity of cancer cells (Zou et al. 2010).
1.1.2 PLGA Based Nanotheranostics
PLGA is one of the most extensively used biocompatible polymers for formulation of a nanoscale theranostic systems. The therapeutic moieties delivered by such formulations spans across drugs, proteins, RNA, DNA and peptides (Bouissou et al. 2006; Jain 2000; Ruhe et al. 2003). An interesting PLGA based theranostic system has been devised by Shi et al. wherein anticancer drug paclitaxel and MRI contrast magnetic nanoparticles were loaded inside PLGA carrier polymer. In order to further track the system under in vivo conditions fluorescent QDs were also grafted onto its surface. The iron oxide nanoparticle incorporated in the complex apart from providing contrast in MRI is also utilized for hyperthermia which synergizes the therapeutic effects of paclitaxel over the cancer cells. The theranostic system thus synthesized was conjugated with anti-prostate specific membrane antigen antibody for attaining targeted delivery to cancer cells alone. The system was validated under in vitro and in vivo systems for its cancer specific theranostic application (Cho et al. 2010).
The earliest reports of PLGA nanoparticles for theranostic application dates back to 2005 where McCarthy et al. used them for encapsulation and delivery of meso-tetraphenyl porpholactol which served the purposed of both imaging agent and cytotoxic agent when stimulated by external source. The complex was observed to be effective in curing cancers in mouse models with simple stimulation by external visible light source (McCarthy et al. 2005).
In another instance PLGA nanoparticles were utilized for overcoming multiple drug resistance with concomitant delivery of paclitaxel. In order to overcome drug resistance MDR1 gene silencer, P-gp targeted siRNA (viabiotin) was attached to PLGA which clearly exhibited an improved anti-cancer activity as compared to paclitaxel alone (Patil et al. 2010). Inclusion of a suitable imaging agent in such complexes can improve the diagnosis of drug resistant tumors which could be very much beneficial for efficient follow-up after cancer therapy. A similar system with targeted theranostic potential was deviced by Deepagan et al. where PLGA and PEG copolymer nanoparticles were used as carriers for delivery of camptothecin and ZnS QD. The complex was functionalized with antibody cetuximab for targeting it to the tumor site. The presence of therapeutic drug and imaging agent (i.e. theranostic) enables continuous follow-up of treatment for better management to treatment procedures (Deepagan et al. 2012). As an alternative to antibody, prostate-specific membrane antigen (PSMA) aptamers conjugated with biodegradable PEG-PLGA and PEG-poly(lactic acid) (PLA) micelles were synthesized by Farokhzad et al. for targeted delivery of docetaxel (Farokhzad et al. 2004, 2006). A specific drawback with PLGA is its imminant clearance from the circulatory system and in an attempt to overcome this often PEG moieties are grafted to PLGA which could have prolonged circulation time as it evaded the RES of the host (Akbarzadeh et al. 2012). In course with this, a PLGA-PEG based nanoparticles by the commercial name BIND-014 is being evaluated in clinical trials for controlled and targeted delivery of chemotherapeutic agents (Hrkach et al. 2012). Another group led by Liu reported synthesis of mPEG-PLGA-b-PLL block copolymer nanocapsules for delivery of anticancer drug adriamycin and siRNA. The complex antitumor efficacy was validated against Huh-7 hepatic carcinoma-bearing mice and was monitored simultaneously by NIR fluorescence dye Cy5 grafted onto its surface (Liu et al. 2012).
1.1.3 Chitosan Based Nanotheranostics
Chitosan is a natural biodegradable polymer with ionisable amino group favorable for functionalization with therapeutic and/or diagnostic molecules. A drawback of chitosan related polymer is its solubility which has led to synthesis of various other water soluble derivatives of chitosan like glycol chitosan and carboxy methyl chitosan. In one of the recent reports, Lee et al. have used glycol chitosan for the delivery of chlorin e6 (Ce6) which could serve the purpose of both in vivo imaging and photodynamic therapy. In order to further improve the Ce6 release profile, circulation half-life and its passive accumulation in tumors the Ce6 molecules were conjugated to hydrophobically modified glycol chitosan i.e. glycol chitosan-5β-cholanic acid. In vivo NIR imaging in mice models clearly indicated accumulation of the theranostic system at tumor sites. The subsequent prognosis of tumor after laser irradiation indicated instance of tumor necrosis and decline in tumor volume (Lee et al. 2011). The same carrier molecule (i.e. 5β-cholanic acid modified glycol chitosan) was utilized by Kyung et al. for encapsulation and delivery of hydrophobic anticancer drug camptothecin. The carrier could provide a sustained and prolonged release profile lasting for a week and also showed a tendency to passively accumulate at the tumor site when investigated in nude mice implanted with MDA-MB231 human breast cancer xenografts. A drastic reduction in tumor size was confirmed by near infrared fluorescence during the course of treatment (Kyung et al. 2008). In another independent work, glycol chitosan-cholanic acid nanoparticles were used to encapsulate and deliver doxorubicin and Bcl-2 siRNA in order to overcome drug resistance and facilitate an effective anticancer action of drug doxorubicin. The in vivo fate of both glycol chitosan based nanoparticles was monitored by non-invasive near infrared fluorescence imaging system (Fig. 2) (Yoon et al. 2014). The same amphipathic version of chitosan was shown to attain tumor targeted delivery by metabolic glycoengineering and click chemistry (Lee et al. 2014). The targeted delivery of precursor molecules by glycol chitosan-cholanic acid carrier molecule enabled tumor cells surface functionalization with azide groups. The following administration of copper-free click chemistry based anticancer drug efficiently localized their accumulation in the pre-functionalized (azide) tumor tissues.
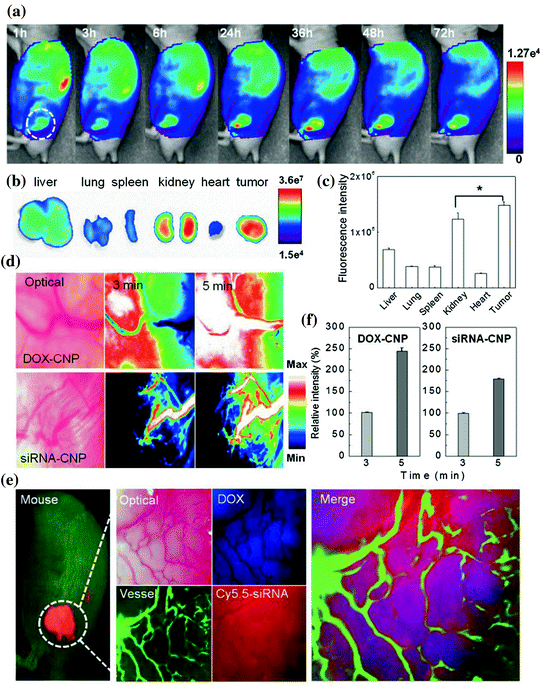
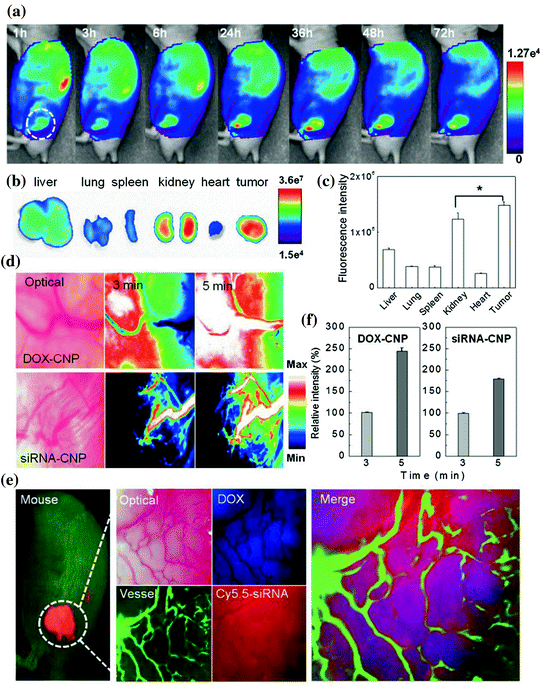
Fig. 2
In vivo fate of both glycol chitosan based nanoparticles by near infrared fluorescence (NIRF) imaging system. a Time dependent whole body distribution of CNPs. b Fluorescence visualization and c quantification from the ex vivo NIR fluorescence images of dissected tumors and organs 72 h post-injection. d, f In vivo real-time tumor accumulation behaviors of both Cy5.5-CNPs in the solid tumor. e The multifiltered live imaging of targeted release and localization of DOX and siRNA of CNPs in a solid tumor. Adapted from Yoon et al., with permission from Nature Publishing Group. Copyright 2014
Chitosan being laden with umpteen number of cationic amine groups is extensively used for delivery of nucleic acid to cancer cells. Inspite of its promising outcomes in delivery of siRNA and DNA to cancer cells under invitro conditions, chitosan in vivo application is limited due to interaction with hyaluronic acids present in the extracellular matrix. Thus, in order to overcome this, Ki et al. worked out on improving the physical stability of chitosan complexes. The authors proposed hybrid nanocomplexes of chitosan protamine, lecithin and thiamine pyrophosphate for delivery of surviving siRNA to Prostatic Small Cell Carcinoma (PC3). A marked decline (i.e. 21.9 %) in SVN expression was observed which suggested tumor targetability and growth inhibitory effects of the composite system (Ki et al. 2014). Another modified form of chitosan, thiolated chitosan nanoparticles were used by Anitha et al. for combined delivery of curcumin and 5-fluorouracil to colon cancer cells. The drug pharmacokinetics and biodistribution was evaluated further in Swiss Albino mouse models. The system demonstrated improved bioavailability of drugs in the blood plasma with simultaneous combinatorial anticancer effects of curcumin and 5-fluorouracil (Anitha et al. 2014).
A group headed by Deng et al. employed hyaluronic acid conjugated chitosan nanoparticles (HA-CS NPs) for delivering miR-34a (a tumor suppressive molecule in breast cancer) and doxorubicin to breast cancer cells with special emphasis on overcoming metastatic relapse. The study also showed the synergistic anticancer effects attained by codelivery of miR-34a and doxorubicin by HA-CS NPs (Deng et al. 2014).
In order to attain stimulated release of drug in a cancer specific milieu, a pH sensitive and temperature responsive poly(N-isopropylacrylamide)-chitosan nanohydrogels were synthesized by Jaiswal et al. Fe3O4 magnetic nanoparticles were also incorporated in nanohydrogel for hyperthermia for temperature stimulation of the composite system by AC magnetic field (AMF). The anticancer potentials of the therapeutic system was validated against human breast (MCF-7) and cervical carcinoma (HeLa) cells under in vitro conditions, which was 35–45 % in HeLa cells and 20–70 % in MCF-7 cells depending upon the AMF applied (Jaiswal et al. 2014).
A chitosan derivative, N-Carboxyethyl chitosan ester (CS-EA) was synthesized by another group to attain aptamer (MUC1 DNA) mediated targeted delivery of SN38 to colon cancer. The anticancer potentials of the system was investigated against MUC1 positive cell line and the corresponding cellular uptake and targeting was confirmed by confocal microscopy (Yoon et al. 2014). Poly(ethylene glycol) grafted chitosan copolymer was conjugated with tumor targeting siRNA/folic acid to form a theranostic nanoformulation. The theranostic system attained better compatibility with erythrocytes and effectively inhibited proliferation by gene knockout in BALB/c mice bearing OVK18 tumor xenograft by in vivo imaging. The preliminary in vitro studies were well complimented with flow cytometry RT-qPCR and western blot analysis for transfection and gene silencing activity (Li et al. 2014). Glycol chitosan has also been modified with different extents of hydrophobic N-acetyl histidine (NAcHis-GC) which is tagged with I131. Similarly I131 tagged doxorubicin was also encapsulated within NAcHis-GC for continuous monitoring using a gamma camera. The same system was evaluated under in vivo conditions with near-infrared fluorescence Cy5.5-labeled NAcHis-GC. The therapeutic outcomes of NAcHis-GC nanoparticles loaded with doxorubicin was compared with other alternative carriers reported thus far and was verified against xenograft mice models (Lee et al. 2010).
It is clear from afore mentioned examples that polymers have revolutionized the field of cancer therapy and diagnostics. The targeted delivery of two or more synergistic drugs to cancer cells with simultaneous real-time imaging system would be sought after as the future theranostic system for efficient management of cancer.
2 Dendrimers: Introduction
Dendrimers represent a class of chemically synthesized globular molecules with very well-defined structures. From the view of polymer chemistry, dendrimers are nearly defect-free, monodisperse (meaning consistent size and form) moieties with highly branched three-dimensional structure. Graphically, their architecture and dimensions resemble to small proteins and hence sometimes referred to as artificial proteins. The structural components of dendrimers include a central core from which branches (interior layers) emanate in an ordered fashion. The terminal groups attached to the outermost interior generations are mainly responsible for the functionality of the dendrimer (Lee et al. 2005) (Fig. 3). Dendrimers are produced by a series of repetitive chemical reactions, in which each additional iteration leads to a new layer i.e. ‘generation’ with double the number of active sites (peripheral end groups) and approximately twice the molecular weight of preceding generation. These moieties gained conception in the late 1970s and early 1980s with the pioneering works of Tomalia, Vogtle, Denkewalter, Newkome and co-workers. Tomalia and group demonstrated the iterative coupling of ethylene diamine to a central ammonia core to form various branched macromolecules and entitled them as ‘starburst dendrimers’ or poly(amidoamine) PAMAM dendrimers (Tomalia et al. 1985). Vogtle and co-workers, studied the controlled synthesis of dendritic units to produce polymeric macromolecules with large cavities and designated them as ‘cascade molecules’ (Vogtle et al. 1978). The first ever dendritic wedge with lysine residues at the branching points was reported by Denkewalter et al. (1981). Newkome’s group called dendrimers as “arborols” (Latin ‘arbor’ means a tree) (Newkome et al. 1985). Synthesis of dendrimers by specific chemical reactions is an appropriate example of controlled-hierarchical synthesis, an approach that enables ‘bottom–up’ creation of intricate systems. Initially, the researchers emphasized on understanding the synthesis, chemical and physical properties of dendrimers, but recently the focus has shifted to exploring the potential biological applications of dendrimers. Recently, dendrimers offer wide scope in various fields ranging from drug/gene delivery to cellular imaging to development of vaccines, antibacterial, antiviral and anticancer agents. (Aulenta et al. 2003; Stiriba et al. 2002; Patri et al. 2002; Boas and Heegaard 2004).
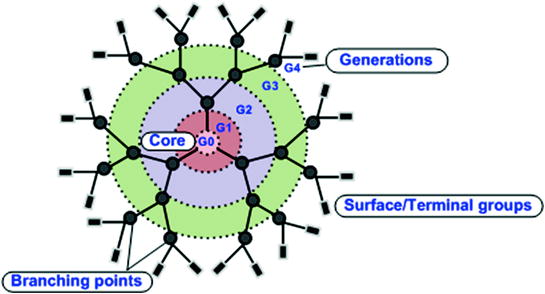
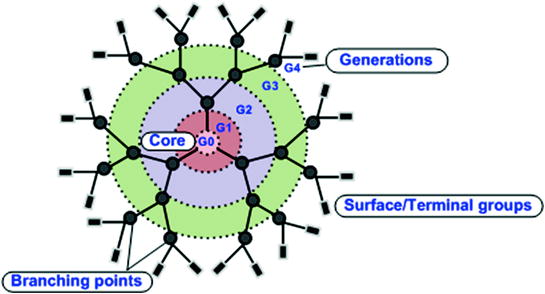
Fig. 3
Structural components of a G4.0 PAMAM dendrimer
The unique molecular architecture of dendrimer macromolecules impart those significantly improved physicochemical properties when compared to linear polymers. In solution, linear chains exist as random coils; while dendrimers tend to stay as dense shells. Moreover, the exterior functional groups of dendrimers impart them with properties of high solubility, miscibility and reactivity. The structural versatility and controlled multivalence of dendrimers can be used to perform multiple conjuagtion reactions to attach several drug molecules, targeting moieties and solubilizing groups to the dendrimer periphery. Moreover, dendrimers with low polydispersity contrarily to some linear polymers with distinct molecular weight can provide reproducible pharmacokinetic behavior. Such interesting properties make dendrimers highly suitable for biological applications (Duncan et al. 2001).
2.1 Dendrimers as Drug Carriers
Polymeric nanocarriers hold great promise as delivery systems to increase the solubility and circulation times of therapeutic cargo (Duncan 1992). Also, several reports suggest the ability of polymer based carriers for drug delivery by passive targeting to solid tumors. Increased permeability of tumor vasculature and poor lymphatic drainage system enable enhanced accumulation of nanocarriers in tumor region by a phenomenon termed the ‘enhanced permeation and retention’ (EPR) effect (Matsumura and Maeda 1986). The monodispersity, multivalency and unique structural properties of dendrimers make them prospective candidates for anticancer drug delivery applications.
2.1.1 Noncovalent Encapsulation of Drugs
The well-defined, three-dimensional shape of dendrimers mediate formation of internal nano-cavities which can act as centres to encapsulate guest molecules by supramolecular host-guest interactions. Large number of functional groups at the surface of high generation dendrimers can form a stearic shell separating the core from the exterior bulk to protect the entrapped guest molecules. Such encapsulation of guest molecules by dendrimers is based on the idea of “dendritic box”. In recent years numerous reports are available wherein high generation dendrimers have been used for accommodation of anticancer drugs under physiological conditions. However, in this approach it is difficult to control the release of drug molecules from the dendrimer core. Bhadra et al. (2003) suggested that introduction of high molecular weight poly(ethylene) glycol (PEG) chains on the dendrimer periphery for 5-fluorouracil (5-FU) delivery can enhance the circulation times, reduce the drug leakage and minimise haemolytic toxicity due to parent dendrimer. Another strategy could be development of pH responsive dendrimers which can specifically release the therapeutic cargo at low pH tumor environments. Jin et al. (2011) developed pH responsive PPD nanocarrier with poly(2-(N,N-diethylamino)ethyl methacrylate) (PDEA) chains introduced onto methoxy-poly(ethylene glycol) (mPEG) poly(amidoamine) (PAMAM) dendrimer for 5-FU delivery under in vivo conditions. The nanocarrier exhibited long half-lives and high tumor targeting ability than free 5-FU. Under acidic conditions (pH < 6.5) typical of tumor environment, the PDEA chains were extended allowing release of 5-FU, whereas in neutral or basic conditions (pH > 7.4) the PDEA chains restrict the release of 5-FU and 5-FU was intact in the nanocarrier.
Moreover, dendrimers with high density of functional groups (such as amine groups and carboxyl groups) on the surface are also known to electrostatically interact with the drug molecules enhancing the solubility of hydrophobic drugs. Non-steroidal anti-inflammatory drugs bearing carbonyl groups such as ibuprofen, ketoprofen, naproxen, diflunisal and indomethacin have been reported to electrostatically interact with dendrimer moieties. Weakly acidic anticancer and antibacterial drugs with carbonyl groups are also known to complex with amine terminated dendrimers (Chen et al. 2004). Such properties make dendrimers highly suitable as drug delivery systems.
2.1.2 Covalent Conjugation of Drugs
Another strategy could be covalent conjugation of drug molecules with the dendrimer external functional groups. In this approach, the covalently bound drugs can be released via chemical or enzymatic cleavage of hydrolytically labile bonds. Complexation of drugs with the dendrimer molecules by simple encapsulation or via electrostatic interactions can retain the chemical integrity and pharmacological properties of drug molecules, while covalent conjugation of drugs to the surface functional groups of dendrimers through chemical linkages can enable controlled drug release which cannot be achieved by simple encapsulation/electrostatic complexation approaches. A schematic representation of different strategies by which dendrimer can bind with the drug molecules is shown in Fig. 4.
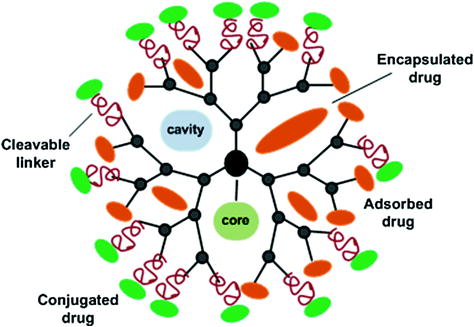
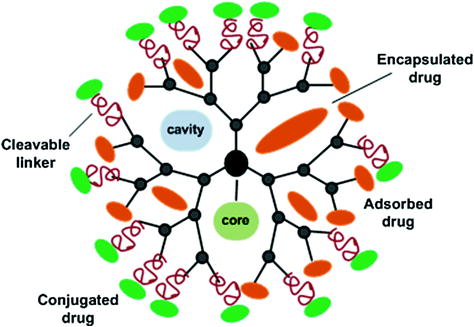
Fig. 4
Schematic representation of dendrimer-drug complexation strategies
Patri and co-workers compared the release kinetics of covalently conjugated and hydrophobically complexed methotrexate (MTX) drug to G5 PAMAM dendrimers. The activity of methotrexate complexed within dendrimer was comparable to free drug in vitro while the covalently conjugated drug was better suited for targeted drug delivery as it inhibits the premature release of the drug under biological conditions (Patri et al. 2005). Another study suggested stochastic functionalization of MTX and TAMRA (tetramethylrhodamine) fluorophore by copper-free click chemistry to PAMAM dendrimers (Fig. 5). The reaction involves esterase stable amide linkages and the formed conjugate exhibited comparatively less toxicity than free MTX drug towards B16-F10 (melanoma) cells (Thomas et al. 2012).
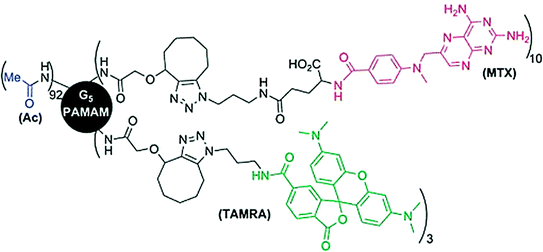
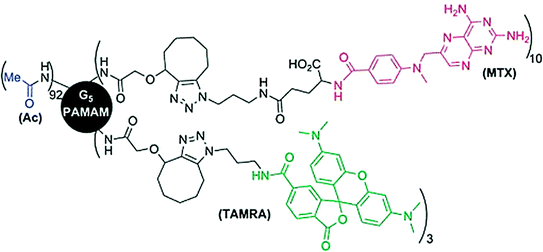
Fig. 5
Stochastic grafting of methotrexate (MTX) by a stable bond to PAMAM G5 dendrimers. Adapted from Caminade et al., with permission from Royal Society of Chemistry. Copyright 2014
However, covalent conjugation of active drugs through stable bonds can alter its efficiency (Goller et al. 2001). In a comparative study, doxorubicin was conjugated to G4 PAMAM dendrimers either through a non-cleavable amide group (a PEG-PAMAM-succinic-DOX (PPSD) conjugate) or an amide group cleavable under acidic conditions (a PEG-PAMAM-cis-aconityl-DOX (PPCD) conjugate) (Fig. 6). PEG derivative was utilized to enhance the conjugates water solubility, reduce toxicity towards healthy cells and enhanced tumor uptake. Although both conjugates were internalized by Skov-3 cells (ovarian carcinoma) but only cleavable PPCD conjugates were able to release DOX to induce cell death while PPSD conjugates displayed no toxic effects (Zhu et al. 2010).
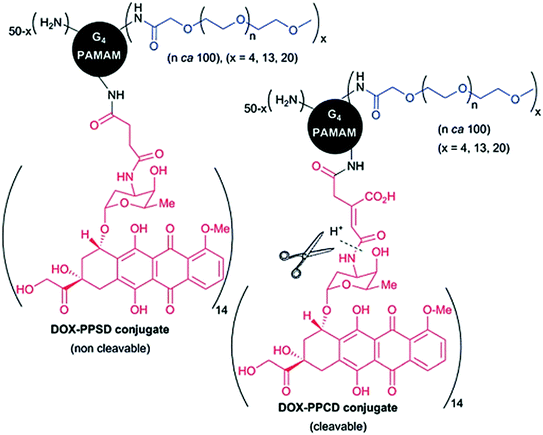
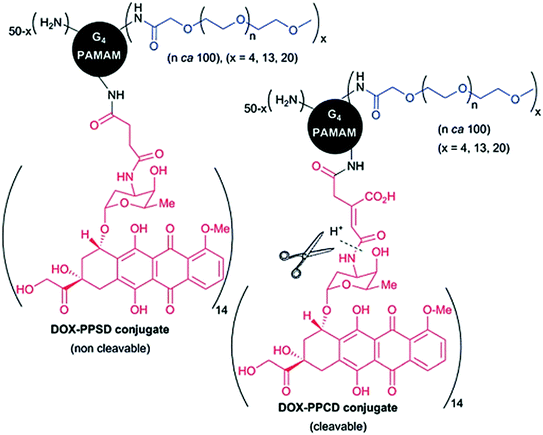
Fig. 6
G4.0 PAMAM dendrimers stochastically modified with DOX through a non-cleavable or cleavable bond. Adapted from Caminade et al., with permission from Royal Society of Chemistry. Copyright 2014
Use of Janus dendrimers (two types of terminal functions in two different areas of the surface of the dendrimer) for the formulation of dendrimer-drug conjugates has been described (Caminade et al. 2012). An asymmetric biodegradable polyester dendrimer (modified with PEG derivatives) conjugated to DOX with cleavable acyl hydrazone linkages has been used for in vivo assessment in mice bearing C-26 colon carcinoma tumors (Fig. 7). A single intra venous (i.v.) injection of the Janus dendrimer-DOX conjugates was able to release DOX (through cleavage of pH sensitive acyl hydrazone linker) causing complete tumor regression and 100 % survival of mice, whereas with free DOX or Janus dendrimer-DOX conjugate linked through stable carbamate bond, no cure was achieved. Ability of dendrimer to favourably modulate the pharmacokinetics of conjugated DOX can be held worthy of the remarkable antitumor activity of dendrimer-DOX conjugate (Lee et al. 2006).
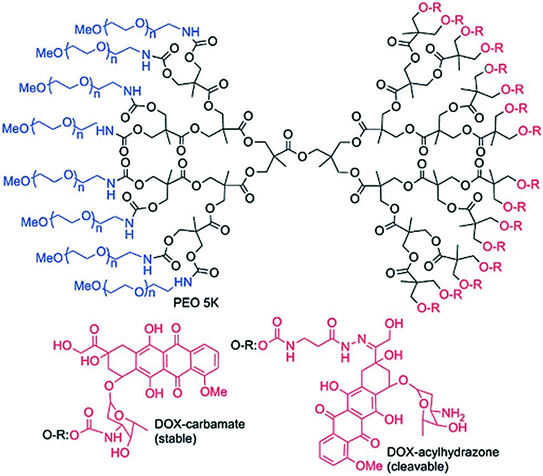
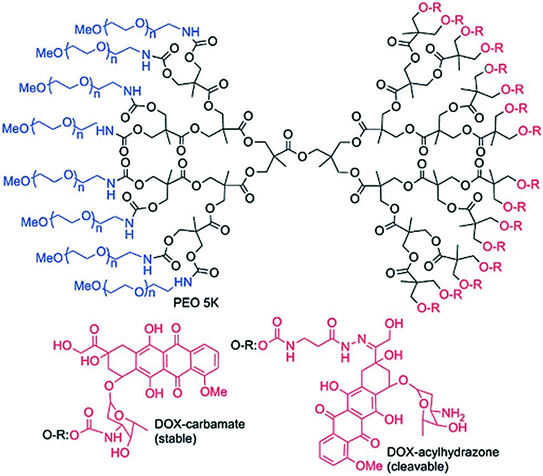
Fig. 7
DOX functionalized Janus dendrimers through cleavable hydrazone linkages or stable carbamate bonds. Adapted from Caminade et al., with permission from Royal Society of Chemistry. Copyright 2014
All the above stated examples of dendrimer-drug conjugates involve use of pH sensitive linkages, i.e. release of attached drug occurs specifically under acidic conditions. Such linkers are expected to be cleaved more rapidly in cancer cells (with low pH conditions) than normal cells, which is still a hypothetical condition (Tannock and Rotin 1989; Griffiths 1991). Generally, as stated before, covalent conjugation of drugs to the dendrimer periphery (wherein the release of drug can be controlled) can exert more antitumor activity compared to free drug or dendrimer-entrapped drug under similar biological conditions.
2.2 Dendrimers for Tumor Imaging
Structural flexibility and availability of multiple attachment sites on the dendrimer surface has open up avenues for development of dendrimer based Computed Tomography (CT), nuclear medicine, optical imaging and Magnetic Resonance (MRI) Imaging agents. The ideal molecular imaging agent would be specifically directed towards the target organ and should deliver the imaging payload without any alteration in its functionality. The targeting ligands can be attached to dendrimer exterior termini and contrast agents can either be conjugated with the peripheral groups or can be resided in the core (Longmire et al. 2008). Two classes of dendrimers with MR imaging proficiencies include: dendrimers incorporating gadolinium (Gd) chelates and magneto-dendrimers (i.e. dendrimers containing paramagnetic iron oxide particles).
Dendrimer based MRI agents offer better relaxivity and increased circulation times than conventional low molecular weight (LMW) contrast agents such as Gd-DTPA (DTPA = diethylenetriaminepentaacetic acid) and Gd-DOTA (DOTA = 1,4,7,10-tetraazacyclododecane-1,4,7,10-tetraacetic acid). Gd-based dendrimer agents for MRI are ‘positive’ contrast agents as compared to iron oxide-based agents which produce a differential enhancement by signal reduction (Barrett et al. 2009). Chiral dendrimer-triamine-coordinated Gd complexes as contrast agents for MRI imaging offer longitudinal relaxivity (r1) 3 times higher than that of clinically used Gd-DTPA (Miyake et al. 2012). PEGylated Gd-loaded dendrimer-entrapped gold nanoparticles (Gd–Au-DENPs) with two radiodense imaging elements AuNPs and Gd(III) in a single system display both CT/MR imaging capabilities with increased circulation times. They were able to image heart, liver, kidney, and bladder of rat or mouse within a time frame of 45 min (Wen et al. 2013). Chen et al. (2013) developed folic acid (FA) conjugated-Gd–Au-DENPs as nanoprobes for targeted CT/MR imaging of cancer cells. Lactobionic acid (LA)-modified dendrimer-entrapped gold nanoparticles (LA-Au DENPs) have been used for in vitro and in vivo targeted CT imaging of human hepatocellular carcinoma. LA-Au DENPs imaging probes were injected both intravenously and intraperitonealy to the mice (Fig. 8). The results suggested that the tumor CT values of the targeted group injected with LA-Au DENPs was much more than the nontargeted group at same time points. Additionally, intravenous injection enabled much more sensitive CT imaging of the tumor model compared to intraperitoneal injection (Liu et al. 2014).
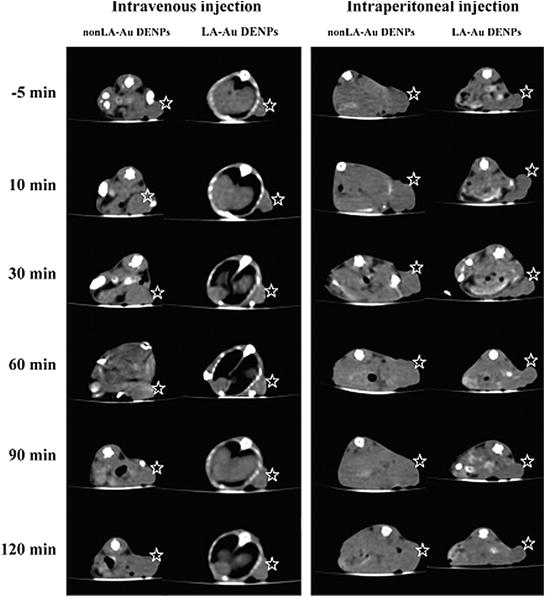
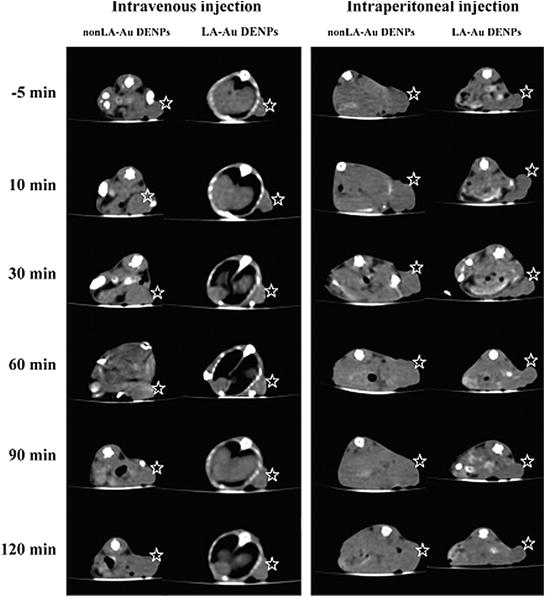
Fig. 8
CT images of the xenografted HepG2 tumor model before and after intravenous or intraperitoneal injection of non-LA-Au DENPs and LA-Au DENPs at different time points post injection. The stars indicate the tumor area. Adapted from Liu et al., with permission from American Chemical Society. Copyright 2014
Biodegradable polydisulfide dendrimer nanoclusters (DNCs) labelled with Gd chelates have been tested as MRI contrast agents. Gd was found to accumulate in various organs of mice including heart, lung, liver, kidney, spleen, and blood 24 h post-injection with the highest uptake observed in the kidney (5.68 ± 2.32 % I.D./g) followed by liver (4.52 ± 1.93 % I.D./g) and then the spleen (2.95 ± 1.36 % I.D./g). Polydisulfide DNCs exhibited a circulation half-life of >1.6 h in mice with considerable contrast enhancement in the abdominal aorta and kidneys for around 4 h. T1-weighted images of kidneys and abdominal aorta showed a significant contrast enhancement after 15 min and 1 h post-injection of Polydisulfide DNCs compared to G3 PAMAM dendrimers (Fig. 9) (Huang et al. 2012).
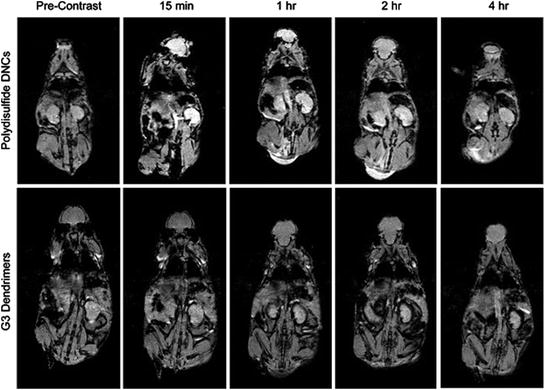
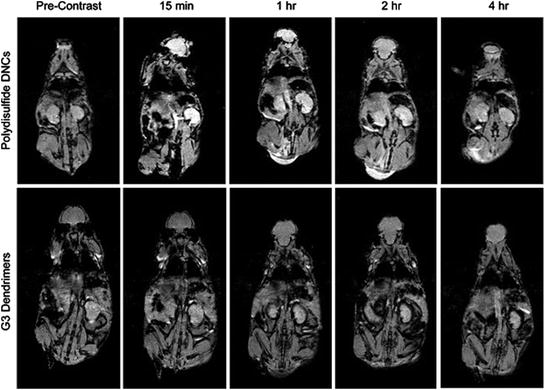
Fig. 9
Magnetic resonance images of mice at various time points after the tail vein injection of polydisulfide DNCs or PAMAM(G3)-[Gd–C-DOTA]. Adapted from Huang et al., with permission from American Chemical Society. Copyright 2012
All the above stated examples clearly implicate that the road towards improvement in efficiency of dendrimer-based contrast agents will increase their availability for clinical applications. Perhaps a new horizon could be combining the molecular contrast agents and therapeutic payloads in a single dendrimer molecule to achieve imaging of malignant tissue for therapeutic benefit.
2.3 Dendrimers as Carriers for Theranostic Agents
The structural versatility and modifiable architecture of dendrimers allows it to be used for simultaneous delivery of imaging and therapeutic agents to the target cells. Owing to multivalence of dendrimers different imaging probes can be attached to the periphery and interior spaces can be used for encapsulation of different anticancer drugs. Chang and co-workers designed FA conjugated poly(ethylene glycol) (PEG)-modified PAMAM dendrimers with paclitaxel (PTX), Cy5.5 fluorophore and superparamagnetic iron oxide (IONPs) (FA-PEG-G3.5-PTX-Cy5.5@IONPs) and studied the in vitro targeting ability towards MCF-7 (breast cancer) cells (Fig. 10).
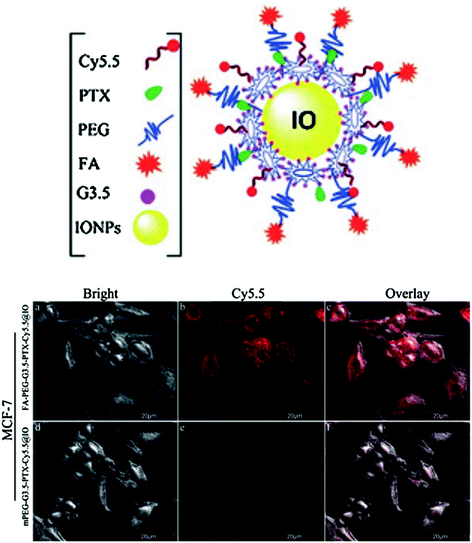
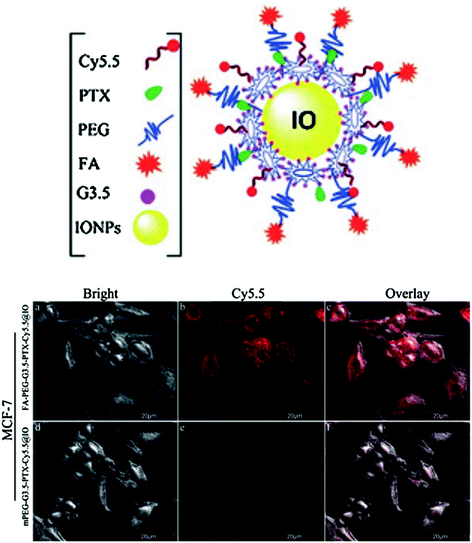
Fig. 10
Digrammatic representation of FA-PEG-G3.5-PTX-Cy5.5@IONPs (upper panel). The in vitro cellular uptake and targeted tumor cell imaging of PTX loaded conjugates. Confocal images show FR positive human breast cancer cell MCF-7 uptake of FA-PEG-G3.5-PTX-Cy5.5@IONPs and mPEG-G3.5-PTXCy5.5@IONPs after 2 h incubation. Adapted from Chang et al., with permission from Royal Society of Chemistry. Copyright 2013
Peptide conjugated PEGylated-polypropylenimine (PPI) dendrimer with numerous hydrophobic pockets can encapsulate photosensitizers such as phthalocyanines (Pc) for fluorescence image-guided drug delivery and noninvasive treatment of deep tumors by photodynamic therapy (PDT) (Fig. 11). Such Pc based theranostic modalities allow fluorescence based imaging of malignant tissue when accumulated and thereafter near infrared (NIR) light can be precisely applied on the detected cancer tissue for PDT leaving the healthy organs untouched (Taratula et al. 2013).
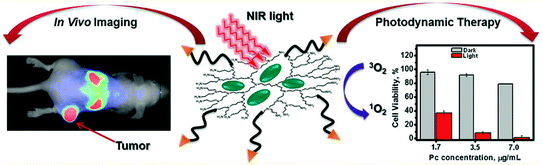
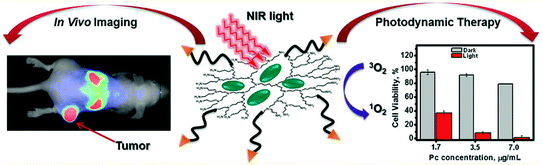
Fig. 11
Schematic representation of tumor targeted theranostic platform based on phthalocyanine-loaded dendrimer for in vivo tumor imaging and PDT therapy. Adapted from Taratula et al., with permission from American Chemical Society. Copyright 2013
Efforts are being laid on development of asymmetric dendritic structures with high structural complexity to provide multiple functionalities for delivery of theranostic agents. Ornelas et al. (2011) synthesized a Janus-like multifunctional dendrimer comprising of 9 azide termini, 9 amine termini and 54 terminal acid groups as next generation of materials for cancer theranostics. Dendrimers can also be linked to other nanomaterials such as carbon nanotubes (CNTs) to improve the drug loading capacity and achieve targeted delivery to cancer cells. In one such attempt, Wen et al. (2013) designed PAMAM G5/FA/FI (fluorescein isothiocyanate)—multiwalled carbon nanotubes (MWCNTs) for targeted and pH responsive delivery of DOX to KB cells over expressing folic acid receptors (FAR). The complexes showed high drug payload and encapsulating efficiency of 97.8 and 67 % decrease in KB cell viability was observed compared to large number of live cells treated with complexes lacking FA ligand suggesting anticancer therapeutic efficacy of these complexes against FAR expressing cells.
Majoros and group reported targeted MTX delivery via FA/FITC conjugated G5 PAMAM dendrimer to epithelial cancer cells (KB) overexpressing FAR (Majoros et al. 2009). Reports exist wherein dendrimer modified imaging nanoprobes have been used for targeted cancer imaging. Aptamer-conjugated PAMAM dendrimer-modified quantum dots have been used specifically for imaging of U251 glioblastoma cells in vitro (Li et al. 2010). By virtue of unique structural characteristics of dendrimers, they have been utilized for design of dendrimer-entrapped metal NPs or dendrimer-stabilized metal NPs for computed tomography (CT)/magnetic resonance (MR) imaging applications. Incorporation of anticancer drug to such modalities can provide the platform for development of multifunctional theranostic agents. Alpha-tocopheryl succinate (apoptosis inducing vitamin E derivative) conjugated multifunctional dendrimer-entrapped gold nanoparticles with FA and FI as targeting entity and imaging probe could act as multifunctional theranostic platform to achieve targeted CT imaging of tumors and therapy (Zhu et al. 2014). All the above stated examples clearly implicate prospective candidature of dendrimers as delivery systems for cancer nanotheranostics. The structural architecture of these nanosized polymeric systems can be fine-tuned according to the therapeutic needs for the clinical benefit. Researchers are trying to deeply understand the pharmacodynamics and pharmacokinetic aspects of drugs associated with dendrimers before entering the clinical trials. Moreover, the biodegradability of dendrimers is still controversial. Upon entering the body and release of therapeutic cargo what is the ultimate fate of dendrimers is still under investigation. Henceforth, still plenty of work needs to be done to develop dendrimers as promising nanotools for cancer theranostics.
3 Porphyrins as Cancer Theranostic Agents
Although various techniques are prevalent in clinical trials for cancer therapy such as surgery, chemotherapy and radiation therapy, etc. Nevertheless cancer therapeutics and diagnostic techniques also benefiting from components that efficiently absorb light (Photonics) includes photothermal and photodynamic therapy (Dolmans et al. 2003; Haung et al. 2006), optical frequency domain imaging (Vakoc et al. 2009), fluorescent and colorimetric detection (Chan and Nie 1998; Storhoff et al. 2004), photoacoustic tomography (also known as optoacoustic tomography) (Wang 2009; Xu et al. 2006), and multimodal techniques (Weissleder and Pittet 2008), etc. Optically active inorganic nanoparticles often interacts strongly with photons thus can be used as agents for above mentioned techniques.
Among the fluorescent probes, quantum dots are valuable and have extinction coefficients in the range of 105–106 M−1 cm−1 (Klostranec and Chan 2006). Gold nanoparticles (GNPs) are also useful for photothermal, colorimetric detection and photoacoustic techniques owing to their much higher extinction coefficients in the order of 109–1,011 M−1 cm−1 (Yguerabide and Yguerabide 1998). Despite being widely studied, they lack extensive medical execution, probably due to lack of concern about the long term safety and drug loading capacity typically being restricted to the nanoparticle surface (Ghosh et al. 2008; Nel et al. 2006). Contrarily, organic nanoparticles (including liposomes, nanospheres micelles, lipoproteins, and polymersomes) have shown several applications owing to their drug delivery potential and robust biocompatibility (Peer 2007). Though, intrinsically their use in biophotonics was limited due to lack of light absorption in the near-infrared region. Although various supramolecular assemblies has been formed entirely by porphyrin conjugates (intensely light-absorbing organic small molecules) though these constructs lacks stability, solubility or biological utility which constraint their use as biophotonic agent (Drain 2009). Hence nanotechnology introduces ‘porphysomes’; organic nanoparticles formed by self-assembled phospholipid-porphyrin conjugates which display liposome like structure and loading capacity, excellent biocompatibility, structure dependent fluorescence quenching, and having high absorption of near-infrared light (NIR), and demonstrate potential for various biophotonic applications (Lovell et al. 2011).
3.1 Porphyrins as Photodynamic Therapy (PDT) Agents
Photodynamic therapy (PDT) is amongst one of the several cancer treatment modalities. PDT is a method involves administration of photosensitizer into the cancer tissue which eventually leads to destruction of cancer by generation of singlet oxygen, a cytotoxic agent (Ethirajan et al. 2011). It encompasses three nontoxic components: a photosensitizer, light, and oxygen (Dolmans et al. 2003). Typically a photosensitizer is defined as chemical entity which upon absorption of quanta induces a physical or chemical modification to another chemical entity. A good photosensitizer must possess high quantum yield (capable of triplet state formation) and high extinction coefficient. Mostly compounds which forms triplet states are capable in generation of reactive oxygen species (ROS) possessing heterocyclic or porphyrin-like ring structures with conjugated π-electron double bond system (Berg et al. 2005). Various articles focuses on several classes of photosensitizers including 5-aminolevulinic acid (Otake et al. 2003) (ALA), phthalocyanines, naphthalocyanines (Allen et al. 2001) and their metallated analogues such as aluminium, cobalt, gallium, silicon and zinc complexes or expanded porphyrins.
Generally all approved and frequently used photosensitizers in cancer therapy (except methylene blue) are tetrapyrrole macrocycle (Dolmans et al. 2003). This macrocycle tetrapyrrole ring structure is named as porphin and various structure derivatives of porphins are named porphyrins. Porphyrin molecule consists of four pyrrole subunits connected with four methine bridges. These are naturally occurring biomolecule pigments which are involved in many biological processes. These molecules are not able to induce any photophysical or photochemical reactions as their fluorescence are rapidly quenched in their normal surroundings, e.g. chlorophyll. Mostly in the tetrapyrroles structure a metal ion is coordinated in the middle of the compounds. Due to the presence of a coordinated metal ion porphyrins have the photo cytotoxic potential based on its electronic properties. These complex compounds becomes efficient photosensitizers by removal of the metal tetrapyrroles, also acquiring fluorescing properties, e.g. exclusion of iron from heme. Hence most proficient porphyrin-based photosensitizers lack coordinated metal ions from complexes. It enhances the possibility for non-radiative decay of the triplet state, paramagnetic metals such as Fe3+ being much more efficient than diamagnetic metals such as Al3+ and Mg2+. Regardless of the fact several metallophotosensitizers have been synthesized for clinical purposes. Even though they have low quantum yields for cell inactivation, they possess other properties such as improved stability and solubility, which sought interest in them as therapeutic substances.
Porphyrins and their structural derivatives have been studied widely as powerful photosensitizers. Their distinctive photophysical and photochemical properties and their presence in natural systems make them an attractive choice for the production of ROS in PDT. Photofrin, one of the commercially existing porphyrin is already used for tumor treatment (Dougherty et al. 1998). There is a great deal of research interest in the exploration of possibility for synthesis of novel porphyrin derivatives for its use in targeted tumor theranostics (Comuzzi et al. 2006). Second most important property of novel photosensitizer is their capacity to absorb at wavelengths in the red region of spectrum, which is most penetratable region of electromagnetic spectrum in living tissues (Das et al. 2010). Besides that, plenty of methodologies for active targeting have been developed to intensify the effectiveness of photosensitizers, eg., their conjugation to antibodies or peptides. Various PDT agents have also been incorporated into several polymers to yield higher local concentrations at specific target sites (Konan et al. 2003).
In recent past a study came up with exploration of quantitative, unquenched activatable photosensitizers (QUaPS) based on porphyrins molecules as shown in Fig. 12. This method exhibit potential for latest scientific scenarios based on molecular feedback-oriented PDT approach to confirm that the target area were well treated with appropriate photosensitizer dose and light. Here porphyrins were used as caspase receptive Forster Resonance Energy Transfer (FRET) acceptors to organic 5-Carboxy-X-Rhodamine (Rox) fluorophore donors. The method relies on usage reactive photosensitizers that can induce both cell death (via ROS) and observe it (via caspase identification) respectively as shown in Fig. 13. Light of higher wavelength could be used to permit for deeper light infiltration into the tissues of interest where QUaPS and porphyrin as red-shifted fluorescence donors and acceptors respectively was used. More effective photosensitization in HT-29 cancer cells were practiced based on unquenching of ROS earlier to caspase activation when compared to porphyrin FRET donor constructs. This large dynamic construct enabled ratio metric sensing (with Rox excitation) via caspase activation in particular cells followed by induction of cell death (with Pyro excitation) using a single construct as shown in Fig. 14. Thus the minimal amount of light could be used by execution of PDT unless QUaPS got activated, that kept target cells into an apoptotic stage (contrarily to nonapoptotic or necrotic) (Lovell et al. 2011a).
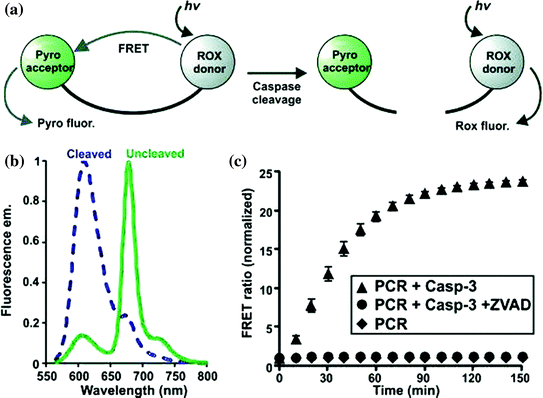
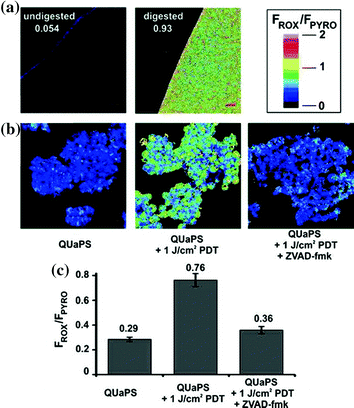
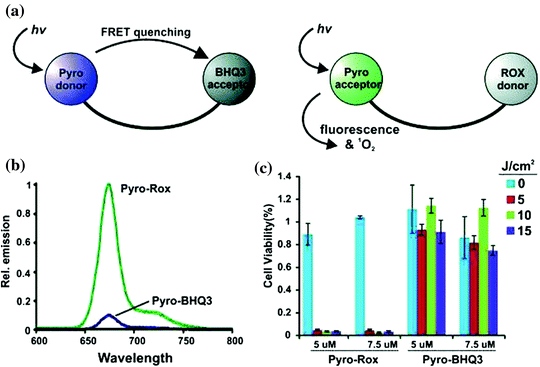
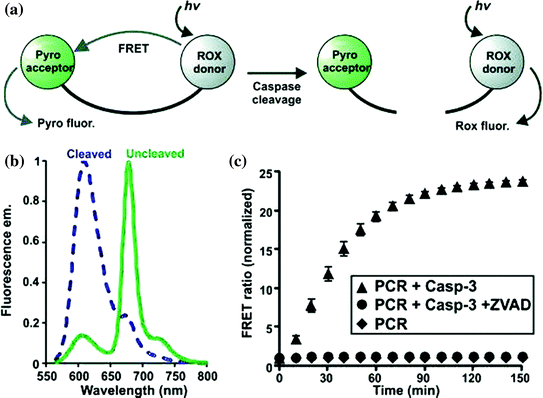
Fig. 12
Ratiometric sensing using QUaPS. a Schematic representation of FRET response to caspase cleavage. b Fluorescence emission spectra of QUaPS before and after proteolytic cleavage. c Normalized FRET ratio change in response to recombinant Caspase-3 with or without ZVAD-fmk, a caspase inhibitor. Adapted from Lovell et al., with permission from American Chemical Society. Copyright 2011
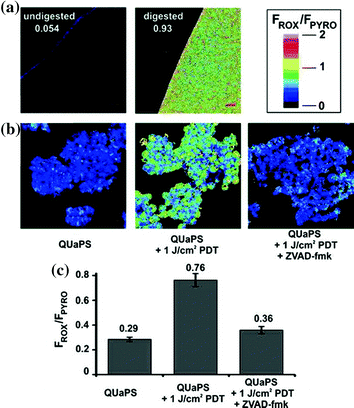
Fig. 13
QUaPS as an inducer and ratiometric sensor of caspase activation. a Fluorometric confocal microscope image of a 2 μM QUaPS solution, with or without proteolytic digestion. Numbers represent the Rox:Pyro average intensity ratio for the solution. FRET ratio legend is indicated. Scale bars represent 20 μm. b Induction of cell death and ratiometric imaging of caspase activation. Cells were incubated with 5 μM QUaPS and treated as indicated. Confocal microscopy was used to image the cells 2 h later. c Single cell analysis of caspase activation. Error bars show mean ± std. dev. for 30 cells for each group from 3 separate experiments. Adapted from Lovell et al., with permission from American Chemical Society. Copyright 2011
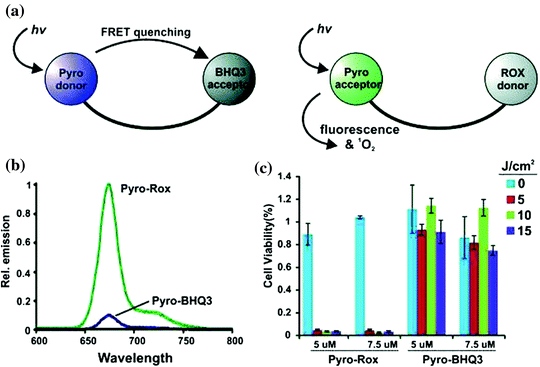
Fig. 14
Increased brightness and photosensitization of QUaPS compared to a Pyro donor aPS. a Differences between FRET donor or FRET acceptor aPS. b Fluorescence of 250 nM peptide linked Pyro-ROX (Pyro-acceptor) and Pyro-BHQ3 (Pyro-donor). c PDT efficiency in HT-29 cancer cells of peptide linked Pyro-ROX and Pyro-BHQ3. Adapted from Lovell et al., with permission from American chemical society. Copyright 2011
3.2 Porphyrins as Photo Thermal Therapy (PTT) Agents
Interest in photonics for detection and treatment of cancer has developed parallely with advancement in light technology, including the consideration of the optical properties of tissues, and the expansion of optical probes. The medical purpose of photonics comprises both therapeutic and diagnostic intervention. Among various emerging cancer treatment modalities, photo thermal therapy (PTT) is well known for the controlled generation of heat which will be further used to ablate diseased tissues (Jori et al. 1990). The phenomena are based upon generation of heat via the interaction of laser light with photo thermal agents, which leads to the switching of light into heat. Excessive off-target damage was prevented by excellent spatial control due to localized heating given to the areas into which the Photothermal therapy (PTT), agent and applied light overlap (Welch 1984). PTT, a hyperthermia based cancer therapeutic methodology, has become one of the research interest due to its minimal invasiveness, reasonable penetrating depth, and good controllability as shown in Fig. 15 (Chen et al. 2005, 2007). With the help of photo-absorbing agents, near-infrared (NIR) light penetrating into the tumor region can be converted into heat for specific ablation of cancer cells without damaging nearby healthy tissues. Another method for controlling PTT damage is via the directed application of light. Light is applied typically through laser fibres for PTT. Proper positioning of the laser fibers is of the principle importance to maximize the deposition of energy in target tissues while minimizing off target heating.
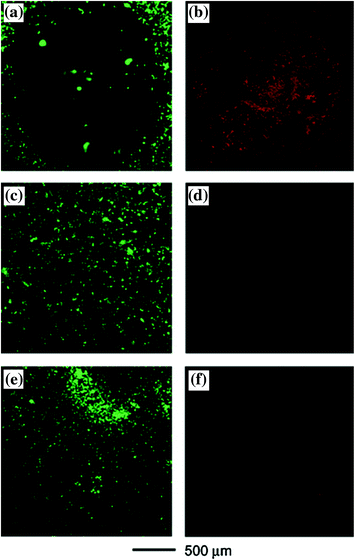
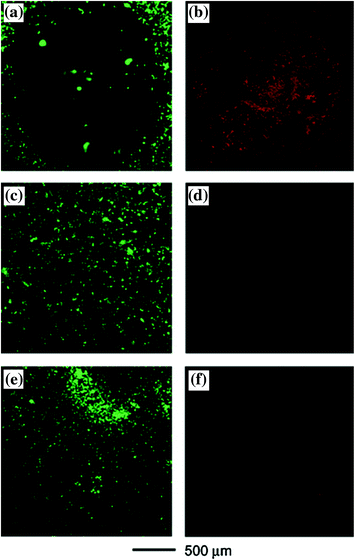
Fig. 15
SK-BR-3 breast cancer cells that were treated with immuno gold nanocages and then irradiated by an 810 nm laser at a power density of 1.5 W/cm2 for 5 min showed a well-defined circular zone of dead cells as revealed by: a calcein AM assay (where green fluorescence indicates the cells were live), and b ethidium homodimer-1 (EthD-1) assay (where red fluorescence indicates the cells were dead). In the control experiment, cells irradiated under the same conditions but without immuno gold nanocage treatment maintained viability, as indicated by c calcein fluorescence assay and d the lack of intracellular EthD-1 uptake. Cells treated with immuno gold nanocages but irradiated at a lower power density (0.5 W/cm2) for 5 min remained alive, as shown by e calcein fluorescence assay and f the lack of intracellular EthD-1 uptake. Adapted from Chen et al., with permission from American Chemical Society. Copyright 2007
In the area of nanomedicine PTT has received attention seeking to exploit its effect. Majority of the PTT agents explored to date are based on inorganic nanomaterials including gold nanoparticles (Huang et al. 2006) [e.g. nanocages (Cobley et al. 2010), nanorods (Maltzahn et al. 2009), nanoshells (Melancon et al. 2008), and nanospheres (Lu et al. 2011)]. Although gold nanoparticles (GNPs) have excellent photo thermal efficiency and photo stability but lack of biodegradability leads to concerns about their long-term fate and safety in vivo (Khlebtsov and Dykman 2011). GNPs are proficient in photo thermal conversion due to the high absorption cross section of NIR light and surface plasmon resonance (SPR) oscillation (Huang et al. 2008). Along with inorganic several organic chromophores have also been developed as PTT agents (Jori et al. 1990) eg., endogenous chromophores in tissue (Chen et al. 1995) externally added dyes such as indocyanine green (Yu et al. 2010), naphthalocyanine (Camerin et al. 2005), and transition-metal-coordinated porphyrins (Soncin et al. 1996). PTT capability of monomeric chromophores is limited by their low light absorption (ε = 104–106 M−1 cm−1) compared to GNPs with higher extinction coefficients (ε = 109–1011 M−1 cm−1) (Yguerabide et al. 1998). An alternative option is porphysomes: a biodegradable and non-toxic organic nanoparticle with photo thermal properties comparable to that of gold (no toxicity in mice up to 1000 mgkg−1) (Jin et al. 2011). Nevertheless porphysomes get potentially photobleach under irradiation, which is among one of the major concern for PTT (Huang et al. 2008). Therefore the improvement of photostability of porphysomes would be of benefit to porphysome related PTT therapy. Porphyrins have also been conjugated to gold nanoparticles or carbon nanotubes for enhanced light energy conversion and combination of photodynamic and photothermal therapy (Jang et al. 2011).
3.3 Porphyrins as Bioimaging Agents
Porphyrins have ability of forming highly stable metallocomplexes based on their excellent metal chelation ability (Ali et al. 1999). Various reports came up with the potential use of metal-porphyrin complexes for the detection of cancer in patients and shown tumor-avid activity in vitro and in vivo to found their ways into clinical studies (Fazaeli et al. 2011). A boronated porphyrin known as BOPP containing 40 atoms of boron-10 per molecule against one atom alone in the borophenylalanine (BPA) molecule was used in boron-neutron capture therapy (Kahl and Koo 1992). There are various types of radiolabelled porphyrins, 109Pd-protoporphyrins (Fawwaz et al. 1994), 109Pd-porphyrins (Fawwaz et al. 1971), 109Pd-derivitized porphyrins (Chakraborty et al. 2007), and 188Re-porphyrins (Sharma et al. 2010) available for imaging purpose. With the increasing trend in the synthesis and utilization of PET radionuclides for nuclear medicine has offered new breakthrough for researchers to focus on the fabrication of new 6 Ga-radiopharmaceuticals. In recent past a 67 Ga-porphyrin complex was synthesized with attractive biological properties for tumor bio imaging, but at the same time 67 Ga-complex demonstrated higher accumulation in liver due to its low hydrophilicity (Fazaeli et al. 2011). For instance, 99mTc-porphyrin conjugate has been evaluated in rodent mammary tumors (Murugesan et al. 2001). Recently 99mTc-porphine was developed for imaging despite its high hepatotoxicity (Wang et al. 2010). At the same time kinetic studies for 111In incorporation into m-tetraphenylporphine have been conducted, and biological application has been seen (Nunn 1978).
Past over half a century ago a study showed the use of 64Cu-porphyrin complex for PET imaging (Bases et al. 1958). The simple and stable chelation of 64Cu affirm further study of other radioisotopes more appropriate for clinical PET imaging studies that could also form highly stable metalloporphyrin complexes such as 60Cu and 62Cu (Williams et al. 2005). Based on the multifunctionality of porphyrins and the efficient and stable encapsulation of 64Cu, this idea of first developing a porphyrin-based optical theranostic probe with brilliant in vivo tumor targeting individuality and then exchange it a targeted nuclear imaging probe through chelation of a radioisotope can be converted further to any targeted porphyrin-based agent. Recently this field of radio-metalloporphyrin got revitalized with the same concept of strong metal chelation complex between Cu-porphyrin, by demonstration of 64Cu-PPF (porphyrin-peptide-folate (PPF)). In this study the PKM linker (pharmacokinetics modifying linker) act as a targeted PET imaging probe for FR (folate receptor) positive tumors while retaining its favorable characteristics such as pharmacokinetics, biodistribution and selective tumor uptake (Shi et al. 2011). The utilization of 64Cu-PPF may be employed for forecast and quantitative measurements of photosensitizer deposition into the tumors tissues to further aid in treatment planning and monitoring of PDT treatments.
In another study SERS based imaging tool was developed as both the Raman dye and a biocompatible surface coating agent on gold nanoparticles surface by the use of a porphyrin−phospholipid conjugate with quenched fluorescence. By the simple synthesis and validation with spectroscopic and microscopic techniques, the study showed porphyrin−lipid stabilized GNPs are a novel SERS probe effective for cellular imaging as shown in Fig. 16. To date, this was the first study on utilization of porphyrin as a Raman reporter molecule for SERS based imaging (Tam et al. 2012).
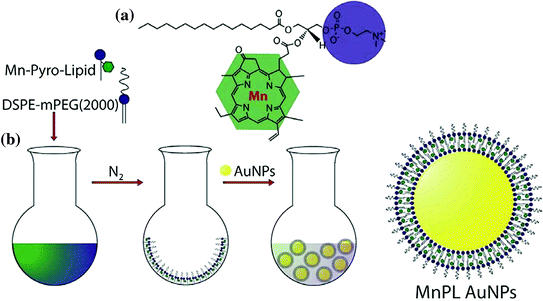
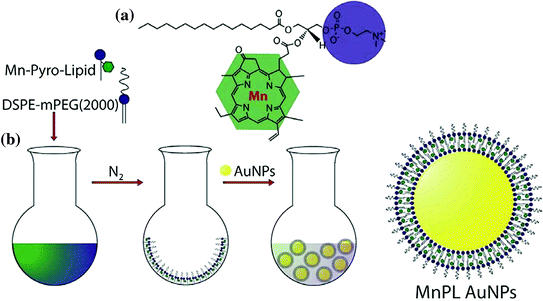
Fig. 16
Scheme 1 Structure of Manganese Pyro-Lipid (MnPL) (a) and three-step procedure for Creating SERS AuNPs with MnPL (b). Adpated from Tam et al. 2012, with permission from American Chemical Society. Copyright 2012
Magnetic resonance imaging (MRI) is one amongst various imaging modalities, is particularly attractive due to its advantages of noninvasive, high spatial resolution and three-dimensional imaging (Feshitan et al. 2012; Sanson et al. 2011). Additionally, the MRI sensitivity can be enhanced by the utilization of contrast agents. Paramagnetic Gd3+ complexes are clinically favorable MRI contrast agents due to their high contrast ability (Zhang et al. 2010; Hartman et al. 2008). However, the renal toxicities of widely used Gd3+ complexes have raised our concerns (Sieber et al. 2008).
In the quest for synthesis of synergistically improved theranostic agent for cancer therapy, a multicomponent and multifunctional DOX@PLA@Au-PEG-MnP NPs which could operate as an intelligent nanotheranostic agent has been successfully synthesized (Jiang et al. 2014). This multifunctional nanoparticle consists of different components, gold nanoshell as NIR photoabsorber, comprising PLA as biodegradable drug carrier for performing PTT and trigger an instant drug release, MnP (Mn-porphyrin) acts as T1 contrast agent for enhancement of MR imaging, and PEG was used for prolonging the circulation time in vivo, into a single agent. Through various in vitro and in vivo experiments, they demonstrated that DOX@PLA@Au-PEG-MnP NPs exhibited a greatly improved longitudinal relaxivity (r1 value of 22.18 mM−1 s−1 of Mn3+), facilitating to capture the location and detailed structure of the tumor via MRI. Upon irradiation of NIR laser, the composite agent triggered DOX release and exhibited excellent PTT therapeutic potential resulting in synergistic therapeutic effect of cancer in both cellular experiments and tumor-bearing nude mice model. It was found that the combined effect of DOX and PTT were more cytotoxic than either of their treatment alone. NIR laser light was used as a noninvasive source for hyperthermia, which overcome the limitations of other types of hyperthermia like radiofrequency (rf) ablation or microwave ablation, which required antenna insertion or interstitial needle. Therefore an intelligent nanotheranostic agent was fabricated as DOX@PLA@Au-PEG-MnP NPs, which could be very useful for accurately visualizing the location and size of the tumor, also for monitoring the therapeutic process, along with the synergistic treatment of cancer (Jiang et al. 2014).
MRI imaging with molecular probes are important tool to diagnose physiological parameters with reasonably high spatial and temporal resolution in living tissues. Generation of cell-permeable imaging agents for detection of intracellular analytes remains a challenge. In recent past, a study came up with construction of metalloporphyrins based on intrinsically membrane-permeable platforms which constitute an effective alternative to probes formed by conjugating contrast agents to specific cell delivery vehicles. It permits analysis of neural structures in living mammalian brains by getting penetration inside the cells in tissue. Recently, an investigation showed that in the paramagnetic metalloporphyrin a MRI molecular imaging agent Mn-(DPA-C2)2-TPPS3 (Mn-TPPS4 analog containing dipicolylamine (DPA) groups) efficiently binds to labile Zn2+ ions, and deeply penetrates inside the cells thus allow it to stains brain tissue in intracranially injected rats persistently. Mn-(DPA-C2)2-TPPS3 were designed for zinc ions sensing, and more pronounced contrast enhancement were there in the hippocampus, (a zinc-rich brain region) than in the other part the caudate nucleus, which contains comparatively little labile Zn2+ ions. In addition to MRI, direct visualization of its distribution by histology was permitted by chromogenicity of the probe. Allocation was concentrated in cell bodies after hippocampal infusion. Excellent functionality for in vivo imaging was offered based on high relaxivity of porphyrin-based contrast agents and membrane permeability (Lee et al. 2010).
In another study, an effort has been made to design a novel theranostic nanoplatform for photodynamic therapy. The click chemistry method was adopted for synthesis of nanoconjugate superparamagnetic iron oxide nanoparticle–porphyrin (SPION-TPP). Upon exposure of light SPION-TPP nanoconstructs promote a PDT effect in vitro in murine amelanotic melanoma B78-H1 cells, with IC50 values in the region of 800 nm. Nevertheless, SPION-TPP nanoconstructs shows reduced cellular uptake, which affect a linear dose–response effect. Conjugation of a well-known cell-penetrating peptide (TAT peptide) to the SPION-TPP nanoparticles leads to the improvement of delivery to cells has also been studied. At lower IC50 values (in the region of 500 nm) the new nanoconstructs showed a distinct dose–response effect. This study suggested that TAT-conjugated SPION-TPP nanoparticles could be an effective nanostructure for tracking drugs by use of MRI-based techniques and for treating cancer cells through PDT, thus functioning as potential theranostic agents (Thandu et al. 2014).
Recently a study showed generation of “two-in-one” magnetic-fluorescent nanocomposites comprising silica-coated magnetite nanoparticles, which get covalently attached to a porphyrin moiety. In the presence of PMNC, initial co-incubation experiments were performed using THP-1 macrophage cells which showed a distinct photobleaching of the porphyrin upon irradiation of light under a fluorescent microscope (Nowostawska et al. 2011).
3.4 Porphyrin Based Nanoparticles (Porphysomes)
The ideal nanomedicine formulation includes design of novel materials for drug delivery application. Designing multicomponent and multifunctional nanoparticles via molecular self-assembly involves control upon nanoparticle size, surface chemistry and geometry, it is especially useful in a process whereby molecules self-associate in solution via weak intermolecular forces (Huynh and Zheng 2013). The concept of activatable nanoparticles can be executed by coupling dynamic structural changes with photophysical phenomena that occur at the nanometer length scale. External stimuli leads to activation of these “smart” agents which further elicit an effect at the nanoscale level. These activatable nanoparticles that get activated by external stimulus played useful role in PDT and photodiagnosis. The specificity of action depends on preferential accumulation of the photoactive agent into target versus normal tissues. Thus additional layer of specificity can be included by activating the agent only in the target tissues into the treatment process.
Porphysomes represents the self-assembled form of porphyrin lipid conjugate into liposome-like nanoparticles (100 nm diameter). The packing density of porphyrin per particle (>80,000 per particle) is high, so their light absorption efficiency is extremely high. Induction of highly self-quenching porphyrin excited states due to the packing density, their absorbed energy will be released as heat, making them exceptionally useful as PTT agents. Unlike monomeric porphyrins, this nanoassembly of porphyrin lipids (Porphyrosome) serves as ideal for PTT enhancement rather than usual PDT agent. Recently a study evaluated the mechanism of nanostructure-driven conversion of the PDT activated porphyrin photosensitizers to activated PTT transducers by performing in vivo studies. It involves the PDT and PTT efficiency of porphysomes for treatment of hyperoxic and hypoxic tumors. This was the first unique study which showed the conversion of porphyrins from PDT to PTT, by comparing porphyrin nanoparticles with porphyrin monomers in vivo. Porphysomes exhibit fluorescence quenching and photothermal properties when formulated mechanically (Jin et al. 2013). This fluorescent lipid conjugated vesicle was capable of ROS generation, and also stably chelate metals for radioimaging applications (Liu et al. 2012). Thus it showed for the first time about the usage of organic PTT agents with comparable optical absoprtions to GNPs for high photothermal efficiency (Lovell et al. 2011).
Nanoparticles (100–200 nm size range) could accumulate in tumors tissues through the enhanced permeation and retention (EPR) effect, but depth of penetration gets affected by the local tumor environment (Jain et al. 2010). In recent past a study have shown that nanoparticles less than 40 nm may be more effectively penetrates deep into the fibrous tumors than their larger counterparts (Pluen et al. 2001; Cabral et al. 2011). The two major difficulty in the design of novel nanoparticles comprises the abilities to deliver and thereafter activation of therapeutic agent at the intended site of action. Deprived tumor permeability due to densely microenvironment around it could impede the delivery of nanoparticles at the target site of action. Synthesis of tiny porphyrosome for better outcome remains a challenge due to growing instability as a result of surface curvature. In an effort to conquer these shortcomings, activatable porphyrin nanodiscs were formulated by complexing apolipoproteins to pyrolipid using the detergent dialysis method. The design of smart biophotonic nanoparticle was done by self-assembly from aggregated porphyrin lipid and nanoconjugate which was stabilized by an amphipathic alpha helical protein and obtain photoactivation once its organization gets disturbed. Conversion of the particle from a disc to a vesicle-shaped structure was done by enzymatic cleavage method of the protein and provides further confirmation that the apolipoprotein serves a practical role on the nanodisc. Thus successfully nanodiscs varying from 10 to 30 nm in size were synthesized and had a disc-like morphology when compared with the larger porphysomes. Further disruption of the nanodisc structure increased both the fluorescence and the singlet oxygen generation in the discs, and the utility of this nanoparticle was demonstrated in an in vitro model. Diffusion properties of the nanodiscs in a collagen-rich environment showed potential improvement in penetration in permeable tumors (Kenneth et al. 2013).
4 Carbon Dots: Introduction
Carbon dots (C-dots) are the newly discovered fluorescent nanomaterials that constitute a new class of nanocarbon family with quasispherical shape and sizes below 10 nm. C-dots have occupied the centre stage for fluorescence related applications due to their size, cost and abundant nature. Carbon is generally a black material and has low solubility and fluorescence. On the other hand, C-dots have strong fluorescence and due to this reason they are sometimes referred to as ‘fluorescent carbon’. The existence of C-dots came to light when researchers were trying to purify single-walled carbon nanotubes (SWCNTs) through preparative electrophoresis in 2004. Accidently, they observed a fast-moving band of highly luminescent carbonaceous material which was certainly not the SWCNTs they were looking for (Xu et al. 2004). Typically, C-dots display some unique properties which include size and excitation dependent emission, multicolour emission, strong fluorescence, broad excitation spectra, high quantum yield and longer fluorescence lifetimes. These features have attracted the use of C-dots for a host of prospective applications, such as sensing, photocatalysis, optoelectronics and energy storage (Baker et al. 2010). In contrast to traditional metal based semiconductor quantum dots (QDs) and organic dyes, C-dots exhibit some remarkable features in terms of being environmentally and biologically benign, high aqueous solubility, colloidal stability, high photostability, ease of surface functionalization and chemical inertness. Consequently, C-dots are gaining grounds for biological applications and emerging as the most sought after alternative to QDs for similar fluorescence related applications. This area is developing fast, with a considerable number of breakthroughs taking place in the recent years. The first complete study regarding the origin and fluorescence of C-dots was provided by Sun et al. (2006). They employed oligomeric species for rendering effective passivation of the dot’s surface, resulting in bright fluorescence emissions (Fig. 17). It was thus found that surface passivation has implications for C-dots to have strong fluorescence.
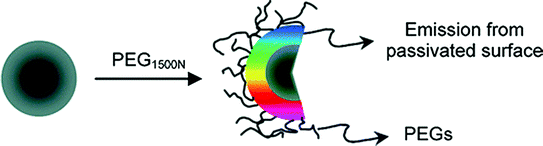
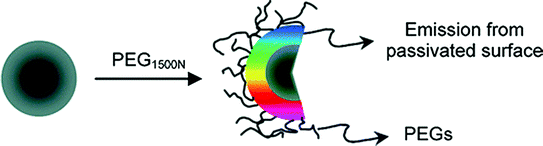
Fig. 17
C-dots with PEG1500N species attached to the surface for passivation. Adapted from Sun et al., with permission from American Chemical Society. Copyright 2006
However, there have been reports of non-passivated C-dots exhibiting fluorescence, but they generally have low quantum yields. The synthetic approaches for C-dots can be categorized into top–down and bottom–up approaches. Top–down approaches include arc discharge, electrochemical and laser ablation which involve the breakdown of chunks of carbon structure, which are quite complicated processes and require energy-consuming devices (Baker and Barker 2010). In comparison, the bottom–up approaches are inexpensive and involve the synthesis of C-dots from molecular precursors by means of chemical reactions like microwave (Sachdev et al. 2013), solvothermal (Mitra et al. 2013) and hydrothermal (Sachdev et al. 2014; Yang et al. 2014) treatments. In addition, C-dots may come from a variety of unconventional sources such as organic matter, natural products such as orange juice, soyabean etc. (Li et al. 2013; Liu et al. 2007; Sahu et al. 2012). This in turn suggests the simplicity and generality of preparative protocols for C-dots synthesis. There are abundant reports depicting one-step synthesis of C-dots through simple kitchen chemistry techniques. For instance, Palashuddin et al. (2012) demonstrated the presence of amorphous carbon nanoparticles of 4–30 nm size by heating daily food items such as bread, jaggery, cornflakes, or biscuits which exhibited photoluminescent emissions. In another study, the same group reported a facile method of synthesis of C-dots using a commercially available induction coil heater by using an aqueous solution of citric acid and a diamine compound (Palashuddin et al. 2014). Generally, the synthetic strategies for C-dots involve the use of a single precursor that acts as both carbon source and a passivating agent or separately employing a passivating agent in addition to carbon precursor. Wang et al. reported the synthesis of C-dots from glycerol with the aid of small amount of an inorganic ion (phosphate solution) without any surface passivation reagent (Wang et al. 2011). A rapid, one-step microwave mediated method for synthesizing C-dots using poly(ethylene glycol) (PEG) as a carbon source as well as a passivating agent has also been reported (Jaiswal et al. 2012). Yang et al. (2014) employed hydrothermal method to synthesize nitrogen-doped C-dots using ammonium citrate, serving as both carbon source as well as meeting the requirements of surface passivation. The groups on the surface of N-doped CDs acted as a self-passivation layer. Polyethylene glycol (PEG), polyethyleneimine (PEI), poly(ethylenimide)-co-poly(ethyleneglycol)-co-poly(ethyl-enimide) (PPEI), 4,7,10-trioxa-1,13-tridecanediamine (TTDDA) are some of the commonly employed agents for surface passivation. Nevertheless, out of the above, the attachment of nitrogen containing moieties onto the surface of C-dots has been found to generate stronger fluorescence emission. Peng et al. (2009) prepared C-dots by dehydration and oxidation of carbohydrates with sulphuric acid and nitric acid respectively, which were weakly emissive. Quantum yield increased to 13 % after passivation with TTDDA. Sachdev et al. (2013) demonstrated a novel one-step method for synthesizing C-dots using chitosan as a carbon source and PEG as a passivating agent through microwave mediated reaction, but the reported quantum yield was lower. In a study of similar relevance, the same group made a comparative analysis between PEG and PEI passivated C-dots synthesized by one-step hydrothermal method, without any post-synthetic treatment (Sachdev et al. 2014). Consequently, the quantum yield of C-dots synthesized by hydrothermal treatment was more compared to those synthesized by microwave treatment. These results suggest that the synthesis of brightly fluorescent C-dots is linked to the selection of right synthetic method along with the passivation polymer. Another interesting scheme of improving the fluorescence emission from C-dots involves the surrounding of C-dots by a metal-containing shell or its association with a metal-based nanostructure. For example, core carbon nanoparticle surface was doped with inorganic salts (ZnO, ZnS, or TiO2) along with the organic functionalization (Baker and Baker 2010; Luo et al. 2013). The resulting C-dots (CZnO-dots, CZnS-dots, or
-dots) exhibited much brighter fluorescence emissions than their undoped counterparts. All the above investigations point out that C-dots contain a carbon nanoparticle core and surface passivation is responsible for tuning the fluorescence performance. The most widely accepted emission mechanism in C-dots is the radiative recombination of surface-confined electrons and holes. Surface passivation schemes make surface sites more active and emissive to facilitate effective recombinations (Sachdev et al. 2013; Sun et al. 2006).

4.1 C-Dots as Bioimaging Agents
Ever since its inception, C-dots have been explored extensively for fluorescence based bioimaging applications. In the current scenario, C-dots are widely being pursued as bioimaging agent as they fulfil the essential attributes of an ideal bioimaging agent. This includes excitation dependent emission behaviour, resistance to photobleaching, aqueous solubility, non-blinking, longer fluorescence lifetimes (nanoseconds) and multicolour emission. Multicolour C-dots that emit in the entire visible spectrum including the near infrared region (NIR) are desirable candidates for bioimaging. It is pertinent to mention that NIR emission of C-dots is particularly noteworthy for in vivo imaging, because of lesser autofluorescence and tissue transparency in the NIR region. Sun et al. (2007) reported the plausibility of C-dots as bioimaging agents for the first time for in vitro and in vivo applications.
4.1.1 Cellular Imaging In Vitro
The biocompatibility and absence of any undesirable effects on cell morphology and physiology encourage further application for in vitro applications. Sun et al. (2006) provided the first ever evidence of biolabelling by incubating E. coli bacteria with surface passivated C-dots and observed multicolour fluorescence by changing the excitation wavelength (Fig. 18).
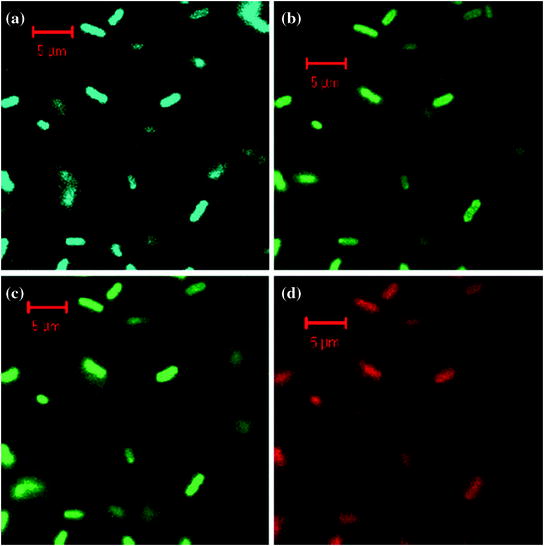
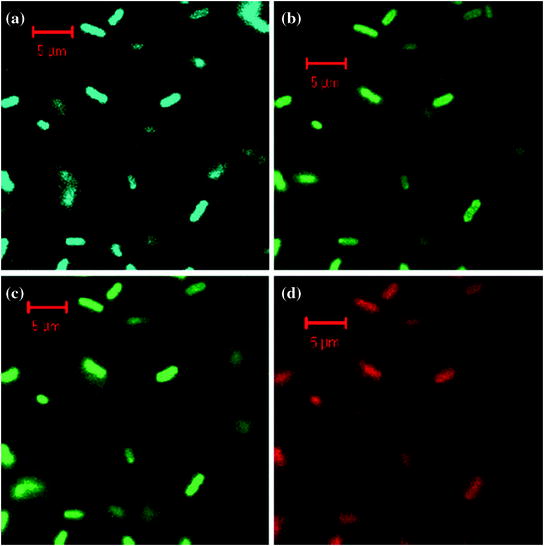
Fig. 18
Confocal microscopy images of E. coli ATCC 25922 cells labeled with the C-dots: a λ EX = 458 nm, detected with 475 nm long pass filter; b λ EX = 477 nm, detected with 505 nm long pass filter; c λ EX = 488 nm, detected with 530 long pass filter; and d λ EX = 514 nm, detected with 560 nm long pass filter. Adapted from Sun et al., with permission from American Chemical Society. Copyright 2006
Similarly, biolabeling mechanism of PEG passivated multicolour C-dots (CPs) was studied using Gram-positive S. aureus and Gram-negative recombinant green fluorescent protein (GFP)-expressing E. coli as model systems. CPs labelled GFP E. coli and S. aureus cells radiated light green, blue and red colour under various fluorescence microscopy filters. The study further established the cellular uptake and labelling time of CPs. Time-dependent microscopy using GFP E. coli bacteria further predicted optimal labelling time of 3 h for multicolour emission. Transmission electron microscopy (TEM) images provided evidence of cellular uptake of CPs inside bacterial cells at a concentration of 0.1 mg/mL without any bactericidal effects. Subsequently, C-dots were pursued for multiphoton bioimaging in mammalian cells such as MCF-7 cells (Fig. 19). PPEI-EI passivated C-dots were found to be strongly emissive under fluorescence microscope following excitation by 800 nm laser. The cellular uptake of C-dots was restricted to the cell membrane and the cytoplasm of MCF-7 cells, without any evidence of nuclear labelling (Cao et al. 2007).
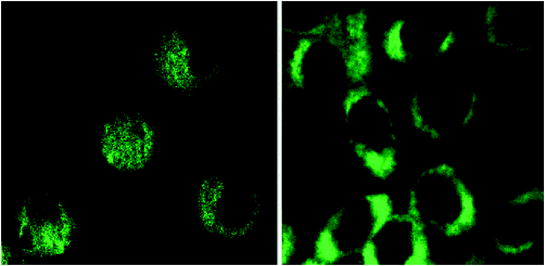
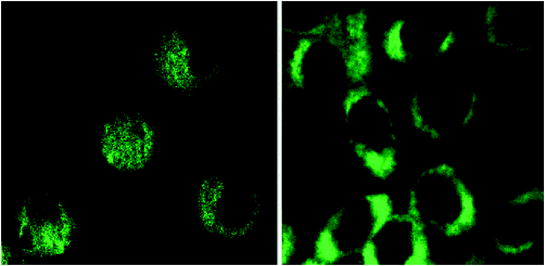
Fig. 19
Representative two-photon luminescence image (800 nm excitation) of MCF-7 cells with internalized C-dots. Adapted from Cao et al., with permission from American Chemical Society. Copyright 2007
In order to explore the effect of surface passivation on cellular uptake of C-dots, these were passivated by two kinds of polymers, including PEG and PEI. Fluorescence microscopy images showed that PEI functionalized C-dots were more efficiently internalized inside A549 and BHK-21 cell lines than PEG functionalized C-dots. The study clearly stated that the surface functionality to a greater extent influences the bioimaging efficiency of C-dots (Sachdev et al. 2014). Most of the available reports on cell imaging applications, suggest that C-dots are mostly localized in the cytoplasm. However, there have been very few reports on nuclear uptake of C-dots. In one such report, cellular localization of C-dots within the nucleus was observed. The internalized C-dots were further able to selectively stain the nucleoli, thereby achieving organelle selection. The difference of fluorescence emission between the cytoplasm and nucleus was clearly visible, suggesting the nuclear uptake (Kong et al. 2014). C-dots have also been used for targeting and detecting cancer cells. Lee et al. developed an aptamer-conjugated imaging probe for targeting cancers. Thiol-terminated C-dots (SH-gC-dots) were conjugated with maleimide-terminated TTA1 aptamer targeting Tnc proteins (TTA1-C-dots). In order to evaluate the ability of TTA1-C-dots for cancer targeting, cancer cell lines, HeLa cells (human cervical cancer cell lines) and C6 cells (rat glioma cell lines) and normal healthy cells, CHO cells (Chinese hamster ovary cell lines) were selected. TTA1-C-dots exhibited higher targeting efficiency compared with SH-gC-dots in HeLa and C6 cells. Increased fluorescence emission intensity for HeLa and C6 cell lines treated with TTA1-C-dots indicated enhanced targeting with respect to the SH-gC-Dots. However, in CHO cells, no detectable difference in fluorescence intensity was observed between TTA1-C-dots and SH-gC-dots (Lee et al. 2013). Song et al. (2012) reported the synthesis of folic acid conjugated fluorescent carbon nanodots (C-dots–FA), which are endocytosible by the overexpressed folate receptor (FR) molecule. The assembly was able to distinguish folate positive HeLa cancer cells from folate negative MCF-7 cancer cells. Additionally, HeLa cells produced bright fluorescence in comparison to normal NIH-3T3 cells due to lower expression of FR receptor in normal cells, resulting in lesser uptake of C-dots–FA. This clearly indicates that the C-dots–FA system could discriminate FR-positive cancerous cells from normal cells. The above reported studies highlight that C-dots are amenable to various cell imaging applications without the use of any complicated protocols.
4.1.2 In Vivo Imaging
C-dots have exhibited the same or even better performances for in vivo imaging in mice models compared to commercially available CdSe–ZnS QDs for optical imaging applications (Cao et al. 2012). The use of C-dots and CZnS-dots passivated by PEG1500N for in vivo mice imaging was first ever reported by Sun’s group (Fig. 20). Upon subcutaneous injection, mice exhibited bright emissions upon excitation of 470 and 545 nm due to uptake of C-dots and CZnS-dots.
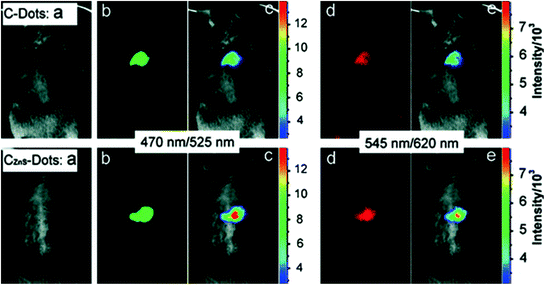
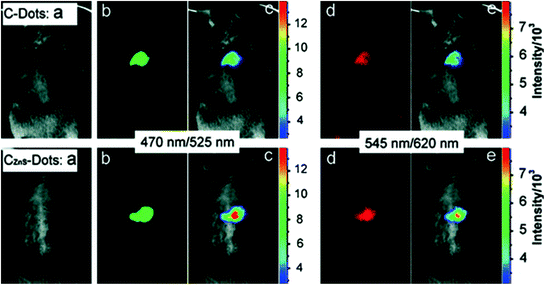
Fig. 20
Subcutaneous injection of (top) C-dots and (bottom) CZnS-dots: a bright field, b, d as-detected fluorescence (excitation/emission wavelengths indicated), and c, e color-coded images (Image from NIH). Adapted from Yang et al., with permission from American Chemical Society. Copyright 2009
Taking advantage of bright green fluorescence of CZnS-dots, they were further employed for tracking the migration through lymph nodes. The harvested auxiliary lymph nodes were dissected after 24 h post injection and exuded appreciable fluorescence (Fig. 21).
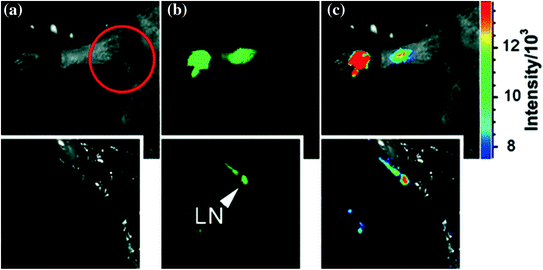
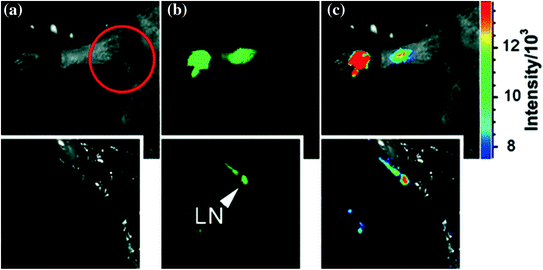
Fig. 21
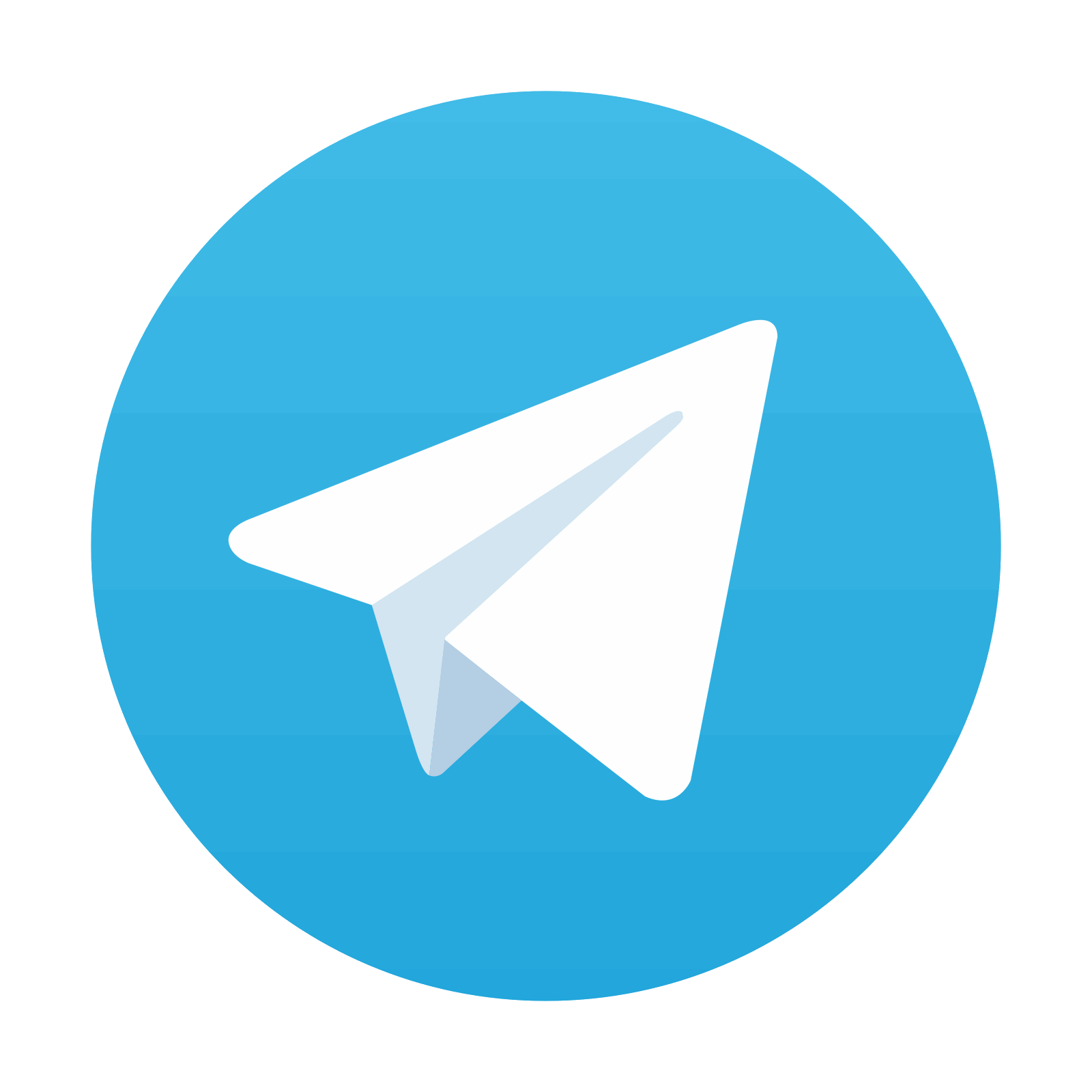
Interdermal injection of CZnS-dots: a bright field, b as-detected fluorescence, and c color-coded images. Insets dissected (in the circled area) axillary lymph node (LN). Adapted from Yang et al., with permission from American Chemical Society. Copyright 2009
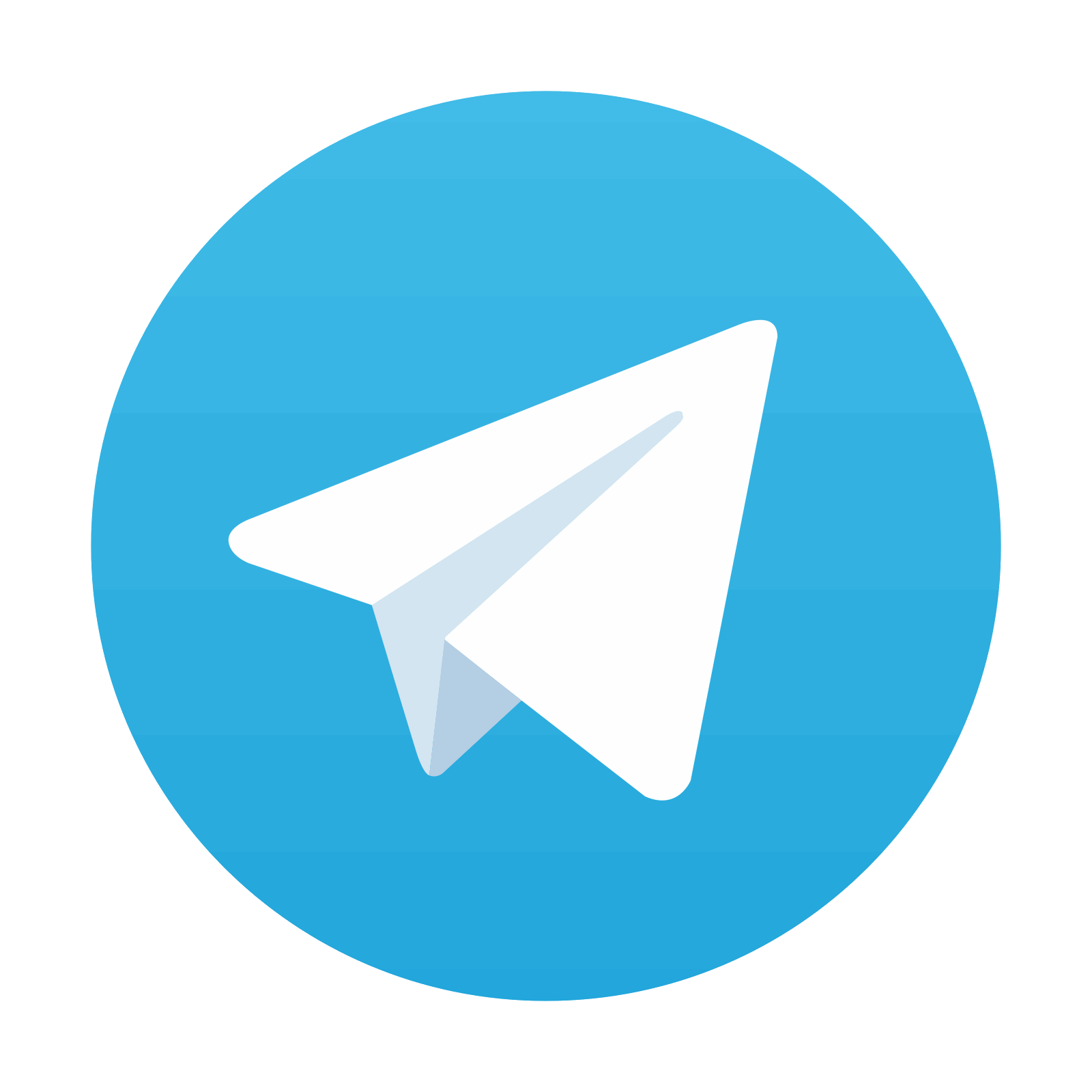
Stay updated, free articles. Join our Telegram channel

Full access? Get Clinical Tree
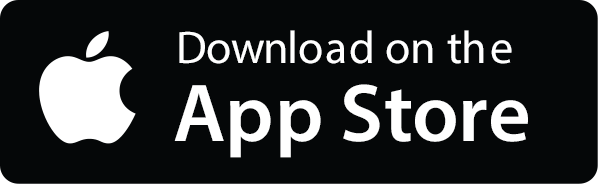
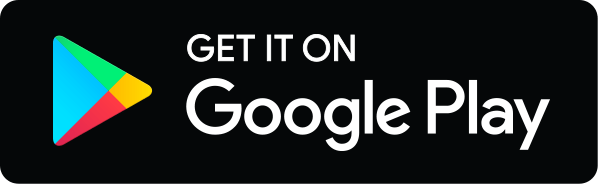
